Abstract
Objective
Over-expression of thrombin in ovarian cancer cells is associated with poor prognosis. In this study, we investigated the role of thrombin in inducing epithelial-mesenchymal transition (EMT) in SKOV3 epithelial ovarian cancer cells.
Methods
After thrombin treatment SKOV3 cells were subjected to western blots, reverse-transcription PCR, and enzyme-linked immunosorbent assay to quantify EMT-related proteins, mRNA expression of SMAD2, DKK1, and sFRP1, and the secretion of matrix metalloproteinases (MMPs) and cytokines. Meanwhile, invasion ability was evaluated using transwell assays.
Results
The results indicated a dose- and time-dependent down-regulation of E-cadherin and upregulation of N-cadherin and vimentin in thrombin-treated SKOV3 cells, compared with the thrombin-free control group (p<0.05). There was a dose- and time-dependent increase in the levels of SMAD2 and DKK1 mRNAs and a decrease in the levels of sFRP1 mRNA in thrombin-treated SKOV3 cells compared to control cells (p<0.05). Thrombin-treated SKOV3 cells exhibited increased secretion of MMP-9, MMP-2, interleukin (IL)-8, and IL-6 and increased invasion compared to untreated cells (p<0.05). Thrombin altered the morphology of SKOV3 cells to a spindle-like phenotype. Addition of hirudin to thrombin-treated cells reversed the effects of thrombin.
Epithelial ovarian cancer (EOC) is the most common gynecological malignancy worldwide, with a 5 year survival rate of around 45% [1]. Early detection remains a major challenge and more than 70% of EOC patients are diagnosed in the advanced stage of the disease, which is characterized by metastasis to the peritoneal cavity lining [1,2]. Invasion and metastasis are known to be critical determinants of patient mortality in EOC.
The process of epithelial-mesenchymal transition (EMT) plays a critical role in embryogenesis and has been shown to be associated with invasion and metastasis of malignant cells and tumor progression [3]. Additionally, EMT has been reported to 1) stimulate the differentiation of monocytes to induce angiogenesis in EOC [4], 2) induce differentiation of EOC cells to mimic tumor stem cells [5], and 3) play a role in chemoresistance [6] and apoptosis [7] of ovarian carcinoma cells.
EMT, which is driven by a number of growth factors and cytokines, results in a loss of the original polarities and connections of epithelial cells and reorganization of the mesenchymal cell-like cytoskeleton [8]. During EMT, invasion and migration of cancer cells is characterized by an alteration in the expression of a number of proteins including 1) conversion from E-cadherin to N-cadherin expression, 2) conversion from cytokeratin to vimentin expression, and 3) over-expression of the matrix metallo-proteinases (MMPs) [9].
Down-regulation of E-cadherin and up-regulation of vimentin are thought to play a key role in inducing invasion and metastasis [10] and the switch in expression from E-cadherin to N-cadherin is used to monitor EMT during embryogenesis and cancer progression. E-cadherin, a 120 kDa protein which regulates calcium-dependent cell adhesion, was shown to be down-regulated in most EOC tissues compared to benign ovarian tumor tissue and was positively correlated with high cancer grade, peritoneal seeding, and poor survival [11]. These data suggest an important role for EMT in EOC progression.
Cancer activates the coagulation cascade and conversely, coagulation factors play a role in the progression of cancer [12]. A number of genes mediating inflammation and coagulation have been shown to be differentially expressed in the peritoneal tissues of EOC patients compared to benign stroma [13]. Thrombin has been reported to be over-expressed in ovarian cancer cells [14] and is a predictor of poor prognosis in EOC patients [15]. Thrombin interacts with its specific G-protein-coupled, protease activated receptors (PARs) to induce angiogenesis [13], and the thrombin-PAR complex was shown to mediate the release of a number of growth factors, cytokines and extra-cellular matrix (ECM) proteins to promote EOC metastasis [13]. However, it is not clear if thrombin could promote EOC cell invasion by inducing EMT.
In this study, we evaluated the role of thrombin in cell invasion and EMT in SKOV3 human EOC cells. The thrombin-specific inhibitor, hirudin, was used to assess the significance of thrombin in the progression of EOC.
The human EOC cell line, SKOV3, was obtained from ATCC. Cells were maintained in McCoy's 5a medium supplemented with 10% fetal bovine serum and 1% penicillin and streptomycin (all from Invitrogen, Carlsbad, CA, USA). Cells were cultured at 37℃ in the presence of 5% CO2 with humidity. SKOV3 cells were plated in 24-well plates at a density of 1×105/mL, incubated in McCoy's 5a medium overnight, and then incubated with different doses of thrombin (R&D Systems, Minneapolis, MN, USA) with or without the specific thrombin inhibitor, hirudin (Merck, Whitehouse Station, NJ, USA).
SKOV3 cells were treated with thrombin in the presence/absence of hirudin, seeded on a coverglass and cultured for 24 hours at 37℃. The cells were then fixed with 4% formaldehyde in phosphate-buffered saline (PBS) and permeabilized with 0.5% Triton X-100. Cells were blocked with 1% bovine serum albumin solution and then incubated at 37℃ with a 1:50 dilution of anti-E-cadherin, anti-N-cadherin, or anti-vimentin antibodies (Epitomics, Burlingame, CA, USA) for one hour. The cells were washed and incubated with a 1:100 dilution of fluorescent-conjugated secondary antibody (Sigma, St. Louis, MO, USA) for one hour at 37℃ after which the cells were washed and incubated with DAPI at room temperature for 15 minutes. The cells were washed once with 100% methanol, sealed with glycerol buffer and observed under a fluorescence microscope, ECLIPSE E400 (Nikon, Tokyo, Japan).
Total protein was extracted from thrombin/hirudin treated SKOV3 cells using cell lysis buffer (Beyotime, Beijing, China) according to the manufacturer's instructions. Protein concentration was determined using the BCA protein assay kit (Beyotime) and 50 µg protein samples were subjected to sodium dodecyl sulfate-polyacrylamide gel electrophoresis. Proteins were transferred to polyvinylidene difluoride membranes which were blocked with 5% skim milk at room temperature for 30 minutes. The membranes were incubated with a 1:200 dilution of primary antibody at 4℃ temperature overnight, washed and then incubated with a 1:2,000 dilution of secondary antibody (Sigma) at room temperature for 2 hours. Protein expression was detected by chemiluminescence using an ECL kit (GE Healthcare, Little Chalfont, UK) and quantitated using the ImageJ software. Glyceraldehyde 3-phosphate dehydrogenase (GAPDH) protein level was used as internal control.
Total cellular RNA was extracted from thrombin/hirudin treated SKOV3 cells (5×106) using Trizol (Invitrogen) according to the manufacturer's instructions. Reverse transcription was performed on 50 ng of total RNA using the RT Enzyme Mix and Primer Mix kit (Toyobo, Osaka, Japan) as previously described [16]. PCR primer sequences are listed in Table 1. SMAD2, DKK1, and sFRP1 were amplified with 35 cycles and the reaction was within the exponential phase. However, GAPDH was amplified with only 17 cycles due to the abundance of this gene.
Culture medium was harvested from treated SKOV3 cells and the levels of transforming growth factor (TGF)-β1, MMP-9, MMP-2, interleukin (IL)-8, and IL-6 were measured using commercially available enzyme-linked immunosorbent assay (ELISA) kits (R&D Systems) according to the manufacturer's instructions.
Matrigel (BD, Franklin Lakes, NJ, USA) and transwell chambers (Corning Inc., Corning, NY, USA) were prepared according to the manufacturer's instructions. SKOV3 cell suspensions were prepared in serum-free conditioned culture medium and 200 µL of the cell suspensions (1×105/mL) were added to the top wells of invasion chambers. The bottom wells contained 600 µL McCoy's 5A culture medium supplemented with 20% FBS which served as a chemoattractant. SKOV3 cells were incubated for 24 hours at 37℃ in the presence of 5% CO2, after which the non-invading cells were scrubbed from the upper membrane of the chamber. The cells invading the lower membrane of the chamber were fixed with 4% formaldehyde for 20 minutes and then stained with crystal violet for 5 minutes. The invading cells were then rinsed with PBS and 10 randomly selected visual fields were counted under the microscope (10×). Data were expressed as mean±SD.
Continuous variables were compared by one-way analysis of variance (ANOVA). When a significant difference between groups was apparent, multiple comparisons of means were performed using the Bonferroni procedure with type-I error adjustment and Dunnett test. Data are presented as mean±SD. All statistical assessments were two-sided and a p-value of 0.05 was considered significant. Statistical analyses were performed using SPSS ver. 15.0 (SPSS Inc., Chicago, IL, USA).
Using western blots, we showed a dose- and time-dependent change in the expression of EMT-related proteins in thrombin-treated SKOV3 cells. SKOV3 cells treated with 0.5 U/mL, 1.0 U/mL, and 2.0 U/mL of thrombin exhibited a significant decrease in the expression of E-cadherin compared to untreated cells and significant increases in the expression of N-cadherin and vimentin (p<0.05, n=3). Notably, these effects were reversed by the addition to thrombin inhibitor, hirudin, to thrombin-treated SKOV3 cells (Fig. 1A).
SKOV3 cells treated with thrombin (1.0 U/mL) for 12 hours, 24 hours, and 48 hours exhibited a significant decrease in the expression of E-cadherin compared to untreated cells and significant increases in the expression of N-cadherin and vimentin, compared to untreated cells (p<0.05, n=3). Notably, these effects were also reversed by the addition to thrombin inhibitor, hirudin, to thrombin-treated SKOV3 cells (Fig. 1B).
SMAD2, DKK1, and sFRP1 have all been shown to be important regulators of EMT and the Wnt pathway and are related to prognosis of ovarian cancer. We used reverse-transcription PCR to evaluate the expression of these genes in thrombin-treated SKOV3 cells. SKOV3 cells stimulated with 0.5 U/mL, 1.0 U/mL, and 2.0 U/mL of thrombin for 4 hours showed slight increases in the expression of SMAD2 mRNA compared to the control group, and dramatic increases in DKK1 mRNA expression compared to the control group (Fig. 2). However, there were significant decreases in the expression of sFRP1 mRNA compared to the control group (p<0.05, n=3). These effects were reversed in the thrombin plus hirudin group.
We evaluated the secretion of cytokines in thrombin-treated SKOV3 cells and showed that thrombin upregulated the secretion of MMP-9, MMP-2, IL-6, and IL-8 in a time- and dose-dependent manner. SKOV3 cells treated for 24 hours with 0.5 U/mL, 1.0 U/mL, and 2.0 U/mL of thrombin exhibited significant increases in the secretion of MMP-9, MMP-2, IL-8, and IL-6 compared to untreated cells (p<0.05, n=3) (Fig. 3A). These effects were reversed by the addition of hirudin (Fig. 3A). Similarly, SKOV3 cells treated with 1 U/mL of thrombin for 12 hours, 24 hours, and 48 hours exhibited significant increases in the secretion of MMP-9, MMP-2, IL-8, and IL-6 compared to untreated cells (p<0.05, n=3) (Fig. 3B). These effects were reversed by the addition of hirudin (Fig. 3B). In contrast, there was no significant change in the secretion of TGF-β1 in SKOV3 cells treated with thrombin (p>0.05, n=3).
Transwell invasion experiments were performed to evaluate the effect of thrombin on the invasiveness of SKOV3 cells (Fig. 4). SKOV3 cells treated for 24 hours with 1.0 U/mL of thrombin exhibited a significant increase in the number of cells invading the lower chamber (115.00±16.87), compared to the control group (79.80±9.86; p<0.05, n=3). The number of cells invading the lower chamber in the thrombin plus hirudin group was 82.70±10.22, which was not significantly different from the control group.
Nest, the role of thrombin in the morphology of SKOV3 cells was investigated using immunofluorescence staining. The expression of E-cadherin, N-cadherin and vimentin in untreated SKOV3 cells and SKOV3 cells treated with thrombin in the absence and presence of hirudin was detected. We showed that untreated SKOV3 cells were mostly irregular in shape or polygonal (Fig. 5, first row). However, thrombin induced a morphological alteration to a "spindle-shaped" phenotype (Fig. 5, second row), which was reversed by hirudin (Fig. 5, third row).
In this study, we showed that thrombin directly induced EMT in SKOV3 cells and this was associated with a time- and dose-dependent down-regulation of E-cadherin accompanied by an up-regulation of N-cadherin and vimentin. Thrombin also induced a morphological alteration in SKOV3 cells to a "spindle-shaped" phenotype, which may be conducive to invasion and metastasis.
There has been a recent focus on the mechanisms by which coagulation factors influence angiogenesis, tumor growth, metastasis and invasion. Thrombin has been reported to act via the PAR1 receptor to promote the proliferation and migration of lung cancer, breast cancer and colon cancer cells [13]. PAR-1 was shown to be activated by plasmin and to induce ERK signaling to initiate EMT [17]. Alveolar epithelial cell treated with thrombin was reported to upregulate PAR and to induce a morphological change from a cobblestone to an elongated phenotype [18]. Our data were consistent with these studies and showed up-regulation of PAR1, PAR3, and PAR4 in thrombin-treated SKOV3 cells. This was accompanied by an alteration in morphology from an irregular, polygonal to a spindle-shaped phenotype.
The dose-effect of thrombin on cancer cells remains unclear. Low concentrations of thrombin (0.1 to 0.5 U/mL) enhanced the growth of cancer cells in vitro, while high concentrations of thrombin (0.5 to 1 U/mL) induced apoptosis, possibly via activation of distinct signaling networks [19]. However, our data showed that thrombin induced EMT in SKOV3 cells at all the concentrations tested and no apoptosis was observed. It will be interesting to explore in greater detail the molecular mechanisms and signaling pathways regulating thrombin-induced EMT in SKOV3 cells.
The TGF-β1 signaling pathway has been shown to regulate EMT and loss of the TGF-β1 receptor was associated with inhibition of EMT, and inhibition of invasion and metastasis of pancreatic cancer [20]. SMAD2, a substrate of the TGF-β1 receptor superfamily was shown to be necessary for TGF-β1-mediated EMT in human squamous cell carcinoma cells [21]. EMT is also regulated by the Wnt signaling pathway which is dysregulated in a number of cancers, resulting in invasion of cancer cells [22]. The dickkopf proteins (DKKs) are reported to block Wnt signaling by binding with membrane Wnt receptor LRP5 and DKK1 co-receptor Kremen1 [23]. Interestingly, although DKK1 was shown to be down-regulated in colon cancer, gastrointestinal cancers, and liver cancer, and was considered a tumor suppressor [24-26], it was recently shown to be over-expressed in lung cancer and intrahepatic cholangiocarcinoma [27,28]. sFRPs also inhibit Wnt signaling [29] and lack of sFRP1 expression was related with the poor prognosis. We showed a significant increase in SMAD2 and DKK1 mRNA levels and a significant decrease in sFRP1 mRNA levels in thrombin-treated SKOV3 cells, suggesting that thrombin activates distinct signaling pathways to induce EMT in SKOV3 cells. It is interesting to note that thrombin-treated SKOV3 cells showed up-regulation of SMAD2, but no alteration in the levels of TGF-β. Our future studies will aim to dissect these pathways in greater detail.
MMPs play a key role in degradation and remodeling of the ECM to drive processes such as embryonic development, reproduction and cancer cell invasion and metastasis [30]. MMP-2 and MMP-9 have been shown to be associated with EOC invasion and metastasis [31,32]. IL-6 and IL-8 are among the interleukins that regulate cell proliferation, angiogenesis and metastasis in EOC and IL-6 is a marker to monitor anti-tumor therapy [33,34]. Our data showed a significant up-regulation in the expression of MMP-2, MMP-9, IL-6, and IL-8 in thrombin-treated SKOV3 cells. Our data expand our understanding of the mechanisms underlying thrombin-mediated EMT in ovarian carcinoma cells.
Anticoagulant drugs such as heparin and warfarin, which prevent fibrin coagulation or platelet aggregation with tumor cells, produced an anti-metastatic effect and improved prognosis [35]. These data suggest an important link between coagulation and carcinogenesis and were consistent with our data which showed that hirudin suppressed thrombin-induced EMT and invasion of SKOV3 cells. Thrombin has previously been shown to regulate the expression of E-cadherin, twist and ZEB1 mRNAs [36-38]. Our future studies will aim to examine whether thrombin regulates the mRNA expression of E-cadherin in this system. To the best of our knowledge, we are the first to suggest that selective anti-coagulant therapy could be used as a novel strategy to treat ovarian carcinoma. In this study, we used SKOV3 cells based on the fact that they are the most commonly used cells in this field [39,40]. However, we do plan to validate our findings in other ovarian cancer cell lines.
In summary, we showed that thrombin induced EMT in human ovarian carcinoma cells. There was a significant up-regulation in the secretion of multiple cytokines, and increased invasion of thrombin-treated SKOV3 cells. Increased invasion of thrombin-treated cells could be associated with the change in phenotype from an irregular, polygonal phenotype to a spindle-shaped phenotype. Thrombin antagonist, hirudin, blocked the effects of thrombin, suggesting that coagulation factors play an important role in the progression of ovarian carcinoma and that anticoagulant therapy could be a novel therapeutic strategy for ovarian carcinoma.
Figures and Tables
Fig. 1
Thrombin alters the expression of epithelial-mesenchymal transition (EMT)-related proteins in SKOV3 cells in a dose- and time-dependant manner. (A) Expression of E-cadherin, N-cadherin, vimentin in untreated and SKOV3 cells treated with different doses of thrombin for 24 hours. Control, thrombin-free group; 0.25T-2.0T, different dose (U/mL) of thrombin; 1.0T+H, thrombin plus hirudin (1.0 U/mL) group; GAPDH, glyceraldehyde 3-phosphate dehydrogenase (internal control). (B) Expression of E-cadherin, N-cadherin, vimentin in untreated and SKOV3 cells treated with 1.0 U/mL of thrombin for different times. 0-48 hr, different time points of stimulation; 24 hr+H, thrombin plus hirudin group. The statistical results of (A) or (B) were shown below the corresponding representative image. *p<0.05 vs. the corresponding control.
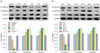
Fig. 2
Thrombin regulates the expression of genes involved in the transforming growth factor (TGF)-β and Wnt signaling pathways. (A) Expression of SMAD2 mRNA, DKK1 mRNA, and sFRP1 mRNA in SKOV3 cells treated with thrombin for 4 hours. Control, thrombin-free group; 0.25T-2.0T, cells treated with different doses (U/mL) of thrombin; 1.0T+H, thrombin plus hirudin (1.0 U/mL) group; GAPDH, glyceraldehyde 3-phosphate dehydrogenase (internal control). (B) Statistical analysis of the mRNA expression data. *p<0.05 vs. the corresponding control.
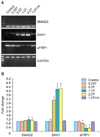
Fig. 3
Thrombin promotes the secretion of matrix metallo-proteinases (MMPs) and cytokines in SKOV3 cells. (A) Statistical results of MMP and cytokine expression in SKOV3 cells treated with different doses of thrombin for 24 hours. (B) Statistical results of MMP and cytokine expression in SKOV3 cells treated with thrombin (1.0 U/mL) for different time periods. *p<0.05 vs. the corresponding control. IL, interleukin; TGF, transforming growth factor.
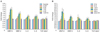
Fig. 4
Transwell invasion assay of SKOV3 cells (×10). Thrombin promotes invasion of SKOV3 cells in thrombin stimulation group (1.0 U/mL) (B) compared with control group (A) and thrombin plus hirudin group (C). (D) Statistical analysis of the different groups. *p<0.05 vs. the corresponding control. †p<0.05 vs. thrombin group.
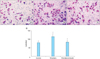
Fig. 5
Thrombin induces alteration in morphology of SKOV3 cells. SKOV3 cells were non-treated (before epithelial-mesenchymal transition, first row), treated with thrombin (second row), or treated with thrombin and hirudin (third row). Cells were stained with E-cadherin (red, first column from the left), N-cadherin (green, second column), or vimentin (red, third column). All cells were co-stained with DAPI (×200).
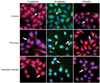
ACKNOWLEDGEMENT
This work was supported by grants from Shanghai Health Bureau Key Disciplines and Specialties Foundation, Shanghai Education Commission Key Disciplines Foundation, Key Discipline Project of Ren Ji Hospital, School of Medicine, Shanghai Jiao Tong University.
References
1. Jemal A, Siegel R, Ward E, Hao Y, Xu J, Murray T, et al. Cancer statistics, 2008. CA Cancer J Clin. 2008; 58:71–96.
2. Sarojini S, Tamir A, Lim H, Li S, Zhang S, Goy A, et al. Early detection biomarkers for ovarian cancer. J Oncol. 2012; 2012:709049.
3. Thiery JP, Acloque H, Huang RY, Nieto MA. Epithelial-mesenchymal transitions in development and disease. Cell. 2009; 139:871–890.
4. Collino F, Revelli A, Massobrio M, Katsaros D, Schmitt-Ney M, Camussi G, et al. Epithelial-mesenchymal transition of ovarian tumor cells induces an angiogenic monocyte cell population. Exp Cell Res. 2009; 315:2982–2994.
5. Mani SA, Guo W, Liao MJ, Eaton EN, Ayyanan A, Zhou AY, et al. The epithelial-mesenchymal transition generates cells with properties of stem cells. Cell. 2008; 133:704–715.
6. Kajiyama H, Shibata K, Terauchi M, Yamashita M, Ino K, Nawa A, et al. Chemoresistance to paclitaxel induces epithelial-mesenchymal transition and enhances metastatic potential for epithelial ovarian carcinoma cells. Int J Oncol. 2007; 31:277–283.
7. Kurrey NK, Jalgaonkar SP, Joglekar AV, Ghanate AD, Chaskar PD, Doiphode RY, et al. Snail and slug mediate radioresistance and chemoresistance by antagonizing p53-mediated apoptosis and acquiring a stem-like phenotype in ovarian cancer cells. Stem Cells. 2009; 27:2059–2068.
8. Guarino M, Rubino B, Ballabio G. The role of epithelial-mesenchymal transition in cancer pathology. Pathology. 2007; 39:305–318.
9. Kalluri R. EMT: when epithelial cells decide to become mesenchymal-like cells. J Clin Invest. 2009; 119:1417–1419.
10. Huber MA, Kraut N, Beug H. Molecular requirements for epithelial-mesenchymal transition during tumor progression. Curr Opin Cell Biol. 2005; 17:548–558.
11. Cho EY, Choi Y, Chae SW, Sohn JH, Ahn GH. Immunohistochemical study of the expression of adhesion molecules in ovarian serous neoplasms. Pathol Int. 2006; 56:62–70.
12. Franchini M, Montagnana M, Targher G, Manzato F, Lippi G. Pathogenesis, clinical and laboratory aspects of thrombosis in cancer. J Thromb Thrombolysis. 2007; 24:29–38.
13. Wang X, Wang E, Kavanagh JJ, Freedman RS. Ovarian cancer, the coagulation pathway, and inflammation. J Transl Med. 2005; 3:25.
14. Yousef GM, Polymeris ME, Yacoub GM, Scorilas A, Soosaipillai A, Popalis C, et al. Parallel overexpression of seven kallikrein genes in ovarian cancer. Cancer Res. 2003; 63:2223–2227.
15. Egan K, Crowley D, Smyth P, O'Toole S, Spillane C, Martin C, et al. Platelet adhesion and degranulation induce pro-survival and pro-angiogenic signalling in ovarian cancer cells. PLoS One. 2011; 6:e26125.
16. Heller RS, Klein T, Ling Z, Heimberg H, Katoh M, Madsen OD, et al. Expression of Wnt, Frizzled, sFRP, and DKK genes in adult human pancreas. Gene Expr. 2003; 11:141–147.
17. Zhang G, Kernan KA, Collins SJ, Cai X, Lopez-Guisa JM, Degen JL, et al. Plasmin(ogen) promotes renal interstitial fibrosis by promoting epithelial-to-mesenchymal transition: role of plasmin-activated signals. J Am Soc Nephrol. 2007; 18:846–859.
18. Ando S, Otani H, Yagi Y, Kawai K, Araki H, Fukuhara S, et al. Proteinase-activated receptor 4 stimulation-induced epithelial-mesenchymal transition in alveolar epithelial cells. Respir Res. 2007; 8:31.
19. Zain J, Huang YQ, Feng X, Nierodzik ML, Li JJ, Karpatkin S. Concentration-dependent dual effect of thrombin on impaired growth/apoptosis or mitogenesis in tumor cells. Blood. 2000; 95:3133–3138.
20. Gordon KJ, Dong M, Chislock EM, Fields TA, Blobe GC. Loss of type III transforming growth factor beta receptor expression increases motility and invasiveness associated with epithelial to mesenchymal transition during pancreatic cancer progression. Carcinogenesis. 2008; 29:252–262.
21. Oft M, Akhurst RJ, Balmain A. Metastasis is driven by sequential elevation of H-ras and Smad2 levels. Nat Cell Biol. 2002; 4:487–494.
22. Ren D, Minami Y, Nishita M. Critical role of Wnt5a-Ror2 signaling in motility and invasiveness of carcinoma cells following Snail-mediated epithelial-mesenchymal transition. Genes Cells. 2011; 16:304–315.
23. Smadja DM, d'Audigier C, Weiswald LB, Badoual C, Dangles-Marie V, Mauge L, et al. The Wnt antagonist Dickkopf-1 increases endothelial progenitor cell angiogenic potential. Arterioscler Thromb Vasc Biol. 2010; 30:2544–2552.
24. Gonzalez-Sancho JM, Aguilera O, Garcia JM, Pendas-Franco N, Pena C, Cal S, et al. The Wnt antagonist DICKKOPF-1 gene is a downstream target of beta-catenin/TCF and is downregulated in human colon cancer. Oncogene. 2005; 24:1098–1103.
25. Maehata T, Taniguchi H, Yamamoto H, Nosho K, Adachi Y, Miyamoto N, et al. Transcriptional silencing of Dickkopf gene family by CpG island hypermethylation in human gastrointestinal cancer. World J Gastroenterol. 2008; 14:2702–2714.
26. Qin X, Zhang H, Zhou X, Wang C, Zhang X, Ye L. Proliferation and migration mediated by Dkk-1/Wnt/beta-catenin cascade in a model of hepatocellular carcinoma cells. Transl Res. 2007; 150:281–294.
27. Sheng SL, Huang G, Yu B, Qin WX. Clinical significance and prognostic value of serum Dickkopf-1 concentrations in patients with lung cancer. Clin Chem. 2009; 55:1656–1664.
28. Shi RY, Yang XR, Shen QJ, Yang LX, Xu Y, Qiu SJ, et al. High expression of Dickkopf-related protein 1 is related to lymphatic metastasis and indicates poor prognosis in intrahepatic cholangiocarcinoma patients after surgery. Cancer. 2013; 119:993–1003.
29. Enomoto-Iwamoto M, Kitagaki J, Koyama E, Tamamura Y, Wu C, Kanatani N, et al. The Wnt antagonist Frzb-1 regulates chondrocyte maturation and long bone development during limb skeletogenesis. Dev Biol. 2002; 251:142–156.
30. Malemud CJ. Matrix metalloproteinases (MMPs) in health and disease: an overview. Front Biosci. 2006; 11:1696–1701.
31. Law AY, Wong CK. Stanniocalcin-2 promotes epithelial-mesenchymal transition and invasiveness in hypoxic human ovarian cancer cells. Exp Cell Res. 2010; 316:3425–3434.
32. Chakravarty D, Roy SS, Babu CR, Dandamudi R, Curiel TJ, Vivas-Mejia P, et al. Therapeutic targeting of PELP1 prevents ovarian cancer growth and metastasis. Clin Cancer Res. 2011; 17:2250–2259.
33. Merritt WM, Lin YG, Spannuth WA, Fletcher MS, Kamat AA, Han LY, et al. Effect of interleukin-8 gene silencing with liposome-encapsulated small interfering RNA on ovarian cancer cell growth. J Natl Cancer Inst. 2008; 100:359–372.
34. Grivennikov S, Karin M. Autocrine IL-6 signaling: a key event in tumorigenesis? Cancer Cell. 2008; 13:7–9.
35. Bobek V, Kovarik J. Antitumor and antimetastatic effect of warfarin and heparins. Biomed Pharmacother. 2004; 58:213–219.
36. Palma-Nicolas JP, Lopez-Colome AM. Thrombin induces slug-mediated E-cadherin transcriptional repression and the parallel up-regulation of N-cadherin by a transcription-independent mechanism in RPE cells. J Cell Physiol. 2013; 228:581–589.
37. Chang LH, Chen CH, Huang DY, Pai HC, Pan SL, Teng CM. Thrombin induces expression of twist and cell motility via the hypoxia-inducible factor-1alpha translational pathway in colorectal cancer cells. J Cell Physiol. 2011; 226:1060–1068.
38. Huang MT, Wei PL, Liu JJ, Liu DZ, Huey-Chun H, An J, et al. Knockdown of thrombomodulin enhances HCC cell migration through increase of ZEB1 and decrease of E-cadherin gene expression. Ann Surg Oncol. 2010; 17:3379–3385.
39. Wang J, Jin L, Li X, Deng H, Chen Y, Lian Q, et al. Gossypol induces apoptosis in ovarian cancer cells through oxidative stress. Mol Biosyst. 2013; 9:1489–1497.
40. Ma Y, Weimer J, Fredrik R, Adam-Klages S, Sebens S, Caliebe A, et al. Aurora kinase inhibitor AZD1152 has an additional effect of platinum on a sequential application at the human ovarian cancer cell line SKOV3. Arch Gynecol Obstet. 2013; 288:173–182.