Abstract
Objective
The purpose of this study was to investigate whether selective cyclooxygenase (COX) inhibitors promote paclitaxel-induced apoptosis in taxane-resistant ovarian cancer cells by suppressing MDR1/P-glycoprotein (P-gp) expression.
Methods
Taxane-resistant ovarian cancer cells were cultured with paclitaxel alone or combined with a selective COX inhibitors. The expression patterns of MDR1/P-gp and the ability of COX inhibitors to inhibit growth of taxane-resistant ovarian cancer cells were measured. The efficacy of prostaglandin E2 (PGE2) supplementation was measured to evaluate the mechanisms involved in suppressing MDR1 gene expression.
Results
P-gp was upregulated in taxane-resistant ovarian cancer cells compared to paired paclitaxel-sensitive ovarian cancer cells. An 3-(4,5-dimethylthiazol-2-yl)-2,5-diphenyltetrazolium bromide (MTT) assay showed that selective COX inhibitors significantly enhanced the cytotoxic effects of paclitaxel in taxane-resistant ovarian cancer cells via a prostaglandin-independent mechanism. These increased apoptotic effects were further verified by measuring an increased percentage of cells in sub-G1 stage using flow cytometry. Selective COX inhibitors suppressed MDR1 and P-gp expression. Moreover, combined treatment with paclitaxel and selective COX inhibitors increased poly (ADP-ribose) polymerase (PARP) cleavage in taxane-resistant ovarian cancer cells.
Conclusion
Selective COX inhibitors significantly promote paclitaxel-induced cell death in taxane-resistant ovarian cancer cells in a prostaglandin-independent manner. COX inhibitors could be potent therapeutic tools to promote paclitaxel sensitization of taxane-resistant ovarian cancers by suppressing MDR1/P-gp, which is responsible for the efflux of chemotherapeutic agents.
Cytoreductive surgery followed by intermittent chemotherapy with taxane and a platinum chemotherapeutic agent is the standard treatment for ovarian cancer, and has an initial response rate of up to 80% [1,2]. The majority of patients, however, relapses after a median period of 18 months, and multi-drug resistance (MDR) to chemotherapeutic agents impedes successful chemotherapy of recurrent ovarian cancers [3]. This MDR phenomenon allows cancer cells to survive in the presence of structurally and functionally different chemotherapeutic agents, and is the main cause of therapeutic failure in ovarian cancers [4].
The most important mechanism responsible for the MDR phenotype is the overexpression of drug efflux transporter genes in cancer cells, such as P-glycoprotein (P-gp), an ATP-binding cassette (ABC) transporter [5,6]. P-gp transporter, encoded by the MDR1 gene, acts as a cell membrane pump extruding drugs to the extracellular space, thereby reducing drug accumulation in cells. As reported previously, various toxins and chemotherapeutic drugs, including taxanes and anthracyclines are MDR1 substrates [7].
Cyclooxygenase-2 (COX-2) is a key enzyme in converting arachidonic acid to prostaglandins, and it has been accepted as an unfavorable prognostic factor in various tumors, including ovarian cancer [8]. To overcome drug resistance by COX-2, COX-2 inhibitors have been studied in various cancers, such as leukemia, colon cancer, and hepatocellular carcinoma [9-12]. Selective COX-2 inhibitors have been recognized for their anticancer effects, in addition to their effects in treating rheumatoid arthritis and managing pain [13]. Moreover, selective COX-2 inhibitors sensitize MDR cancer cells to chemotherapeutic drugs in either COX-2-dependent or COX-2-independent mechanisms [14].
The aim of the present study was to demonstrate the usefulness of selective COX inhibitors in promoting paclitaxel-induced apoptosis in taxane-resistant ovarian cancer cells, and to understand the mechanisms involved in the selective COX inhibitors suppressing MDR1 gene expression.
NS-398 and SC-560, selective COX inhibitors, were purchased from Cayman Chemical Co. (Ann Arbor, MI, USA). Paclitaxel was purchased from the Cheil General Hospital Pharmacy (Seoul, Korea). LY294002, a selective PI3K inhibitor, was purchased from Cell Signaling Technology (Beverly, MA, USA). Prostaglandin E2 (PGE2) was purchased from Sigma-Aldrich (St Louis, MO, USA). Antibodies against COX-1, COX-2, and cleaved poly ADP ribose polymerase (PARP) were supplied by Cell Signaling Technology (Beverly, MA, USA). The anti-MDR1 antibody was obtained from Abcam (Cambridge, UK). The anti-actin antibody was purchased from Santa Cruz Biotechnology (Santa Cruz, CA, USA). The anti-rabbit and anti-mouse horseradish peroxidase-conjugated secondary antibodies were obtained from Cell Signaling Technology.
Human ovarian carcinoma cell lines SKOV3ip1, HeyA8 (taxane-sensitive), SKOV3ip2-TR, HeyA8-MDR (taxane-resistant) were provided by Dr. AK Sood (Texas MD Anderson Cancer Center, TX, USA). SKOV3ip1 and HeyA8 cells were grown in RPMI 1640 supplemented with 10% fetal bovine serum (FBS) and 0.5% gentamicin. SKOV3ip2-TR and HeyA8-MDR cells were grown in RPMI 1640 supplemented with 10% FBS and 0.5% gentamicin, and with 300 ng/mL paclitaxel.
Cells (5×105) were lysed in a lysis buffer (10 mM Tris-HCl [pH 8.0], 150 mM NaCl, 1% NP-40, 1% sodium deoxycholate, 0.1% sodium dodecyl sulphate [SDS]), proteins were resolved by SDS-polyacrylamide gel electrophoresis (PAGE) and transferred onto nitrocellulose membranes (Millipore, Bedford, MA, USA). Membranes were blocked with 5% skim milk in tris-buffered saline with tween 20 (TBS-T) buffer (10 mM Tris-HCl [pH 7.5], 150 mM NaCl, 0.1% Tween-20) for 1 hour and then incubated with relevant antibodies for 18 hours at 4℃. Membranes were washed with TBS-T buffer and incubated for 1 hour at room temperature (RT) with horseradish peroxidase-conjugated anti-rabbit IgG or anti-mouse IgG at a dilution of 1:5,000. Protein bands were visualized using enhanced chemiluminescence (Amersham-Pharmacia, Piscataway, NJ, USA).
Cells (3×103) were seeded in 96-well microplates treated with COX inhibitors, PGE2, or paclitaxel as indicated. At 72 hours after incubation, 100 µL/well of 2 mg/mL 3-(4,5-dimethylthiazol-2-yl)-2,5-diphenyltetrazolium bromide (MTT) solution was added to the microplates. Two hours after MTT treatment, the medium was removed and formazan crystals were dissolved by adding 100 µL dimethylsulfoxide per well. Cell viability was analyzed by measuring the absorbance at 590 nm using an enzyme-linked immunosorbent assay reader.
Total RNA was extracted using TRIzol reagent (Invitrogen, Carlsbad, CA, USA). One microgram of the isolated total RNA was converted to cDNA using M-MLV reverse transcriptase (Promega, Madison, WI, USA). AvPCR primer set specific for each gene was used for amplification. PCR products were resolved on a 2.0% agarose gel and visualized by ethidium bromide staining.
Cells (5×105) were harvested and fixed with 70% (w/v) ice-cold ethanol at 4℃ for 1 hour. Fixed cells were washed twice with phosphate-buffered saline (PBS) and stained with PBS containing 50 µg/mL propidium iodide (PI) and 100 µg/mL Ribonuclease A (RNase A). Following incubation for 15 minutes in the dark at RT, the number of cells in each cell cycle stage was analyzed using FACSCalibur Flow Cytometry (BD Bioscience, Franklin Lakes, NJ, USA) and CellQuest software (BD Bioscience).
To investigate whether taxane resistance is associated with increased expression of MDR1/P-gp, COX-1, or COX-2, we examined their expression in ovarian cancer cell lines (HeyA8-MDR, SKOV3ip2-TR, HeyA8, and SKOV3ip1). As expected, the taxane-resistant ovarian cancer cell lines (HeyA8-MDR and SKOV3ip2-TR) expressed more MDR1/P-gp than the taxane-sensitive ovarian cancer cell lines (HeyA8 and SKOV3ip1) (Fig. 1). COX expression, however, was inconsistent. COX-1 was not expressed in all cell lines, and COX-2 was only expressed in SKOV3ip2-TR, a taxane-resistant cell line (Fig. 1).
To determine the cytotoxic effect of COX inhibitors in paclitaxel-resistant ovarian cancer cells, the viability of cells treated with COX inhibitors was measured using an MTT assay. The results show that selective COX inhibitors enhanced paclitaxel cytotoxiticy in the taxane-resistant ovarian cancer cell lines (HeyA8-MDR and SKOV3ip2-TR) (Fig. 2). Subsequently, paclitaxel-resistant ovarian cancer cells were treated with paclitaxel (100 nM) combined with a selective inhibitor for COX-1 (SC560) or COX-2 (NS398) at various concentrations. SC560 and NS398 significantly enhanced the cytotoxic effects of paclitaxel at 20 and 100 µM, respectively, in SKOV3ip2-TR cells. In HeyA8-MDR cells, SC560 significantly increased the cytotoxic effect of paclitaxel at 20 µM.
To investigate whether paclitaxel and COX inhibitors could enhance apoptosis in taxane-resistant ovarian cancer cells, we analyzed the distribution of HeyA8-MDR and SKOV3ip2-TR cells in various cell cycle stages when treated with paclitaxel (100 nM) alone or combined with SC560 (20 µM) or NS398 (100 µM) (Fig. 3A, B). Combined treatment with paclitaxel and SC560 or NS398 increased the sub-G1 fraction. Furthermore, immunoblot analysis for PARP cleavage revealed that combined treatment with paclitaxel and selective COX inhibitors increased the cytotoxic effect in taxane-resistant ovarian cancer cells (Fig. 3C). These results indicate that selective COX inhibitors increase apoptosis in taxane-resistant ovarian cancer cells treated with paclitaxel.
To determine whether enhanced apoptosis of taxane-resistant ovarian cancer cells treated with paclitaxel and a selective COX inhibitor was mediated by prostaglandins, we added PGE2 (10 µM) to taxane-resistant ovarian cells treated with paclitaxel and COX inhibitors (Fig. 4). Adding PGE2 did not change the effects of COX inhibitors in taxane-resistant ovarian cancer cells. These results indicate that selective COX inhibitors may overcome paclitaxel resistance by ovarian cancer cells in a prostaglandin-independent manner.
To clarify the precise mechanisms by which selective COX inhibitors inhibit the growth of paclitaxel-resistant ovarian cancer cells, MDR gene expression was analyzed in ovarian cancer cells treated with a COX inhibitor. Combined treatment with paclitaxel and COX inhibitors reduced expression of the MDR1 gene (Fig. 5A) and P-gp protein (Fig. 5B). These findings suggest that selective COX inhibitors suppressed MDR1/P-gp expression in taxane-resistant ovarian cancer cells.
The aim of this study was to investigate whether the selective COX inhibitors promote paclitaxel-induced cytotoxicity in taxane-resistant ovarian cancer cells by suppressing MDR1/P-gp expression, and to clarify whether these effects depend on the COX pathway. We found that the combined treatment with paclitaxel and a selective COX inhibitor promoted cytotoxicity in taxane-resistant ovarian cancers by suppressing MDR1 gene and P-gp protein expression (Fig. 5). Our results correspond well with previous studies that reported that COX inhibitors significantly enhanced the cytotoxic effects of chemotherapeutic agents in drug-resistant cancer cells by inhibiting P-gp expression [11,12,15-17].
In this study, we documented that P-gp is overexpressed in the taxane-resistant ovarian cancer cell lines, HeyA8-MDR and SKOV3ip2-TR, but not in the taxane-sensitive cell lines, HeyA8 and SKOV3ip1. COX expression, however, was inconsistent among these cell lines. COX-2 was only expressed in one taxane-resistant cell line, SKOV3ip2-TR (Fig. 1). Because taxane-resistant ovarian cancer cells did not express significantly more COX than taxane-sensitive ovarian cancer cells, the inhibitory effect of selective COX inhibitors on MDR1/P-gp expression may not be mediated through the COX pathway. Thus, COX inhibitors may increase intracellular accumulation of paclitaxel and subsequently enhance cytotoxicity by directly suppressing P-gp expression, without depending on the COX pathway.
We showed that adding the end product of COX pathway, PGE2, did not reverse the inhibitory effects of selective COX inhibitors on paclitaxel-resistant ovarian cancer cell growth (Fig. 4), which further suggests that the cytotoxic effects of COX inhibitors are COX- and PGE2-independent. These findings are consistent well with previous studies [12,17,18]. Xia et al. [17] reported that a selective COX-2 inhibitor, celecoxib, enhances the drug sensitivity of human breast cancer cells. Ye et al. [12] showed that indomethacin and a selective COX-2 inhibitor enhanced doxorubicin cytotoxicity in human hepatocellular carcinoma cells, by inhibiting P-gp expression in a COX-2-independent manner. However, Roy et al. [11] reports results that are in contrast ours. They suggested that selective COX inhibitors regulate MDR1 expression and potentiate the effects of doxorubicin in human hepatocellular carcinoma cells by altering the activity of ABC proteins in a COX-2 dependent manner. They concluded that the cytotoxicity of COX-2 inhibitors is mediated by down-regulating MDR1 in a PGE2- or COX-dependent manner. Interestingly, our study showed that taxane-resistant ovarian cancer cells did not consistently express more COX-1 or COX-2 than taxane-sensitive ovarian cancer cells. Further, adding PGE2, the end product of the COX pathway, did not reverse the effects of selective COX inhibitors. Xia et al. [17] also suggested that the effects of selective COX inhibitors on P-gp are COX-2-independent but closely related to hypermethylation of the MDR1 promoter. Further studies are required to elucidate the precise mechanisms involved in chemosensitization by COX inhibitors.
With taxane-sensitive ovarian cancer cell line, SKOV-3, Li et al. [19,20] reported that combined treatment of COX inhibitors with taxol has effects on angiogenesis and apoptosis in human ovarian cancer xenografts. They report that COX inhibitor enhances the anti-angiogenic and pro-apoptotic effects of taxol in taxane-sensitive ovarian cancer in vivo, thus they suggest combined treatment of COX inhibitors with taxol in ovarian cancer therapy. Our studies with taxane-sensitive ovarian cell lines were also consistent with theirs (data not shown). The synergistic effects of paclitaxel and COX inhibitor were also seen in taxane-sensitive HeyA8 and SKOV3ip1 cell lines. These findings suggest that COX inhibitor can be a potent therapeutic tools not only as a drug sensitizer, but also as an anti-angiogenic and pro-apoptotic agents.
In conclusion, our data imply that selective COX inhibitors significantly increase paclitaxel-induced cell death in taxane-resistant ovarian cancer cells in a prostaglandin- and COX-independent manner. These findings suggest that combined treatment with selective COX inhibitors and paclitaxel could be a potent therapeutic tool to promote paclitaxel sensitization of taxane-resistant ovarian cancers by suppressing MDR1/P-gp expression, which is responsible for efflux of chemotherapeutic agents. Thus, selective COX inhibitors could be good chemosensitizers to improve chemotherapy.
Figures and Tables
Fig. 1
P-glycoprotein and cyclooxygenase (COX) expression in ovarian cancer cell lines. HMDR, STR, H, and SK represent HeyA8-MDR, SKOV3ip2-TR, HeyA8 and SKOV3ip1, respectively.
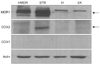
Fig. 2
Treatment of taxane-resistant ovarian cancer cells with selective cyclooxygenase (COX) inhibitors combined with paclitaxel. Cell viability was determined by using the 3-(4,5-dimethylthiazol-2-yl)-2,5-diphenyltetrazolium bromide (MTT) assay. The viability of the vehicle treated cells was considered as 100%. Statistical significance is indicated by asterisks. *p<0.05. (A) HeyA8-MDR, (B) SKOV3ip2-TR cell line.
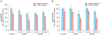
Fig. 3
Selective cyclooxygenase (COX) inhibitors increase apoptosis in taxane-resistant ovarian cancer cells treated with paclitaxel. (A, B) Fluorescence activated cell sorting (FACS) analyses were performed to examine distribution of cells in various cell cycle stages when treated with paclitaxel (100 nM) alone or combined with SC560 (20 µM) or NS398 (100 µM). (C) Increased apoptosis in taxane-resistant ovarian cancer cells treated with paclitaxel combined with a selective COX inhibitor. Taxane-resistant cells were treated with paclitaxel (100 nM) alone or combined with a COX inhibitor (SC560, 20 µM or NS398, 100 µM) and Western blotting for cleaved poly (ADP-ribose) polymerase (PARP) was performed to examine apoptosis.
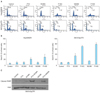
Fig. 4
Adding prostaglandin E2 (PGE2) to ovarian cancer cells treated with paclitaxel combined with SC560 or NS398. PGE2 (10 µM) was added to cultures with paclitaxel (100 nM) and SC560 or NS398 for 72 hours. Cell viability was determined using the MTT assay. The viability of the vehicle treated cells was considered as 100%. Statistical significance is indicated by asterisks. *p<0.05. (A) HeyA8-MDR, (B) SKOV3ip2-TR cell line.
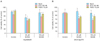
Fig. 5
Reverse transcription polymerase chain reaction (RT-PCR) for MDR1 mRNA and Western blotting for P-glycoprotein (P-gp) protein in HeyA8-MDR and SKOV3ip2-TR cells. HeyA8-MDR and SKOV3ip2-TR cells were treated with paclitaxel, SC560, or NS398, or a combination for 24 hours and cell lysates were harvested for RT-PCR or Western blotting. RT-PCR for 18S rRNA and Western blotting for actin were performed as internal controls. (A) RT-PCR, (B) Western blotting.
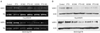
ACKNOWLEDGMENTS
This work was supported by the National Research Foundation of Korea (NRF) grant funded by the Korea government (MEST) (No. 2012R1A1A4A01014504).
References
1. Yap TA, Carden CP, Kaye SB. Beyond, chemotherapy: targeted therapies in ovarian cancer. Nat Rev Cancer. 2009; 9:167–181.
2. Shih KK, Chi DS. Maximal cytoreductive effort in epithelial ovarian cancer surgery. J Gynecol Oncol. 2010; 21:75–80.
3. Balch C, Huang TH, Brown R, Nephew KP. The epigenetics of ovarian cancer drug resistance and resensitization. Am J Obstet Gynecol. 2004; 191:1552–1572.
4. Lage H. An overview of cancer multidrug resistance: a still unsolved problem. Cell Mol Life Sci. 2008; 65:3145–3167.
5. Szakacs G, Paterson JK, Ludwig JA, Booth-Genthe C, Gottesman MM. Targeting multidrug resistance in cancer. Nat Rev Drug Discov. 2006; 5:219–234.
6. Gottesman MM, Fojo T, Bates SE. Multidrug resistance in cancer: role of ATP-dependent transporters. Nat Rev Cancer. 2002; 2:48–58.
7. Zhou SF. Structure, function and regulation of P-glycoprotein and its clinical relevance in drug disposition. Xenobiotica. 2008; 38:802–832.
8. Denkert C, Kobel M, Pest S, Koch I, Berger S, Schwabe M, et al. Expression of cyclooxygenase 2 is an independent prognostic factor in human ovarian carcinoma. Am J Pathol. 2002; 160:893–903.
9. Puhlmann U, Ziemann C, Ruedell G, Vorwerk H, Schaefer D, Langebrake C, et al. Impact of the cyclooxygenase system on doxorubicin-induced functional multidrug resistance 1 overexpression and doxorubicin sensitivity in acute myeloid leukemic HL-60 cells. J Pharmacol Exp Ther. 2005; 312:346–354.
10. Saikawa Y, Sugiura T, Toriumi F, Kubota T, Suganuma K, Isshiki S, et al. Cyclooxygenase-2 gene induction causes CDDP resistance in colon cancer cell line, HCT-15. Anticancer Res. 2004; 24:2723–2728.
11. Roy KR, Reddy GV, Maitreyi L, Agarwal S, Achari C, Vali S, et al. Celecoxib inhibits MDR1 expression through COX-2-dependent mechanism in human hepatocellular carcinoma (HepG2) cell line. Cancer Chemother Pharmacol. 2010; 65:903–911.
12. Ye CG, Wu WK, Yeung JH, Li HT, Li ZJ, Wong CC, et al. Indomethacin and SC236 enhance the cytotoxicity of doxorubicin in human hepatocellular carcinoma cells via inhibiting P-glycoprotein and MRP1 expression. Cancer Lett. 2011; 304:90–96.
13. Gasparini G, Longo R, Sarmiento R, Morabito A. Inhibitors of cyclo-oxygenase 2: a new class of anticancer agents? Lancet Oncol. 2003; 4:605–615.
14. Grosch S, Maier TJ, Schiffmann S, Geisslinger G. Cyclooxygenase-2 (COX-2)-independent anticarcinogenic effects of selective COX-2 inhibitors. J Natl Cancer Inst. 2006; 98:736–747.
15. Zrieki A, Farinotti R, Buyse M. Cyclooxygenase-2 inhibitors prevent trinitrobenzene sulfonic acid-induced P-glycoprotein up-regulation in vitro and in vivo. Eur J Pharmacol. 2010; 636:189–197.
16. Wang CH, Zheng WB, Qiang O, Tang CW. Effects of non-cytotoxic drugs on the growth of multidrug-resistance human gastric carcinoma cell line. J Dig Dis. 2009; 10:91–98.
17. Xia W, Zhao T, Lv J, Xu S, Shi J, Wang S, et al. Celecoxib enhanced the sensitivity of cancer cells to anticancer drugs by inhibition of the expression of P-glycoprotein through a COX-2-independent manner. J Cell Biochem. 2009; 108:181–194.
18. Chuang HC, Kardosh A, Gaffney KJ, Petasis NA, Schonthal AH. COX-2 inhibition is neither necessary nor sufficient for celecoxib to suppress tumor cell proliferation and focus formation in vitro. Mol Cancer. 2008; 7:38.
19. Li W, Zhai L, Tang Y, Cai J, Liu M, Zhang J. Antitumor properties of taxol in combination with cyclooxygenase-1 and cyclooxygenase-2 selective inhibitors on ovarian tumor growth in vivo. Oncol Res. 2012; 20:49–59.
20. Li W, Tang YX, Wan L, Cai JH, Zhang J. Effects of combining Taxol and cyclooxygenase inhibitors on the angiogenesis and apoptosis in human ovarian cancer xenografts. Oncol Lett. 2013; 5:923–928.