Abstract
Objective
The BCL2 family proteins are critical mediators of cellular apoptosis and, as such, have been implicated as determinants of cancer cell chemo-sensitivity. Recently, it has been demonstrated that the phosphorylation status of the BCL2 antagonist of cell death (BAD) protein may influence ovarian cancer (OVCA) cell sensitivity to cisplatin. Here, we sought to evaluate how kinase and phosphatase components of the BAD apoptosis pathway influence OVCA chemo-sensitivity.
Methods
Protein levels of cyclin-dependent kinase 1 (CDK1) and protein phosphatase 2C (PP2C) were measured by immunofluorescence in a series of 64 primary advanced-stage serous OVCA patient samples. In parallel, levels of cAMP-dependent protein kinase (PKA), AKT, and PP2C were quantified by Western blot analysis in paired mother/daughter platinum-sensitive/resistant OVCA cell lines (A2008/C13, A2780S/A2780CP, Chi/ChiR). BAD pathway kinase CDK1 was depleted using siRNA transfection, and the influence on BAD phosphorylation and cisplatin-induced apoptosis was evaluated.
Results
OVCA patient samples that demonstrated complete responses to primary platinum-based therapy demonstrated 4-fold higher CDK1 (p<0.0001) and 2-fold lower PP2C (p=0.14) protein levels than samples that demonstrated incomplete responses. Protein levels of PP2C were lower in the platinum-resistant versus that shown in the platinum-sensitive OVCA cell line sub-clones. Levels of PKA were higher in all platinum-resistant than in platinum-sensitive OVCA cell line sub-clones. Selective siRNA depletion of CDK1 increased sensitivity to cisplatin-induced apoptosis (p<0.002).
The development of chemo-resistance has a dramatic influence on survival for patients with ovarian cancer (OVCA). Although patients with OVCA demonstrate remarkable sensitivity to platinum-based chemotherapy during primary therapy, the majority of patients eventually develop recurrent or persistent disease that becomes resistant to platinum-based treatment [1,2]. Patients with platinum-resistant disease are treated with a selection of agents, including pegylated liposomal encapsulated doxorubicin, topotecan, taxanes, gemcitabine, etoposide, and cyclophosphamide [1-3]. Despite this array of available drugs, once platinum resistance has developed, few active therapeutic options exist and patient survival is generally short-lived [3]. In this context, platinum resistance is frequently viewed as a surrogate marker for more generic chemo-resistance. As such, targeted therapies that enhance cancer cell sensitivity to cytotoxic agents offer significant potential to improve outcome. Although changes in cellular drug efflux, increased cellular glutathione levels, increased DNA repair, and drug tolerance have all been shown to contribute to platinum-resistance, the fundamental mechanisms that drive OVCA chemo-resistance remain to be completely defined [4-6].
Recently, it has been suggested that cisplatin-induced DNA damage leads to phosphorylation of the BCL2 antagonist of cell death (BAD) protein at the serine-112 and serine-136 residues and that inhibition of phosphorylation at these sites (via inhibition of ERK and/or AKT pathways) increases OVCA cell sensitivity to cisplatin- and paclitaxel-induced apoptosis [7,8]. In light of these findings, in this study, we sought to further characterize the role of a series of BAD-apoptosis pathway phosphatases and kinases as determinants of OVCA chemo-sensitivity. We therefore examined the association of key BAD pathway protein kinases and phosphatases with OVCA cells in vitro and examined the chemo-resistance of these kinases and phosphatases in in vivo OVCA samples and also evaluated the pathway as a potential therapeutic target to increase OVCA cell response to cisplatin.
Cell lines C13, A2780S, A2780CP, A2008, Chi, and ChiR were kindly provided by Dr. Patricia Kruk (Department of Pathology, University of South Florida College of Medicine, Tampa, FL, USA). Cell lines were cultured in RPMI-1640 supplemented with 10% fetal bovine serum, 1% sodium pyruvate, and 1% nonessential amino acids and maintained at 37℃, 5% CO2. All tissue culture reagents were obtained from Sigma Aldrich (St. Louis, MO, USA).
We evaluated 64 advanced-stage (stage III/IV) serous epithelial OVCA samples, resected at the time of primary surgery, from patients who went on to receive platinum-based primary therapy. All tissues were collected under Internal Review Board-approved protocols and with written informed patient consent. Samples were subjected to histopathologic review by a gynecologic oncology pathologist to confirm diagnosis and to confirm greater than 70% tumor content, before being fixed in formalin and embedded in paraffin. Response to therapy was evaluated from the medical record by a single gynecologic oncologist using standard criteria for patients with measurable disease, based upon WHO guidelines [9]. CA-125 was used to classify responses only in the absence of a measurable lesion; CA-125 response criteria were based on established guidelines [10,11]. A complete response (CR) was defined as a complete disappearance of all measurable and assessable disease or, in the absence of measurable lesions, a normalization of the CA-125 level following adjuvant therapy. An incomplete response (IR) was classified as patients who demonstrated only a partial response, had no response, or progressed during primary therapy. A partial response was considered a 50% or greater reduction in the product obtained from measurement of each bi-dimensional lesion for at least 4 weeks or a drop in the CA-125 level by at least 50% for at least 4 weeks. Disease progression was defined as a 50% or greater increase in the product from any lesion documented within 8 weeks of initiation of therapy, the appearance of any new lesion within 8 weeks of initiation of therapy, or any increase in the CA-125 level from baseline at initiation of therapy. Stable disease was defined as disease not meeting any of the above criteria. Forty-one OVCA patient samples demonstrated a CR and 23 showed an IR to primary platinum-based therapy following surgery.
Slides were de-paraffinized and rehydrated using xylene and then subjected to serial dilutions of ethanol. Slides then underwent heat-induced epitope retrieval by incubation in Tris-EDTA buffer (10 mM Tris base, 1 nM EDTA, 0.05% Tween 20, pH 9.0) for 20 minutes at 100℃. Slides were washed in phosphate-buffered saline (PBS), incubated in blocking serum (0.3% Triton X-100, 0.1% normal goat serum in PBS) for 1 hour at room temperature, and exposed to primary antibody in blocking serum for 48 hours at 4℃. Slides were washed 3 times in PBS, incubated with fluorescent-labeled secondary antibody in blocking serum for 2 hours at room temperature, counterstained, and mounted with Prolong Gold containing DAPI (Invitrogen, Grand Island, NY, USA). Images were acquired as TIFF files using the Zeiss Axio Imager Z1 (Carl Zeiss Inc., Jena, Germany) automated fluorescent scope and analyzed for percent expressing cells using Image Pro Plus software.
Cells were harvested in media using a Cell Lifter (Thermo-Fisher, Suwanee, GA, USA) and then washed with cold PBS, and lysates prepared with SDS lysis buffer (2% SDS, 10% glycerol, 0.06 M Tris, pH 6.8). Lysates were evaluated for protein concentration using the BCA method (Pierce, Rockford, IL, USA). Proteins (50 µg) were separated on 10% SDS-PAGE gels and transferred to nitrocellulose membranes. Membranes were blocked in Tris-buffered saline containing 0.05% Tween 20 (TBST)-5% nonfat milk and incubated with primary antibody in TBST-5% nonfat milk overnight at 4℃. Membranes were washed three times for 10 minutes with TBST and incubated with the appropriate secondary antibody in TBST-5% non-fat milk for 90 minutes at room temperature. Antibody binding was visualized by chemiluminescence on autoradiography film.
RNA duplexes for BAD (catalog no. s1861) were purchased from Applied Biosystems Inc. (Foster City, CA, USA), and cyclin-dependent kinase 1 (CDK1; catalog no. 6376) was purchased from Cell Signaling Technology (Boston, MA, USA). Cells were transfected by electroporation using the Nucleofector transfection kit according to manufacturer's recommendations (Amaxa, Gaithersburg, MD, USA). Cells (4×106) were suspended in 0.1 mL electroporation buffer V containing 1 µM siRNA and pulsed using program X-001. Pulsed cells were resuspended in 0.5 mL of complete media without antibiotics and incubated at 37℃ for 15 minutes before experimentation. The Silencer negative control no. 2 siRNA (catalog no. 4390846, ABI), a nonsense siRNA duplex, was used as a control. A2780S was selected based upon our significant experience with it as a model for chemosensitivity and for functional analyses associated with targeted therapeutic interventions.
Percent apoptotic nuclei were determined by morphologic assessment of condensed chromatin and fragmented DNA. Cells were harvested in media using a Cell Lifter (Thermo-Fisher, Suwanee, GA, USA), washed with cold PBS, and fixed in 4% paraformaldehyde for 10 minutes at room temperature. Cell nuclei were stained with 0.5 µg/mL of bis-benzimide trihydrochloride (Hoechst 33258, Molecular Probes, Eugene, OR, USA) and evaluated by fluorescence microscopy. Two hundred cells were counted per condition and scored for apoptosis (nuclei/all nuclei ×100). Each experiment was repeated at least in triplicate. Error bars were used to indicate standard deviation.
The influences of kinases that phosphorylated BAD at serine-112, -136, and -155 in the sensitivity of OVCA to chemotherapy are well established. However, the role of the BAD protein phosphatase, PP2C, and the BAD protein kinase, CDK1, which acts at -128, is controversial (some studies suggesting it is anti-apoptotic, and some the opposite). We therefore sought to explore the role of CDK1 as well as PP2C in OVCA chemosensitivity. The relationships between the expression levels of BAD pathway kinase (CDK1) and BAD protein phosphatase 2C (PP2C) and OVCA chemosensitivity were examined in 64 primary OVCA patient samples using immunofluorescence analysis. OVCA samples demonstrating IR to primary platinum-based therapy had four-fold higher CDK1 levels (p<0.0001) than OVCAs demonstrating CR (Fig. 1A). In contrast, the PP2C levels were two-fold lower in IR than in CR samples (p=0.14) (Fig. 1B).
Western blot analysis revealed that the platinum-resistant OVCA cells (ChiR and C13) exhibited lower expression levels PP2C (serine-155) than the corresponding platinum-sensitive parental clones (Chi and A2008) (Fig. 2). Consistently, levels of phospho-cAMP-dependent protein kinase (PKA), as a fraction of total PKA, were higher in all platinum-resistant sub-clones (ChiR, A2780CP, and C13) versus that shown in the platinum-sensitive parental lines (Fig. 2). Levels of activated phospho-AKT (as a fraction of total AKT) were higher in platinum-resistant daughter cells (A2780CP and C13) than in their platinum-sensitive parental lines (Fig. 2), although both the Chi and ChiR cell lines expressed very low levels of phospho-AKT.
The effect of BAD pathway kinases and phosphatases on platinum sensitivity may be mediated through the regulation of BAD protein expression or via post-translational modification, such as phosphorylation. To determine the contribution of BAD protein expression to OVCA cell line platinum sensitivity, levels of BAD were depleted using siRNA (Fig. 3A). Depletion of BAD protein significantly increased the percentage of apoptotic nuclei both in the presence and absence of cisplatin when compared to cells transfected with the non-targeting siRNA (p=0.02 and p=0.02, respectively) (Fig. 3B).
To further determine the influence of BAD post-translational phosphorylation on OVCA sensitivity to cisplatin, we evaluated the effect of CDK1 depletion by siRNA. The OVCA cell line A2780S was subjected to transfection with siRNAs to CDK1 in the presence and absence of cisplatin, and the effect on cisplatin-induced cell death (Fig. 4A) was evaluated. After 48 hours of cisplatin treatment, A2780S cells depleted of CDK1 showed a significant increase in the percentage of apoptotic nuclei (p<0.002, respectively) (Fig. 4B).
Few clinical or biologic events impact patient outcome more than the development of resistance to chemotherapy. Although only 30% of patients with advanced-stage epithelial OVCA have de novo (intrinsic) platinum-resistant disease at diagnosis, eventually the majority will develop acquired resistance to platinum-based regimens. The presence of such platinum-resistant disease is frequently viewed as a clinical biomarker that predicts low likelihood of response to other cytotoxic agents, short disease-free intervals, and poor overall survival. Several studies have sought to determine the molecular determinants of chemo-resistance [12-15]. However, to date, a comprehensive understanding of the genes, molecular pathways and the post-translational events that may influence chemo-resistance remains elusive. Jazaeri et al. [14] have suggested that the molecular pathways that govern intrinsic resistance to primary therapy may be different from the pathways activated during acquired chemo-resistance. In contrast, a study by Kim et al. [16] found significant similarities in gene expression signatures derived from intrinsic resistant and acquired resistant tumors samples. These contrasting findings underscore the molecular heterogeneity of cancer development and response to chemotherapy. Using cell line models of chemoresistance, we and others have found that aberrant regulation of apoptosis to be associated with the development of chemoresistance [17,18].
Apoptosis is controlled in part by the BCL2 family of proteins which include the pro-apoptotic (e.g., Bcl-xS, BAD, Bax, Bak) and anti-apoptotic (e.g., Bcl-2, Bcl-xL, MCL-1, A1, BAG-1) family members [19-22]. BAD is known to hetero-dimerize with Bcl-xL and Bcl-2 but not with Bcl-xS, Bax, A1, or Mcl-1. Dimerization of BAD with Bcl-xL results in displacement of Bax and an increase in mitochondrial membrane permeability, which induces apoptosis [23]. BAD is regulated by phosphorylation at three primary sites, including serine-112, -136, and -155. When BAD is phosphorylated, it is unable to heterodimerize with Bcl-2 or Bcl-xL, such that Bcl-xL is released to bind Bax, inhibiting apoptotic function [23]. In this way, BAD phosphorylation status influences Bax displacement from Bcl-xL and ability to induce cell death. BAD phosphorylation at serine-136 is induced by protein kinase B (AKT) [24]. BAD serine-112 is phosphorylated by mitogen-activated protein kinase-activated protein kinase-1 (also called RSK) and PKA, and BAD serine-155 is phosphorylated by PKA, which also inhibits Bcl-xL binding [25-27]. Phosphatases PP1, PP2A, and PPM1 (PP2C/PPM1A), and calcineurin have pro-apoptotic effects believed to be influenced by the de-phosphorylation of BAD [28,29]. In light of data supporting a role for BAD phosphorylation status in determination of apoptosis and survival [30,31], we sought to explore the possibility that BAD pathway kinases and phosphatases that influence BAD phosphorylation may not only influence OVCA cisplatin sensitivity but may represent therapeutic targets to increase human cancer cell sensitivity to cisplatin and other cytotoxic agents.
We demonstrated differential levels of BAD pathway kinase and phosphatase protein levels in platinum-sensitive and platinum-resistant patient samples and cell lines. Our results provide further support for the association between BAD pathway activity and chemotherapy resistance, likely via phosphorylation of the BAD protein. Although levels of BAD protein are similar between platinum-sensitive parental lines and their platinum-resistant sub-clones, siRNAs to the BAD gene induced an increase in apoptosis, both in the presence and absence of cisplatin. Using siRNAs to CDK1, a kinase that phosphorylates BAD, we were able to demonstrate an increase in cisplatin-induced apoptosis. It is unclear from these data whether absolute levels of BAD, versus the proportion of BAD that is phosphorylated, dominate the balance between cellular survival and apoptosis, and also whether the effect is driven by changes to BAD phosphorylation at the serine-128 site, or by CDK1 effects on cell cycle. It is noteworthy that our analysis focuses on relative differences in expression/protein level between chemo-sensitive and - resistant tissues in a qualitative fashion. If PP2C and CDK1 are to be further explored as biomarkers of response, criteria for positivity will need to be established, requiring more quantitative future studies. We also recognize that the low absolute levels of expression may make such studies challenging and limit the potential utility of these markers in clinical practice. Our data demonstrate that the relative levels of differential expression of BAD kinases and phosphatases vary between cell line pairs (Fig. 2), appearing more evident in Chi/Chi-cisR and 2008/C13 than in A2780S/CP cell line pairs. These data suggest that, although the BAD pathway is important in determination of cisplatin-induced apoptosis, likely via the phosphorylation status of the BAD protein, there are multiple other molecular mechanisms that underlie OVCA chemo-sensitivity and also by which changes in BAD phosphorylation can occur. This also further underscores the previously recognized molecular heterogeneity of ovarian cancer development and response to therapy. Our findings underscore the complexity of the role of the BCL2 family of proteins in cell survival and apoptosis, as well as the pro- versus anti-apoptotic influence of the BAD serine-128 site. BAD expression levels and also post-translational events likely influence OVCA cell apoptotic signaling and subsequent sensitivity to cytotoxic challenges. Our data provide additional evidence to support an important role for the BAD apoptotic pathway not only as a potential biomarker of OVCA chemo-resistance but also as an appealing therapeutic target.
Figures and Tables
Fig. 1
Cisplatin-resistant tumor samples express higher levels of BCL2 antagonist of cell death (BAD) pathway kinases and lower levels of BAD pathway phosphatases. Protein levels of the BAD pathway cyclin dependent kinase 1 (CDK1) (A) and protein phosphatase 2C (PP2C) (B) were evaluated by immunofluorescence in 64 primary ovarian cancer samples from tumors that demonstrated a complete response (CR, 41) or incomplete response (IR, 23) to primary platinum therapy. Error bars depict the standard error of the mean.
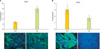
Fig. 2
(A) Western blots showing the relative expression of AKT, phosphorylated AKT (pAKT), PKA, and phosphorylated PKA (pPKA), PP2C, and BCL2 antagonist of cell death (BAD) in the paired cisplatin-sensitive and cisplatin-resistant ovarian cancer cell lines: Chi/ChiR, A2780S/A2780CP, A2008/C13. The expression of GAPDH served as a loading control for each paired cell line. (B) densitometry analysis of the Western blots for each sensitive/resistant cell line pair, where square pixel surface area for each band was normalized to GAPDH expression. Relative protein expression between sensitive and resistant cell line pairs is presented as a percentage of the sensitive cell line.
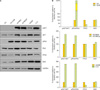
Fig. 3
BCL2 antagonist of cell death (BAD) protein depletion increases cisplatin sensitivity. The OVCA cell line A2780S was evaluated for BAD protein levels by Western blot (A) and apoptotic nuclei in the presence of cisplatin after depletion of BAD protein by siRNA (B). GAPDH served as a protein loading control. A non-targeting (NT) siRNA served as a negative transfection control. Errors bars depict the standard error of the mean.
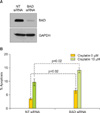
Fig. 4
Depletion of cyclin-dependent kinase 1 (CDK1) protein increases cisplatin sensitivity. The OVCA cell line A2780S was transfected with a non-targeting (NT) siRNA or siRNA specific to CDK1 and evaluated for protein expression by Western blot analysis (A) and percent apoptotic nuclei in the presence of cisplatin (B). GAPDH was used as a protein loading control. Error bars indicate standard error of the mean.
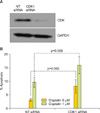
ACKNOWLEDGMENT
This work was funded by the following: the Ocala Royal Dames for Cancer Research Inc., the Phi Beta Psi Sorority, and the Jacquie Liggett Fellowship, Hearing the Ovarian Cancer Whisper (H.O.W.), a Program of Palm Healthcare Foundation. And We thank Rasa Hamilton (Moffitt Cancer Center) for editorial assistance.
References
1. Baker VV. Salvage therapy for recurrent epithelial ovarian cancer. Hematol Oncol Clin North Am. 2003. 17:977–988.
2. Hansen HH, Eisenhauer EA, Hansen M, Neijt JP, Piccart MJ, Sessa C, et al. New cytostatic drugs in ovarian cancer. Ann Oncol. 1993. 4:Suppl 4. 63–70.
3. Herrin VE, Thigpen JT. Chemotherapy for ovarian cancer: current concepts. Semin Surg Oncol. 1999. 17:181–188.
4. Godwin AK, Meister A, O'Dwyer PJ, Huang CS, Hamilton TC, Anderson ME. High resistance to cisplatin in human ovarian cancer cell lines is associated with marked increase of glutathione synthesis. Proc Natl Acad Sci USA. 1992. 89:3070–3074.
5. Johnson SW, Laub PB, Beesley JS, Ozols RF, Hamilton TC. Increased platinum-DNA damage tolerance is associated with cisplatin resistance and cross-resistance to various chemotherapeutic agents in unrelated human ovarian cancer cell lines. Cancer Res. 1997. 57:850–856.
6. Johnson SW, Swiggard PA, Handel LM, Brennan JM, Godwin AK, Ozols RF, et al. Relationship between platinum-DNA adduct formation and removal and cisplatin cytotoxicity in cisplatin-sensitive and -resistant human ovarian cancer cells. Cancer Res. 1994. 54:5911–5916.
7. Hayakawa J, Ohmichi M, Kurachi H, Kanda Y, Hisamoto K, Nishio Y, et al. Inhibition of BAD phosphorylation either at serine 112 via extracellular signal-regulated protein kinase cascade or at serine 136 via Akt cascade sensitizes human ovarian cancer cells to cisplatin. Cancer Res. 2000. 60:5988–5994.
8. Mabuchi S, Ohmichi M, Kimura A, Hisamoto K, Hayakawa J, Nishio Y, et al. Inhibition of phosphorylation of BAD and Raf-1 by Akt sensitizes human ovarian cancer cells to paclitaxel. J Biol Chem. 2002. 277:33490–33500.
9. Miller AB, Hoogstraten B, Staquet M, Winkler A. Reporting results of cancer treatment. Cancer. 1981. 47:207–214.
10. Rustin GJ, Nelstrop AE, Bentzen SM, Piccart MJ, Bertelsen K. Use of tumour markers in monitoring the course of ovarian cancer. Ann Oncol. 1999. 10:Suppl 1. 21–27.
11. Rustin GJ, Nelstrop AE, McClean P, Brady MF, McGuire WP, Hoskins WJ, et al. Defining response of ovarian carcinoma to initial chemotherapy according to serum CA 125. J Clin Oncol. 1996. 14:1545–1551.
12. Benedetti V, Perego P, Luca Beretta G, Corna E, Tinelli S, Righetti SC, et al. Modulation of survival pathways in ovarian carcinoma cell lines resistant to platinum compounds. Mol Cancer Ther. 2008. 7:679–687.
13. Dressman HK, Berchuck A, Chan G, Zhai J, Bild A, Sayer R, et al. An integrated genomic-based approach to individualized treatment of patients with advanced-stage ovarian cancer. J Clin Oncol. 2007. 25:517–525.
14. Jazaeri AA, Awtrey CS, Chandramouli GV, Chuang YE, Khan J, Sotiriou C, et al. Gene expression profiles associated with response to chemotherapy in epithelial ovarian cancers. Clin Cancer Res. 2005. 11:6300–6310.
15. Potti A, Dressman HK, Bild A, Riedel RF, Chan G, Sayer R, et al. Genomic signatures to guide the use of chemotherapeutics. Nat Med. 2006. 12:1294–1300.
16. Kim HK, Choi IJ, Kim CG, Kim HS, Oshima A, Michalowski A, et al. A gene expression signature of acquired chemoresistance to cisplatin and fluorouracil combination chemotherapy in gastric cancer patients. PLoS One. 2011. 6:e16694.
17. Li M, Balch C, Montgomery JS, Jeong M, Chung JH, Yan P, et al. Integrated analysis of DNA methylation and gene expression reveals specific signaling pathways associated with platinum resistance in ovarian cancer. BMC Med Genomics. 2009. 2:34.
18. Marchion DC, Cottrill HM, Xiong Y, Chen N, Bicaku E, Fulp WJ, et al. BAD phosphorylation determines ovarian cancer chemosensitivity and patient survival. Clin Cancer Res. 2011. 17:6356–6366.
19. Danial NN, Korsmeyer SJ. Cell death: critical control points. Cell. 2004. 116:205–219.
20. Dejean LM, Martinez-Caballero S, Guo L, Hughes C, Teijido O, Ducret T, et al. Oligomeric Bax is a component of the putative cytochrome c release channel MAC, mitochondrial apoptosis-induced channel. Mol Biol Cell. 2005. 16:2424–2432.
21. Desagher S, Osen-Sand A, Nichols A, Eskes R, Montessuit S, Lauper S, et al. Bid-induced conformational change of Bax is responsible for mitochondrial cytochrome c release during apoptosis. J Cell Biol. 1999. 144:891–901.
22. Kuwana T, Mackey MR, Perkins G, Ellisman MH, Latterich M, Schneiter R, et al. Bid, Bax, and lipids cooperate to form supramolecular openings in the outer mitochondrial membrane. Cell. 2002. 111:331–342.
23. Yang E, Zha J, Jockel J, Boise LH, Thompson CB, Korsmeyer SJ. Bad, a heterodimeric partner for Bcl-XL and Bcl-2, displaces Bax and promotes cell death. Cell. 1995. 80:285–291.
24. del Peso L, Gonzalez-Garcia M, Page C, Herrera R, Nunez G. Interleukin-3-induced phosphorylation of BAD through the protein kinase Akt. Science. 1997. 278:687–689.
25. Lizcano JM, Morrice N, Cohen P. Regulation of BAD by cAMP-dependent protein kinase is mediated via phosphorylation of a novel site, Ser155. Biochem J. 2000. 349(Pt 2):547–557.
26. Tan Y, Demeter MR, Ruan H, Comb MJ. BAD Ser-155 phosphorylation regulates BAD/Bcl-XL interaction and cell survival. J Biol Chem. 2000. 275:25865–25869.
27. Zhou XM, Liu Y, Payne G, Lutz RJ, Chittenden T. Growth factors inactivate the cell death promoter BAD by phosphorylation of its BH3 domain on Ser155. J Biol Chem. 2000. 275:25046–25051.
28. Klumpp S, Selke D, Krieglstein J. Protein phosphatase type 2C dephosphorylates BAD. Neurochem Int. 2003. 42:555–560.
29. Yang L, Omori K, Suzukawa J, Inagaki C. Calcineurin-mediated BAD Ser155 dephosphorylation in ammonia-induced apoptosis of cultured rat hippocampal neurons. Neurosci Lett. 2004. 357:73–75.
30. Datta SR, Dudek H, Tao X, Masters S, Fu H, Gotoh Y, et al. Akt phosphorylation of BAD couples survival signals to the cell-intrinsic death machinery. Cell. 1997. 91:231–241.
31. Datta SR, Ranger AM, Lin MZ, Sturgill JF, Ma YC, Cowan CW, et al. Survival factor-mediated BAD phosphorylation raises the mitochondrial threshold for apoptosis. Dev Cell. 2002. 3:631–643.