Abstract
Objective
Vascular endothelial growth factor (VEGF) interaction with its receptor, VEGFR-3/Flt-4, regulates lymphangiogenesis. VEGFR-3/Flt-4 expression in cancer cells has been correlated with clinical stage, lymph node metastasis, and lymphatic invasion. The objective of this study is to identify a VEGFR-3/Flt-4-interacting peptide that could be used to inhibit VEGFR-3 for ovarian cancer therapy.
Methods
The extracellular fragment of recombinant human VEGFR-3/Flt-4 (rhVEGFR-3/Flt-4) fused with coat protein pIII was screened against a phage-displayed random peptide library. Using affinity enrichment and enzyme-linked immunosorbent assay (ELISA) screening, positive clones of phages were amplified. Three phage clones were selected after four rounds of biopanning, and the specific binding of the peptides to rhVEGFR-3 was detected by ELISA and compared with that of VEGF-D. Immunohistochemistry and immunofluorescence analyses of ovarian cancer tissue sections was undertaken to demonstrate the specificity of the peptides.
Results
After four rounds of biopanning, ELISA confirmed the specificity of the enriched bound phage clones for rhVEGFR-3. Sequencing and translation identified three different peptides. Non-competitive ELISA revealed that peptides I, II, and III had binding affinities for VEGFR-3 with Kaff (affinity constant) of 16.4±8.6 µg/mL (n=3), 9.2±2.1 µg/mL (n=3), and 174.8±31.1 µg/mL (n=3), respectively. In ovarian carcinoma tissue sections, peptide III (WHWLPNLRHYAS), which had the greatest binding affinity, also co-localized with VEGFR-3 in endothelial cells lining lymphatic vessels; its labeling of ovarian tumors in vivo was also confirmed.
Epithelial ovarian cancer remains a significant cause of gynecological cancer-related mortality in the Western world [1]. Owing to the paucity of symptoms and its insidious onset, ovarian cancer is often detected at an advanced stage, resulting in poor prognosis with a 5-year survival rate of approximately 30% [2]. Most deaths from ovarian cancer are caused by metastases that are resistant to conventional therapies. Although ovarian cancers metastasize primarily by exfoliation followed by peritoneal implantation, approximately 40% of patients with advanced ovarian cancer show lymph node metastasis and/or extra-abdominal metastasis [3]. Therefore, suppression of tumor angiogenesis and lymphangiogenesis is a major therapeutic approach towards the management of ovarian cancer [4].
Vascular endothelial growth factor receptor 3 (VEGFR-3/Flt-4) interacts with vascular endothelial growth factor C and D (VEGF-C and VEGF-D, respectively) and is extensively expressed in embryonic tissues as well as adult lymphatic endothelia [5]. Its interaction with VEGF-C could promote proliferation of lymphatic endothelia and lymphangiogenesis [6]. In addition, VEGFR-3/Flt-4 expression in cervical cancer cells has been correlated with clinical stage, lymph node metastasis and lymphatic invasion [7]. Furthermore, in esophageal squamous cell carcinoma, patients with VEGF-C- and VEGFR-3-positive tumors had poorer prognosis and greater microlymphatic vessel density, suggesting the importance of this signaling pathway in tumor lymphangiogenesis [8]. Similarly, in ovarian cancer patients, increased levels of circulating CD34+/VEGFR-3+lymphatic/vascular endothelial progenitor cells were associated with lymph node metastasis [9]. Conversely, inhibition of VEGFR-3 suppressed ovarian cancer cell proliferation and downregulated BRCA gene expression [10].
Given the importance of VEGF/VEGFR-3 signaling in lymphangiogenesis, blocking this signaling axis may reduce metastasis. Indeed, targeting VEGF signaling is a well-known treatment option for patients with ovarian cancer [1112]. For example, addition of bevacizumab, a monoclonal antibody specific for VEGF, after carboplatin and paclitaxel chemotherapy increases the median progression-free survival of patients with advanced epithelial ovarian cancer by 4 months [13]. However, serious adverse events, including venous thromboembolism, hypertension, proteinuria, arterial thrombosis, bleeding, and gastrointestinal perforation, have been associated with bevacizumab [1415]. Furthermore, a recent meta-analysis that included 34 trials suggests that the risk of fatal adverse events is increased with bevacizumab [16]. Therefore, additional approaches to blocking this signaling cascade have been developed, including a VEGFR-3 neutralizing antibody [17], a soluble VEGFR-3 [18], monoclonal antibody fragments against VEGF [1920], and inhibitory peptides against VEGF [21].
In the present study, we aimed to identify a VEGFR-3/Flt-4-interacting peptide that could be used to inhibit VEGFR-3 for ovarian cancer therapy. The extracellular fragment of recombinant human VEGFR-3/Flt-4 (rhVEGFR-3/Flt-4) fused with coat protein pIII was screened against a phage-displayed random peptide library. Because peptides can target a small epitope, this approach may overcome some of the limitations of monoclonal antibodies and provide greater targeting specificity [222324] as well as good antigenicity that may make it useful as an anti-tumor vector [25].
The direct coating method of panning was undertaken as previously described [26]. Briefly, 96-well plates were coated with 100 µL of rhVEGFR-3 (5 µg/mL, blocked with bovine serum albumin [BSA]; R&D Systems, Minneapolis, MN, USA) for 1 hour at room temperature. We used the Ph.D.12 phage display peptide library (New England Biolabs, Ipswich, MA, USA) that consists of a combinatorial library of random dodecapeptides fused to a minor coat protein (pIII) of the filamentous M13 bacteriophage with a single-stranded circular DNA genome (6,407 bp) and DNA marker size of 2 Kb. After the phage display peptide library was diluted to approximately 10 plaque forming unit (pfu)/100 µL with TBS-T (Tris-glycine with 0.1% Tween-20, pH 7.4; Sigma-Aldrich, St. Louis, MO, USA), it was incubated with the immobilized rhVEGFR-3 for 7 hours at room temperature. After the wells were washed six times with TBS-T to remove the unbound phages, they were then washed once with glycine buffer, pH 2.2 to elute the bound phages. The titer of the eluted phages was calculated and amplified. Three additional rounds of biopanning were carried out as described above except that unbound phages were washed with TBS-0.2% Tween-20, and the coating protein (rhVEGFR-3) was 5 µg/mL in the second round and 2 µg/mL in the third and fourth rounds. After neutralization in the fourth round of biopanning, the eluted phages were directly inoculated on Luria-Bertani (LB) medium and cultured overnight at 37℃.
After a 96-well plate was coated with 5 µg/mL rhVEGFR-3 at 4℃ overnight and blocked with BSA for 2 hours at room temperature, 50 µL of positive phage solutions were added to each well in serial dilution concentrations, including 2×1010, 1×1010, 0.5×10, 0.25×1010, 0.125×1010, and 0.625×1010 pfu/mL, and incubated for 2 hours at room temperature. Once the plate was washed with TBS-T, 200 µL of horseradish peroxidase (HRP)-conjugated anti-M13 antibody (diluted 1:5,000 in blocking buffer; Amersham Biosciences, Piscataway, NJ, USA) was added to each well and incubated at room temperature for 1 hour with agitation. After a continual TBS-T wash, 2,2'-azino-bis (3-ethylbenzothiazoline-6-sulphonic acid) (ABTS) peroxidase substrate (Roche Diagnostics, Mannheim, Germany) was added to each well, and the absorbance was measured at 410 nmol/L using a microplate reader.
Single-stranded DNA was prepared from overnight cultures (1.5 mL) by extraction and purification using a single-strand M13 extraction and purification kit (Huashun Bio. Co., Guangzhou, China) following the manufacturer's instructions. The isolated single-stranded phage DNA was extracted, visualized by agarose gel electrophoresis and sequenced (100 ng) using an ABI PRISM 310 (PE Applied BioSystems, Foster City, CA, USA). The following primer that is complementary to the genomic DNA of the phage and the downstream of the insert was used for the DNA sequencing: 5'-CCCTCATAGTTAGCGTAACG-3'.
A competitive phagemid enzyme-linked immunosorbent assay (ELISA) was essentially performed as described by Beatty et al. [27]. Briefly, selected phages (synthesized, 109) were mixed with BSA in boric acid buffer, pH 10 (10:1). After dialysis with boric acid buffer, pH 8.5, the phages were coated onto 96-well microtiter plates at different concentrations (20, 10, 5, 2.5, 1.25, and 0.625 µg/mL) overnight at 4℃. After three washes with washing buffer consisting of phosphate buffer saline-Tween-20 (PBS-T; 0.01M PBS, pH 7.2 with 0.05% Tween-20, and 0.01% sodium azide), the wells were blocked with BSA in PBS (pH 7.2) and incubated with 100 µL of a VEGFR-3/Fc chimera diluted in carbonate buffer, pH 9.6 at different concentrations (20, 10, 5, and 2.5 µg/mL) overnight at 4℃. After three washes with wash buffer, the wells were incubated with 50 µL/well of HRP-labeled goat anti-human immunoglobulin G Fc antibody (1:5,000 dilution in 0.5% fish gelatin in PBS; Rockland Immunochemicals, Boyertown, PA, USA) at 37℃ for 1 hour. After three washes with PBS, the wells were incubated with ABTS, and the absorbance was determined at 410 nmol/L (A410) using a microplate reader. When the value of A410 is equivalent to 50% of the largest absorbance (A50), the affinity constant (Ka) of the phages can be calculated as follows: Kaff=n-1/2 ×(n[Ab']-[Ab]) and n=[Ag]/[Ag'] in which, [Ag] and [Ag'] are the concentrations of VEGFR-3 in two different coating solutions and [Ab] and [Ab'] are the amount of the phages at A-50 in the plates coated with two different concentrations of VEGFR-3 [12]. Similar experiments were conducted to analyze the interaction of various concentrations of VEGF-D (1,000, 500, 250, 125, 62.5, and 31.25 µg/mL; R&D Systems).
Ovarian adenocarcinoma tissues were collected by surgical resection from four ovarian cancer stage III patients. Informed consent was obtained from all the patients in advance and this study was approved by the Institutional Review Board of the No.117 Center Military Hospital, Hangzhou, China.
Immunohistochemical and immunofluorescence staining was carried out as previously described [26]. Briefly, 3 µm-thick continual tissue sections from a representative block were placed onto aminopropyltriethoxysilane-treated slides (Sigma, Poole, Dorset, UK) and dried overnight at 37℃. Endogenous peroxidase activity was blocked in 3% hydrogen peroxide for 10 minutes followed by epitope retrieval by microwave boiling the sections in 10 mmol/L citrate buffer (pH 6.0) for 12 minutes. Immunostaining was performed with an Envision kit (Dako, Carpinteria, CA, USA) on a Dako automatic stainer. Detection was made with Envision+ (Dako), a biotin-free detection system that consists of a secondary antibody that is covalently linked to peroxidase-coated dextrose polymers. The primary antibody consisted of a rabbit anti-human VEGFR-3/Flt-4 polyclonal antibody (Chemicon International Inc., Temecula, CA, USA) with 3, 5' diaminobenzidine (DAB, Dako) as the chromogen and hematoxylin as the counterstain. Antibody binding was visualized and photographed under a light microscope.
Immunofluorescence was also performed with an Envision kit on the next continual section. Tissues were incubated with fluorescein isothiocyanate (FITC)-labeled peptide III [FITC-(aminohexanoic, AHX)-WHWLPNLRHYAS], which was synthesized by Zhongkeya Guang (Beijing, China), for overnight at 4℃. Negative controls consisted of FITC-labeled negative peptide [FITC-(AHX)-WHNSLKANYTWG], which has shown negative binding in the phage screening procedure. Slides were analyzed by confocal laser scanning fluorescence microscopy (LSM 510 META, Carl Zeiss GmbH, Oberkochen, Germany) using Zeiss LSM ver. 3.2.
Four to six week-old female Balb/c-nu mice were purchased from Vital River Laboratory Animal Technology (Beijing, China). The mice were injected subcutaneously with 2×106 SKOV3 ovarian cancer cells (Culture Collection of the Chinese Academy of Sciences, Shanghai, China). Once the tumor was 1 cm in diameter, the tumor-bearing mice were injected with technetium (99mTc)-labeled peptide prepared as described below.
99mTc-labeled peptide was freshly prepared using standard protocols [28]. Briefly, 50 µL of peptide (2 µg/µL), NHS-MAG3-SHSWHWLPNLRHYAS, was mixed with 100 µL of 0.1 M citratephosphate buffer, 300 µL tartrate, 100 µL SnCl2 and 100 µL 99mTcO4 in saline. The mixture was sealed with nitrogen gas and incubated at 100℃ for 30 minutes. The labeling efficacy was evaluated by thin layer chromatography. Peptide with 95% 99mTc labeling was used. Each mouse in the experimental group (n=6) was injected with 100 µL of peptide (~3,700 kBq 99mTc/100 µg) via the tail vein. Control mice (n=6) received 100 µL of 9mTcO4 (~3,700 kBq) in saline. After 30 minutes, the mice were anesthetized by ketamine, and radioactivity from the tumor cells was measured using Millennium MPR Spectrometer (BC Technical, West Jordan, UT, USA). The images were acquired over a 15-minute duration. The radioactivity acquired from ovarian tumor cells peaked 3 hours after the tail veil injection. This study was approved by the animal care and use committee of Third Military Medical University (Chongqing, China).
To identify peptides that could be used to inhibit VEGFR-3/Flt-4 for ovarian cancer, phage library screening was undertaken. The yields obtained after four biopanning rounds are listed in Table 1. The yields increased with each round, indicating that specific phages were being enriched.
ELISA using immobilized VEGFR-3 and selected phage clones after four rounds of phage enrichments revealed that the selected phages could interact with VEGFR-3 specifically; weak interaction with BSA or polyethylene was observed (Fig. 1A). As shown in Fig. 1B, the binding affinity of all 10 clones for VEGFR-3 was much higher than that of both BSA and blank control (both p<0.001).
The phage DNA was subjected to agarose gel electrophoresis. As shown in Fig. 2, the length of all the clones was the same as the M13 single-stranded DNA marker and, therefore, the absence of contamination. After subsequent sequencing of the phage DNA, three separate peptides were obtained (Table 2).
The interaction of the isolated peptides with rhVEGFR-3 was next analyzed after preparing peptides I to III for a non-competitive ELISA. One representative experimental curve plot from three experiments of the Kaff (affinity constant) of peptides I, II, and III was shown in Fig. 3 as well as the VEGF-D versus VEGFR-3/Fc interaction was shown in Fig. 4. The Kaff (affinity constant) of peptides I, II, and III were16.4±8.6 µg/mL (n=3), 9.2±2.1 µg/mL (n=3), and 174.8±31.1 µg/mL (n=3), respectively. Peptide III (WHWLPNLRHYAS) had the highest affinity for VEGFR-3 and was nearly as strong as the VEGF-D versus VEGFR-3/Fc interaction that had a Kaff of 264.01±56.7 µg/mL (n=3).
Immunohistochemical and immunofluorescence analysis of VEGFR-3 and peptide III in ovarian carcinoma tissue sections was next undertaken. As shown in Fig. 5, strong immunostaining for VEGFR-3 (Fig. 5A, C, E) appeared on the endothelial cells lining lymphatic vessels. In addition, immunofluorescence analysis confirmed that a FITC-tagged peptide III, could interact with VEGFR-3 (Fig. 5B, D).
After an in vivo ovarian tumor model was established, tumor-bearing mice received a tail vein injection of either 99mTc-labeled peptide or 9mTcO4 as a control. As shown in Fig. 6, 99Tc labeled peptide was observed ovarian tumor (Fig. 6A); however, no such labeling was observed in mice injected with the free probe, which accumulated in the liver, kidney, and bladder (Fig. 6B).
Ovarian cancer remains a significant cause of cancer-related mortality due in large part to the failure to detect it at its early stages [12]. Targeting VEGF signaling is a well-known treatment option for patients with ovarian cancer. Because VEGFR-3 expression in cancer cells has been correlated with clinical stage, lymph node metastasis, and lymphatic invasion [7], we identified three VEGFR-3/Flt-1-interacting peptides. Non-competitive ELISA revealed that peptides I, II, and III had binding affinities for VEGFR-3 of 16.4±8.6, 9.2±2.1, and 174.8±31.1 µg/mL, respectively. Peptide III (WHWLPNLRHYAS) colocalized with VEGFR-3 in endothelial cells lining lymphatic vessels in ovarian carcinoma tissues, suggestive of its binding capabilities.
A large proportion of ovarian cancer-related mortality is due to lymphatic metastasis. VEGFR-3 is an effective tumor marker of lymphatic vessels [29], and roles for VEGF signaling via VEGFR-3 in lymphangiogenesis in cancer patients have been shown [78930]. Therefore, targeting VEGF-VEGFR-3 signaling via an interfering peptide may represent a novel therapeutic approach for ovarian cancer. Peptide-based therapies have several advantages over monoclonal antibodies in that they are smaller, which favors increased tissue penetration. Furthermore, given the specificity of peptides, these targeted ligands may represent carriers for the delivery of genes and/or therapeutic compounds as well as imaging agents [3132]. Using ovarian carcinoma tissues, the co-localization of peptide III with VEGFR-3 in the endothelial cells of lymphatic vessels was confirmed. This result is consistent with another published VEGFR-3-targeted peptide that could specifically bind VEGFR-3-positive carcinoma cells [33]. These results suggest that peptide III may also be a potential carrier for ovarian cancer biotherapy, which will be analyzed in further in vivo studies.
Phage display offers an effective tool for the detection of protein interaction [34]. In the present study, to select the possible carrier peptide of ovarian cancer, a random phage display peptide library constructed on peptide III was screened by biopanning using rhVEGFR-3 as a selective molecule. After three rounds of biopanning, there was a remarkable enrichment in the titer of bound phages after which 10 phage clones were selected from the fourth biopanning that corresponded with three peptides. Further studies will evaluate if any of the three peptides may be a suitable carrier for targeted ovarian cancer biotherapy.
Although the binding between the peptides displayed on the coat protein III and VEGFR-3 was detected, it is important to also analyze the binding properties of the synthesized short linear peptide chain. Non-competitive ELISA is a convenient way to reliably measure the affinity constant [353637], and our results showed that peptide III strongly interacted with VEGFR-3. However, the interaction of peptide III with other VEGF receptors will be assessed in further studies to fully determine its specificity for VEGFR-3.
Although the present study did evaluate the binding of peptide III to VEGFR-3 and compared it with VEGF-D, its antagonism of VEGFR-3 was not demonstrated. Therefore, further studies will evaluate if peptide III inhibits VEGF signaling and compare it to other inhibitors, including monoclonal antibodies. In addition, we did not perform co-immunoprecipitation analysis to evaluate the in vitro interaction of peptide III with VEGFR-3 as we currently do not have antibodies specific for peptide III. Furthermore, the potential function of the peptide, including its effect on angiogenesis as well as endothelial cell proliferation as in Erdag et al. [21], will be assessed to determine the clinical significance of this interaction.
In summary, this study identified VEGFR-3-interacting peptides by screening a phage-displayed random peptide library. The association of peptide III with VEGFR-3 was verified in ovarian cancer tissues by immunofluorescence analysis. Further in vivo studies will explore the capacity of this peptide as a carrier to specifically target VEGFR-3 signaling.
Figures and Tables
Fig. 1
Interaction of phages with recombinant human vascular endothelial growth factor receptor-3 (rhVEGFR-3). (A) The interaction of all 10 phage clones with rhVEGFR-3 was determined by enzyme-linked immunosorbent assay. Negative controls consisted of bovine serum albumin (BSA) as well as uncoated wells. Interaction was determined at an optical density (OD) 410 nmol/L. S and T had no specific meanings; they are just different clone names. (B) The binding affinity of all 10 clones for VEGFR-3 was much higher than that of both BSA and blank control (both p<0.001).
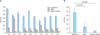
Fig. 2
Agarose gel electrophoresis of single-stranded DNA isolated from the phage clones. Lanes 1 to 10: single-stranded DNA of phage clones; lane M, DNA marker IV.
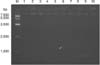
Fig. 3
Interaction of (A) peptides I, (B) II, and (C) III with vascular endothelial growth factor receptor-3 (VEGFR-3). Interaction of peptides I, II, and III with VEGFR-3 was analyzed by non-competitive enzyme-linked immunosorbent assay. A, B, C, and D are the various concentrations of VEGFR-3 analyzed (20, 10, 5, and 2.5 µg/mL, respectively). Interaction was determined at an optical density (OD) 410 nmol/L. One representative experimental curve plot from three experiments of the Kaff (affinity constant) of peptides I, II, and III was shown. The Kaff of peptides I to III was 16.4±8.6, 9.2±2.1, and 174.8±31.1 µg/mL, respectively.
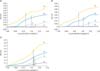
Fig. 4
Interaction of vascular endothelial growth factor (VEGF)-D with vascular endothelial growth factor receptor-3 (VEGFR-3)/Fc. Different concentrations of VEGF-D (1,000, 500, 250, 125, 62.5, and 31.25) were coated on the enzyme-linked immunosorbent assay plates and incubated with VEGFR-3/Fc chimera and immunoglobulin G Fc antibody to generate the binding curves. Interaction was determined at an optical density (OD) 410 nmol/L. One representative experimental curve plot from three experiments of the Kaff (affinity constant) of peptides I, II, and III was shown. The Ka was 264.01±56.7 µg/mL.
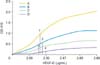
Fig. 5
Immunohistochemical and immunofluorescence staining of ovarian carcinoma tissue sections. Tissue sections were analyzed for vascular endothelial growth factor receptor-3 (A, C, E) and peptide III (B, D) as determined by immunohistochemistry and immunofluorescence staining, respectively. Both were detected on the endothelial cells of lymphatic vessel. (F) The fluorescein isothiocyanate (FITC)-labeled negative peptide [FITC-(aminohexanoic)-WHNSLKANYTWG] was used as negative controls for immunofluorescence (A, B, E, F: ×100; C, D: ×200; A-D: scale bar, 20 µm; E, F: scale bar, 10 µm).
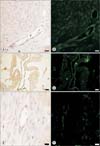
Fig. 6
Localization of peptide III to ovarian tumors in vivo. (A) Technetium-99 (99Tc)-labeled peptide (phage-WHWLPNLRHYAS) interacted with the ovarian tumor in vivo (arrow). (B) Free probe (99Tc) accumulated in the liver, kidney, and bladder, but not at the tumor (arrow).
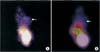
Table 1
Yields of washed phages
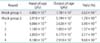
ACKNOWLEDGMENTS
This work was supported by the National Science Foundation of China (30371484). We are very grateful for the comments from Dr. Zhiqing Liang (Department of Obstetrics and Gynecology, Third Military Medical University). We thank Drs. Ling Wang and Guangyuan Liu (Department of Nuclear Medicine, Third Military Medical University) for their technical support and assistance in using the imaging facility.
References
1. Greenlee RT, Murray T, Bolden S, Wingo PA. Cancer statistics, 2000. CA Cancer J Clin. 2000; 50:7–33.
2. Agarwal R, Kaye SB. Ovarian cancer: strategies for overcoming resistance to chemotherapy. Nat Rev Cancer. 2003; 3:502–516.
3. Sant M, Allemani C, Santaquilani M, Knijn A, Marchesi F, Capocaccia R. EUROCARE-4. Survival of cancer patients diagnosed in 1995-1999. Results and commentary. Eur J Cancer. 2009; 45:931–991.
4. Della Pepa C, Banerjee S. Bevacizumab in combination with chemotherapy in platinum-sensitive ovarian cancer. Onco Targets Ther. 2014; 7:1025–1032.
5. Al-Rawi MA, Mansel RE, Jiang WG. Molecular and cellular mechanisms of lymphangiogenesis. Eur J Surg Oncol. 2005; 31:117–121.
6. Ding MX, Li JC, Tang XH. Lymphatic metastasis and VEGF-C expression in ovarian epithelial tumors. Shi Yan Sheng Wu Xue Bao. 2003; 36:445–452.
7. Zhang S, Yu H, Zhang L. Role of vascular endothelial growth factor receptor-3/Flt-4 in early-stage cervical cancer. Oncol Lett. 2010; 1:453–456.
8. Omoto I, Matsumoto M, Okumura H, Uchikado Y, Setoyama T, Kita Y, et al. Expression of vascular endothelial growth factor-C and vascular endothelial growth factor receptor-3 in esophageal squamous cell carcinoma. Oncol Lett. 2014; 7:1027–1032.
9. Qiu H, Cao L, Wang D, Xu H, Liang Z. High levels of circulating CD34+/VEGFR3+ lymphatic/vascular endothelial progenitor cells is correlated with lymph node metastasis in patients with epithelial ovarian cancer. J Obstet Gynaecol Res. 2013; 39:1268–1275.
10. Lim JJ, Yang K, Taylor-Harding B, Wiedemeyer WR, Buckanovich RJ. VEGFR3 inhibition chemosensitizes ovarian cancer stemlike cells through down-regulation of BRCA1 and BRCA2. Neoplasia. 2014; 16:343–353.e2.
11. Underiner TL, Ruggeri B, Gingrich DE. Development of vascular endothelial growth factor receptor (VEGFR) kinase inhibitors as anti-angiogenic agents in cancer therapy. Curr Med Chem. 2004; 11:731–745.
12. He Y, Kozaki K, Karpanen T, Koshikawa K, Yla-Herttuala S, Takahashi T, et al. Suppression of tumor lymphangiogenesis and lymph node metastasis by blocking vascular endothelial growth factor receptor 3 signaling. J Natl Cancer Inst. 2002; 94:819–825.
13. Burger RA, Brady MF, Bookman MA, Fleming GF, Monk BJ, Huang H, et al. Incorporation of bevacizumab in the primary treatment of ovarian cancer. N Engl J Med. 2011; 365:2473–2483.
14. Gordon MS, Cunningham D. Managing patients treated with bevacizumab combination therapy. Oncology. 2005; 69:Suppl 3. 25–33.
15. Nalluri SR, Chu D, Keresztes R, Zhu X, Wu S. Risk of venous thromboembolism with the angiogenesis inhibitor bevacizumab in cancer patients: a meta-analysis. JAMA. 2008; 300:2277–2285.
16. Huang H, Zheng Y, Zhu J, Zhang J, Chen H, Chen X. An updated meta-analysis of fatal adverse events caused by bevacizumab therapy in cancer patients. PLoS One. 2014; 9:e89960.
17. Roberts N, Kloos B, Cassella M, Podgrabinska S, Persaud K, Wu Y, et al. Inhibition of VEGFR-3 activation with the antagonistic antibody more potently suppresses lymph node and distant metastases than inactivation of VEGFR-2. Cancer Res. 2006; 66:2650–2657.
18. Lin J, Lalani AS, Harding TC, Gonzalez M, Wu WW, Luan B, et al. Inhibition of lymphogenous metastasis using adeno-associated virus-mediated gene transfer of a soluble VEGFR-3 decoy receptor. Cancer Res. 2005; 65:6901–6909.
19. Rinderknecht M, Villa A, Ballmer-Hofer K, Neri D, Detmar M. Phage-derived fully human monoclonal antibody fragments to human vascular endothelial growth factor-C block its interaction with VEGF receptor-2 and 3. PLoS One. 2010; 5:e11941.
20. Lamdan H, Gavilondo JV, Munoz Y, Pupo A, Huerta V, Musacchio A, et al. Affinity maturation and fine functional mapping of an antibody fragment against a novel neutralizing epitope on human vascular endothelial growth factor. Mol Biosyst. 2013; 9:2097–2106.
21. Erdag B, Balcioglu KB, Kumbasar A, Celikbicak O, Zeder-Lutz G, Altschuh D, et al. Novel short peptides isolated from phage display library inhibit vascular endothelial growth factor activity. Mol Biotechnol. 2007; 35:51–63.
22. Bird RE, Hardman KD, Jacobson JW, Johnson S, Kaufman BM, Lee SM, et al. Single-chain antigen-binding proteins. Science. 1988; 242:423–426.
23. Yokota T, Milenic DE, Whitlow M, Schlom J. Rapid tumor penetration of a single-chain Fv and comparison with other immunoglobulin forms. Cancer Res. 1992; 52:3402–3408.
24. Mayer A, Chester KA, Flynn AA, Begent RH. Taking engineered anti-CEA antibodies to the clinic. J Immunol Methods. 1999; 231:261–273.
25. Akabani G, McLendon RE, Bigner DD, Zalutsky MR. Vascular targeted endoradiotherapy of tumors using alpha-particle-emitting compounds: theoretical analysis. Int J Radiat Oncol Biol Phys. 2002; 54:1259–1275.
26. Sambrook J, Russell DW. Molecular cloning: a laboratory manual. 3rd ed. Cold Spring Harbor: Cold Spring Harbor Laboratory Press;2001.
27. Beatty JD, Beatty BG, Vlahos WG. Measurement of monoclonal antibody affinity by non-competitive enzyme immunoassay. J Immunol Methods. 1987; 100:173–179.
28. Winnard P Jr, Chang F, Rusckowski M, Mardirossian G, Hnatowich DJ. Preparation and use of NHS-MAG3 for technetium-99m labeling of DNA. Nucl Med Biol. 1997; 24:425–432.
29. Kato S, Shimoda H, Ji RC, Miura M. Lymphangiogenesis and expression of specific molecules as lymphatic endothelial cell markers. Anat Sci Int. 2006; 81:71–83.
30. Ohta Y, Shridhar V, Bright RK, Kalemkerian GP, Du W, Carbone M, et al. VEGF and VEGF type C play an important role in angiogenesis and lymphangiogenesis in human malignant mesothelioma tumours. Br J Cancer. 1999; 81:54–61.
31. Trepel M, Arap W, Pasqualini R. In vivo phage display and vascular heterogeneity: implications for targeted medicine. Curr Opin Chem Biol. 2002; 6:399–404.
32. Sergeeva A, Kolonin MG, Molldrem JJ, Pasqualini R, Arap W. Display technologies: application for the discovery of drug and gene delivery agents. Adv Drug Deliv Rev. 2006; 58:1622–1654.
33. Qin X, Wan Y, Li M, Xue X, Wu S, Zhang C, et al. Identification of a novel peptide ligand of human vascular endothelia growth factor receptor 3 for targeted tumour diagnosis and therapy. J Biochem. 2007; 142:79–85.
34. Smith GP, Petrenko VA. Phage display. Chem Rev. 1997; 97:391–410.
35. Capiaumont J, Jacob C, Sarem M, Nabet P, Belleville F, Dousset B. Assay of a seric human hexapeptide (HWESAS) using a monoclonal antibody and ELISA. Clin Chim Acta. 2000; 293:89–103.
36. Barkia A, Martin C, Puchois P, Gesquiere JC, Cachera C, Tartar A, et al. Enzyme-linked immunosorbent assay for human proapolipoprotein A-I using specific antibodies against synthetic peptide. J Lipid Res. 1988; 29:77–84.
37. Pesce AJ, Michael JG. Artifacts and limitations of enzyme immunoassay. J Immunol Methods. 1992; 150:111–119.