Abstract
Objective
To investigate whether granulocyte-colony stimulating factor (G-CSF) can decrease the extent of ovarian follicle loss caused by cisplatin treatment.
Methods
Twenty-one adult female Sprague-Dawley rats were used. Fourteen rats were administered 2 mg/kg/day cisplatin by intraperitoneal injection twice per week for five weeks (total of 20 mg/kg). Half of the rats (n=7) were treated with 1 mL/kg/day physiological saline, and the other half (n=7) were treated with 100 µg/kg/day G-CSF. The remaining rats (n=7, control group) received no therapy. The animals were then euthanized, and both ovaries were obtained from all animals, fixed in 10% formalin, and stored at 4℃ for paraffin sectioning. Blood samples were collected by cardiac puncture and stored at -30℃ for hormone assays.
Cancer-related mortality in women has significantly decreased during the last decade due to advancements in early diagnostic tests, effective medical treatments, and surgical techniques as well as increased public awareness attributed to successful press and media communications regarding primary care and preventive strategies [1]. Unfortunately, these successful treatment strategies give rise to other challenging problems, especially in young female patients receiving chemotherapy, as most chemotherapeutic agents decrease the number of primordial and larger growing follicles, leading to premature menopause and infertility [2].
Cisplatin, a platinum-based anticancer agent, is widely used for a variety of cancers such as sarcoma, small cell lung cancer, ovarian cancer, cervical cancer, and lymphomas. The mechanism of its antitumor activity relies on the formation of a platinum complex inside of a cell, leading to DNA cross-linking, the arrest of cells in the G2 phase, and apoptosis [3]. Moreover, cisplatin is thought to induce the peroxidation of cell membranes [4], mitochondrial dysfunction [5], and inhibition of protein synthesis [6]. These effects on dividing cancer cells also affect healthy dividing cells in the body, such as the granulosa cells of the follicles, causing acute and chronic ovarian failure resulting in infertility.
Therapeutic options for preventing ovarian damage in female patients desiring future fertility have been previously studied; however, currently available methods remain limited and include fertility-sparing surgery in gynecologic cancers and hormonal protection with gonadotropin releasing hormone analogues and antagonists [7]. Recently, stem cell studies have begun to emerge in reproductive biology. The generation of male and female gametes from mouse embryonic stem cells [8,9] and the development of oocyte-like cells from porcine skin and rat pancreatic stem cells [10,11] have been described. Studies by Lee at al. [12] and Johnson et al. [13] demonstrating follicle protection and long-term fertility preservation in female mice treated with high-dose alkylating chemotherapy followed by bone marrow transplantation have inspired researchers to hypothesize that the mobilization of endogenous bone marrow stem cells by induction with granulocyte-colony stimulating factor (G-CSF) might provide a similar protection of ovarian follicles and fertility.
G-CSF is a glycoprotein with growth factor and cytokine functions [14]. G-CSF has been successfully used in congenital neutropenia and in chemotherapy-induced neutropenia without a decrease in the efficacy of chemotherapeutic agents. With long-term use, its efficacy and safety have been proven. Although toxic and adverse events have been reported, these are not clinically troublesome and seldom necessitate stopping therapy [15]. Recently, the capacity of hematopoietic stem cells to migrate to other organs in response to acute damage to participate in tissue repair has been established in various tissues including the intestine [16], heart [17], liver [18], and kidneys [19]. In addition to its stimulating effects on bone marrow cells for transdifferentiation, G-CSF also has antiapoptotic [20] and anti-inflammatory functions [21].
The potential of G-CSF to protect against cisplatin-induced ovarian failure has not been investigated to date. Thus, here we examined whether G-CSF can reduce the ovarian injury caused by cisplatin in an experimental rat model.
Twenty-one adult female Sprague-Dawley rats weighing 200 to 210 g were used in this study. Animals were housed in cages and maintained under standard conditions with a 12-hour light/dark cycle at room temperature (22℃±2℃). The rats were fed a standard pellet diet and were provided tap water ad libitum throughout the study. The protocol used in this study was approved by the Institutional Animal Care and Ethical Committee of Ege University.
For 14 rats, cisplatin (Sisplatin Flakon, Koçak, Farma, Turkey; 2 mg/kg/day) was administered by intraperitoneal injection twice per week for 5 weeks (total 20 mg/kg) [22]. No drug was administered to the remaining rats, which were considered a control group (n=7). The 14 rats receiving cisplatin were randomly divided into two groups: the first cisplatin group was treated with 1 mL/kg/day physiological saline (PS) (CP+PS; n=7), and the second cisplatin group was treated with 100 µg/kg/day G-CSF (Neupogen, Roche, Mannheim, Germany; 48 MU/mL=480 µg) [23,24]. Injections were administered intraperitoneal every day for 5 weeks. None of the rats died in the study.
All rats were euthanized at the end of the treatment period. Both ovaries from all animals were recovered, fixed in 10% formalin, and stored at 4℃ for paraffin sectioning. Blood samples were collected by cardiac puncture and stored at -30℃ for hormone assays.
Formalin-preserved ovarian tissues were embedded in paraffin. The ovaries were sectioned at a thickness of 4 µm using a microtome, and the sections were mounted onto glass slides and stained with hematoxylin and eosin. An Olympus C-5050 digital camera (Olympus, Tokyo, Japan) mounted on an Olympus BX51 microscope was used to photograph all sections. Morphological analysis of the ovaries was performed using a computerized image analysis system (Image-ProExpress 1.4.5, Media Cybernetics Inc., Rockville, MD, USA) on 10 fields of view per section at a magnification of 20×; the observer was blinded to the study groups. Ovarian follicles at different developmental stages were categorized according to standards established by Oktay et al. [25] (Fig. 1). Primordial follicles are localized immediately below the cortex and contain a single layer of squamous granulosa cells. Primary follicles consist of a single layer of cuboidal granulosa cells. Secondary follicles include multiple layers of granulosa cells, and tertiary follicles are characterized by a stratum granulosum and a fluid filled antral space.
Plasma anti-Müllerian hormone (AMH) levels were measured using a commercially available enzyme-linked immunosorbent assay kit (USCN Life Science Inc., Hubei, China). Samples from each animal were measured in duplicate according to the manufacturer's instructions. The detection range was 0.156 to 10 ng/mL.
Data analyses were performed using SPSS ver. 15.0 (SPSS Inc., Chicago, IL, USA). The groups of parametric variables were evaluated by an analysis of variance (ANOVA) followed by the post hoc Tukey honest significant difference test. The results are presented as the mean±standard error. A value of p<0.05 was considered to be statistically significant.
Histological examination of the ovaries demonstrated significant alterations in cisplatin+PS group including decreased follicle numbers and follicular maturation whereas control group exhibited normal ovarian morphology. However, treatment of the rats with G-CSF significantly lessened the follicular impairment and enhanced the immature follicles (Fig. 1).
The follicle counts of the groups are summarized in Table 1. ANOVA results showed significant differences between the study groups (p=0.001 for primordial follicles, p=0.01 for primary follicles, p<0.001 for secondary follicles, and p=0.005 for tertiary follicles). The number of primordial follicles showed a significant reduction in the cisplatin+PS group compared to the control group (2.8±0.4 vs. 18.2±0.8, respectively; p<0.001). Treatment of rats with G-CSF considerably improved the number of primordial follicles (14.5±1.4, p<0.001). Similarly, primary and secondary follicle numbers of the cisplatin+PS group were significantly different compared to control group (8.3±0.5 vs. 14.6±1.1 and 6.7±0.5 vs. 10.8±0.9; p<0.001). Treatment of rats with G-CSF effectively prevented the cisplatin-induced damage in primary and secondary follicles (15.1±2.1 and 10.3±1.3; p=0.02 and p=0.004, respectively). Tertiary follicle counts were 4.7±0.2 in the control group, 1.2±0.3 in the cisplatin+PS group, and 3.5±0.7 in the cisplatin+G-CSF group. The differences between the groups were also statistically significant (p<0.001 and p=0.01, respectively).
Plasma AMH levels were measured to determine the effects of G-CSF on follicle pool of cisplatin-treated rats. Table 2 shows the alterations in AMH levels of the study groups. Compared to the control group, plasma AMH levels were significantly decreased in cisplatin+PS group (2.38±0.17 ng/mL vs. 0.41±0.02 ng/mL, respectively; p<0.001). G-CSF treatment significantly improved the AMH levels compared to cisplatin+PS group (1.56±0.247 ng/mL, p=0.006) (Table 2).
We examined the effects of G-CSF on ovarian reserves by measuring follicle counts and serum AMH levels after cisplatin therapy. Treatment without G-CSF caused a significant decrease in the follicle number and serum AMH levels, whereas these measures were significantly increased when G-CSF was added to the treatment.
Although the specific protective mechanisms of G-CSF remain unclear, we present several hypotheses. One possible explanation is that G-CSF has anti-apoptotic properties that occur through the Jak/Stat, PI3K/AKT, and MAPK/AKT pathways [26,27]. AKT is an important antiapoptotic protein that balances cell survival and cell death through the phosphorylation of Bad, which is a proapoptotic member of the Bcl-2 family. In its unphosphorylated form, Bad binds to Bcl-XL (an antiapoptotic protein) and thus blocks cell survival. However, the activation of AKT leads to Bad phosphorylation and inhibits its proapoptotic activity [28]. G-CSF also induces the expression of Bcl-2 and decreases Bax translocation to the mitochondria, leading to apoptosis inhibition and cell survival [20]. Another important antiapoptotic mechanism of G-CSF acts through the JAK/Stat signaling pathway and leads to subsequent activation of Bcl-2, which protects against cell death [29]. Although further work is needed to better understand the molecular mechanisms, it is likely that G-CSF protects against apoptosis.
A second possible explanation of the protective mechanism of G-CSF is that G-CSF has anti-inflammatory properties. In several studies, a decreased production of pro-inflammatory cytokines, such as interleukin 6 (IL-6), tumor necrosis factor-α (TNF-α), nuclear factor-κB, and IL-1β, upon G-CSF administration has been demonstrated [20,26,30], leading to reduced inflammation and an amelioration of a potentially destructive inflammatory response. Accumulating evidence also indicates that elevated plasma levels of IL-6 and TNF-α are commonly observed in animals treated with cisplatin, which might contribute to germ cell apoptosis [31]. Thus, G-CSF might contribute to the survival of follicles by suppressing the production of proinflammatory cytokines.
A third potential protective mechanism is that G-CSF plays a role in neoangiogenesis. In a study by Lee et al. [32], a 3-day treatment with G-CSF (50 µg/kg/day) after occlusion of the middle cerebral artery enhanced angiogenesis in the rat brain. An up-regulation of intracellular adhesion molecule 1 by G-CSF was also reported to facilitate the migration of monocytes that engage in angiogenesis [33]. In an acute myocardial infarction experimental rat model, Kocher et al. [34] injected G-CSF intravenously and observed significantly increased neovascularization at the site of the myocardial infarction. In a recent study by Skaznik-Wikiel et al. [35], high-dose cyclophosphamide and busulfan chemotherapy caused a significant reduction in ovarian microvessel density compared to vehicle-treated controls. In contrast, the microvessel density in mice treated with chemotherapeutic agents and G-CSF was similar to that observed in vehicle treated control mice.
G-CSF is known to mobilize hematopoietic stem cells from bone marrow into peripheral blood. These undifferentiated cells are thought to commit to a differentiation program, integrate into tissue, and replace damaged cells to reconstruct a damaged cell mass. This transdifferentiation has been reported in many studies [36]. However, it was recently reported that cell fusion, rather than transdifferentiation, is the major mechanism underlying organ repair [37]. Thus, it remains controversial whether fusion or the transdifferentiation of bone marrow cells into other tissues aids organ repair. In a study by Yannaki et al. [38], G-CSF administration ameliorated histological damage and accelerated the regeneration process in both an acute and chronic liver injury model. However, the acceleration of recovery after chemical injury and G-CSF treatment was mainly mediated by an increased proliferation of host hepatocytes with less support from cells of bone marrow origin. Another study demonstrated that in ex vivo perfused rat hearts subjected to ischemia-reperfusion injury, the injury was ameliorated by G-CSF without any bone marrow effect [29]. Based on these findings, we propose that G-CSF may have non-hematopoietic effects and function as a growth factor for tissue repair and regeneration.
Another explanation for the protective effects of G-CSF is that G-CSF as a growth factor to initiate or trigger endogenous repair mechanisms. In a study by Hess et al. [39], bone marrow-derived cells were found to reverse hyperglycemia in streptozotocin-treated mice by inducing endogenous pancreatic tissue repair through possible interactions with endothelial cells. In addition a study by Ledee et al. [40] reported that follicular fluid G-CSF influenced future embryo implantation, suggesting that G-CSF plays an autocrine/paracrine role in the reproductive system.
A final hypothesis is that the protective mechanism of G-CSF is via the beneficial effects of G-CSF in neutralizing the effects of reactive oxygen species (ROS), which are strongly induced in tissues after chemotherapy treatment [41]. In many studies, G-CSF was found to induce the synthesis of endogenous nitric oxide, which is a powerful scavenger of excessive ROS, by either activating the Akt/eNOS pathway [42] or by shifting the balance between the pro- and anti-inflammatory cytokines towards a more favorable anti-inflammatory net effect [20,26,30].
Despite the interesting findings, our study had certain limitations. The number of subjects included in the subgroups was small; hence, a larger sample size is necessary to enhance the power of our study. Moreover, we did not examine the exact molecular mechanisms by which G-CSF ameliorates follicle loss after cisplatin treatment. Detailed studies are necessary to evaluate the optimal timing and dosage of G-CSF relative to chemotherapy.
In summary, our results showed that G-CSF is beneficial in decreasing follicle loss in an experimental rat model of cisplatin chemotherapy. One of the main inferences of our study is that harmless effects of the cisplatin for patients desiring further fertility may be diminished with G-CSF. It is hoped that our findings will inspire others to undertake more detailed researches focused on the underlying molecular mechanisms of the observed effect of G-CSF, ultimately leading to protection of the patients from ovarian damage caused by cisplatin chemotherapy.
Figures and Tables
Fig. 1
Histological evaluation of ovaries in control (A), cisplatin (CP)+physiological saline (PS) (B), and cisplatin+granulocyte-colony stimulating factor (G-CSF) group (C). Cisplatin+PS group showed stromal and follicular damage. G-CSF treatment significantly reduced cisplatin-induced changes in ovaries (H&E, ×20). CL, corpus luteum; pr, primary follicle; sf, secondary follicle; tf, tertiary follicle; v, vessel.
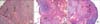
ACKNOWLEDGMENTS
This study received the scientific research award in 18th World Congress on Controversies in Obstetrics, Gynecology & Infertility (COGI), 24 to 27 October, 2013, Vienna, Austria.
References
1. Siegel R, Naishadham D, Jemal A. Cancer statistics, 2012. CA Cancer J Clin. 2012; 62:10–29.
2. Anderson RA, Themmen AP, Al-Qahtani A, Groome NP, Cameron DA. The effects of chemotherapy and long-term gonadotrophin suppression on the ovarian reserve in premenopausal women with breast cancer. Hum Reprod. 2006; 21:2583–2592.
3. Meraner V, Gamper EM, Grahmann A, Giesinger JM, Wiesbauer P, Sztankay M, et al. Monitoring physical and psychosocial symptom trajectories in ovarian cancer patients receiving chemotherapy. BMC Cancer. 2012; 12:77.
4. Sugiyama S, Hayakawa M, Kato T, Hanaki Y, Shimizu K, Ozawa T. Adverse effects of anti-tumor drug, cisplatin, on rat kidney mitochondria: disturbances in glutathione peroxidase activity. Biochem Biophys Res Commun. 1989; 159:1121–1127.
5. Brady HR, Kone BC, Stromski ME, Zeidel ML, Giebisch G, Gullans SR. Mitochondrial injury: an early event in cisplatin toxicity to renal proximal tubules. Am J Physiol. 1990; 258(5 Pt 2):F1181–F1187.
6. Leibbrandt ME, Wolfgang GH, Metz AL, Ozobia AA, Haskins JR. Critical subcellular targets of cisplatin and related platinum analogs in rat renal proximal tubule cells. Kidney Int. 1995; 48:761–770.
7. Li X, Kang X, Deng Q, Cai J, Wang Z. Combination of a GnRH agonist with an antagonist prevents flare-up effects and protects primordial ovarian follicles in the rat ovary from cisplatin-induced toxicity: a controlled experimental animal study. Reprod Biol Endocrinol. 2013; 11:16.
8. Hubner K, Fuhrmann G, Christenson LK, Kehler J, Reinbold R, De La Fuente R, et al. Derivation of oocytes from mouse embryonic stem cells. Science. 2003; 300:1251–1256.
9. Geijsen N, Horoschak M, Kim K, Gribnau J, Eggan K, Daley GQ. Derivation of embryonic germ cells and male gametes from embryonic stem cells. Nature. 2004; 427:148–154.
10. Dyce PW, Wen L, Li J. In vitro germline potential of stem cells derived from fetal porcine skin. Nat Cell Biol. 2006; 8:384–390.
11. Danner S, Kajahn J, Geismann C, Klink E, Kruse C. Derivation of oocyte-like cells from a clonal pancreatic stem cell line. Mol Hum Reprod. 2007; 13:11–20.
12. Lee HJ, Selesniemi K, Niikura Y, Niikura T, Klein R, Dombkowski DM, et al. Bone marrow transplantation generates immature oocytes and rescues long-term fertility in a preclinical mouse model of chemotherapy-induced premature ovarian failure. J Clin Oncol. 2007; 25:3198–3204.
13. Johnson J, Bagley J, Skaznik-Wikiel M, Lee HJ, Adams GB, Niikura Y, et al. Oocyte generation in adult mammalian ovaries by putative germ cells in bone marrow and peripheral blood. Cell. 2005; 122:303–315.
14. Weisbart RH, Gasson JC, Golde DW. Colony-stimulating factors and host defense. Ann Intern Med. 1989; 110:297–303.
15. Martino M, Fedele R, Massara E, Recchia AG, Irrera G, Morabito F. Long-term safety of granulocyte colony-stimulating factor in normal donors: is it all clear? Expert Opin Biol Ther. 2012; 12:609–621.
16. Kudo T, Matsumoto T, Nakamichi I, Yada S, Esaki M, Jo Y, et al. Recombinant human granulocyte colony-stimulating factor reduces colonic epithelial cell apoptosis and ameliorates murine dextran sulfate sodium-induced colitis. Scand J Gastroenterol. 2008; 43:689–697.
17. Orlic D, Kajstura J, Chimenti S, Limana F, Jakoniuk I, Quaini F, et al. Mobilized bone marrow cells repair the infarcted heart, improving function and survival. Proc Natl Acad Sci U S A. 2001; 98:10344–10349.
18. Spahr L, Lambert JF, Rubbia-Brandt L, Chalandon Y, Frossard JL, Giostra E, et al. Granulocyte-colony stimulating factor induces proliferation of hepatic progenitors in alcoholic steatohepatitis: a randomized trial. Hepatology. 2008; 48:221–229.
19. Iwasaki M, Adachi Y, Minamino K, Suzuki Y, Zhang Y, Okigaki M, et al. Mobilization of bone marrow cells by G-CSF rescues mice from cisplatin-induced renal failure, and M-CSF enhances the effects of G-CSF. J Am Soc Nephrol. 2005; 16:658–666.
20. Solaroglu I, Tsubokawa T, Cahill J, Zhang JH. Anti-apoptotic effect of granulocyte-colony stimulating factor after focal cerebral ischemia in the rat. Neuroscience. 2006; 143:965–974.
21. Sehara Y, Hayashi T, Deguchi K, Zhang H, Tsuchiya A, Yamashita T, et al. Decreased focal inflammatory response by G-CSF may improve stroke outcome after transient middle cerebral artery occlusion in rats. J Neurosci Res. 2007; 85:2167–2174.
22. Authier N, Gillet JP, Fialip J, Eschalier A, Coudore F. An animal model of nociceptive peripheral neuropathy following repeated cisplatin injections. Exp Neurol. 2003; 182:12–20.
23. Sugiyama Y, Yagita Y, Oyama N, Terasaki Y, Omura-Matsuoka E, Sasaki T, et al. Granulocyte colony-stimulating factor enhances arteriogenesis and ameliorates cerebral damage in a mouse model of ischemic stroke. Stroke. 2011; 42:770–775.
24. Shima C, Adachi Y, Minamino K, Okigaki M, Shi M, Imai Y, et al. Neuroprotective effects of granulocyte colony-stimulating factor on ischemia-reperfusion injury of the retina. Ophthalmic Res. 2012; 48:199–207.
25. Oktay K, Schenken RS, Nelson JF. Proliferating cell nuclear antigen marks the initiation of follicular growth in the rat. Biol Reprod. 1995; 53:295–301.
26. Schneider A, Kruger C, Steigleder T, Weber D, Pitzer C, Laage R, et al. The hematopoietic factor G-CSF is a neuronal ligand that counteracts programmed cell death and drives neurogenesis. J Clin Invest. 2005; 115:2083–2098.
27. Oishi A, Otani A, Sasahara M, Kojima H, Nakamura H, Yodoi Y, et al. Granulocyte colony-stimulating factor protects retinal photoreceptor cells against light-induced damage. Invest Ophthalmol Vis Sci. 2008; 49:5629–5635.
28. Pastuszko P, Schears GJ, Pirzadeh A, Kubin J, Greeley WJ, Wilson DF, et al. Effect of granulocyte-colony stimulating factor on expression of selected proteins involved in regulation of apoptosis in the brain of newborn piglets after cardiopulmonary bypass and deep hypothermic circulatory arrest. J Thorac Cardiovasc Surg. 2012; 143:1436–1442.
29. Harada M, Qin Y, Takano H, Minamino T, Zou Y, Toko H, et al. G-CSF prevents cardiac remodeling after myocardial infarction by activating the Jak-Stat pathway in cardiomyocytes. Nat Med. 2005; 11:305–311.
30. Xu Y, Hunt NH, Bao S. The role of granulocyte macrophage-colony-stimulating factor in acute intestinal inflammation. Cell Res. 2008; 18:1220–1229.
31. Miller RP, Tadagavadi RK, Ramesh G, Reeves WB. Mechanisms of Cisplatin nephrotoxicity. Toxins (Basel). 2010; 2:2490–2518.
32. Lee ST, Chu K, Jung KH, Ko SY, Kim EH, Sinn DI, et al. Granulocyte colony-stimulating factor enhances angiogenesis after focal cerebral ischemia. Brain Res. 2005; 1058:120–128.
33. Deindl E, Zaruba MM, Brunner S, Huber B, Mehl U, Assmann G, et al. G-CSF administration after myocardial infarction in mice attenuates late ischemic cardiomyopathy by enhanced arteriogenesis. FASEB J. 2006; 20:956–958.
34. Kocher AA, Schuster MD, Szabolcs MJ, Takuma S, Burkhoff D, Wang J, et al. Neovascularization of ischemic myocardium by human bone-marrow-derived angioblasts prevents cardiomyocyte apoptosis, reduces remodeling and improves cardiac function. Nat Med. 2001; 7:430–436.
35. Skaznik-Wikiel ME, McGuire MM, Sukhwani M, Donohue J, Chu T, Krivak TC, et al. Granulocyte colony-stimulating factor with or without stem cell factor extends time to premature ovarian insufficiency in female mice treated with alkylating chemotherapy. Fertil Steril. 2013; 99:2045–2054.e3.
36. Al-Awqati Q, Oliver JA. Stem cells in the kidney. Kidney Int. 2002; 61:387–395.
37. Wang X, Willenbring H, Akkari Y, Torimaru Y, Foster M, Al-Dhalimy M, et al. Cell fusion is the principal source of bone-marrow-derived hepatocytes. Nature. 2003; 422:897–901.
38. Yannaki E, Athanasiou E, Xagorari A, Constantinou V, Batsis I, Kaloyannidis P, et al. G-CSF-primed hematopoietic stem cells or G-CSF per se accelerate recovery and improve survival after liver injury, predominantly by promoting endogenous repair programs. Exp Hematol. 2005; 33:108–119.
39. Hess D, Li L, Martin M, Sakano S, Hill D, Strutt B, et al. Bone marrow-derived stem cells initiate pancreatic regeneration. Nat Biotechnol. 2003; 21:763–770.
40. Ledee N, Lombroso R, Lombardelli L, Selva J, Dubanchet S, Chaouat G, et al. Cytokines and chemokines in follicular fluids and potential of the corresponding embryo: the role of granulocyte colony-stimulating factor. Hum Reprod. 2008; 23:2001–2009.
41. Masuda H, Tanaka T, Tateishi M, Naito M, Tamai H. Detection and cytotoxicity of cisplatin-induced superoxide anion in monolayer cultures of a human ovarian cancer cell line. Cancer Chemother Pharmacol. 2001; 47:155–160.
42. Park KW, Kwon YW, Cho HJ, Shin JI, Kim YJ, Lee SE, et al. G-CSF exerts dual effects on endothelial cells: opposing actions of direct eNOS induction versus indirect CRP elevation. J Mol Cell Cardiol. 2008; 45:670–678.