Abstract
Gastric cancer is one of the most common cancers in the world. Animal models have been used to elucidate the details of the molecular mechanisms of various cancers. However, most inbred strains of mice have resistance to gastric carcinogenesis. Helicobacter infection and carcinogen treatment have been used to establish mouse models that exhibit phenotypes similar to those of human gastric cancer. A large number of transgenic and knockout mouse models of gastric cancer have been developed using genetic engineering. A combination of carcinogens and gene manipulation has been applied to facilitate development of advanced gastric cancer; however, it is rare for mouse models of gastric cancer to show aggressive, metastatic phenotypes required for preclinical studies. Here, we review current mouse models of gastric carcinogenesis and provide our perspectives on future developments in this field.
Gastric cancer is the second leading cause of death from cancer worldwide and is associated with a poor prognosis and a high incidence of drug resistance.1,2 The molecular mechanisms that promote gastric carcinogenesis are not yet fully understood. Gastric carcinomas can be divided into intestinal and diffuse types according to histological characteristics.3 Intestinal-type carcinomas, which are thought to be derived from gastric mucosa cells, are histologically differentiated and exhibit well-defined glandular structures with expanding growth patterns developing through sequential stages, including chronic gastritis, atrophy, intestinal metaplasia (IM), spasmolytic polypeptide-expressing metaplasia (SPEM), dysplasia, and submucosal invasion; these changes are typical of precancerous epithelium.4 On the other hand, diffuse-type carcinomas are histologically undifferentiated and have a diffuse infiltrative growth pattern, with tumor developing through a shorter, less well-characterized sequence of events from gastric epithelial cells.5
Abate-Shen6 suggested an association between development and gastric carcinogenesis. Inappropriate activation of specific developmental pathways seems to be involved in the development of IM and intestinal-type gastric carcinomas. An appropriate animal model needs to be developed in order to improve our understanding of the mechanisms involved in gastric cancer and to promote the discovery of novel therapeutic interventions. The gastric anatomy of mice is different from that of humans. In mice, the squamo-columnar junction does not universally approximate the gastro-esophageal junction as it does in normal human anatomy. Moreover, rodents rarely develop spontaneous gastric cancer, although cotton rats (Sigmodon hispidus) and the Z strain of the African rodent Mastomys natalensis exhibit enterochromaffin-like cell carcinoids and develop gastric tumors more frequently.7,8,9,10,11 Thus, studies have concentrated on the development of chemical, infectious, or genetic tools to induce gastric cancer in animals.
Here, we review chemically induced, Helicobacter infection-induced, and genetic models of gastric carcinogenesis and compare their pathological patterns, limitations, and applications to improve our understanding of gastric carcinogenesis.
Establishment of adequate mouse models of gastric cancer is necessary for exploring the mechanisms of gastric tumorigenesis. To this end, researchers have tested the utility of various chemical carcinogens to induce gastric cancer in mice. N-nitroso compounds (NOCs), which are generated in the stomach by anaerobic bacteria following ingestion of nitrates and nitrites, have been studied as cancer inducers. N-methyl-N-nitro-N-nitrosoguanidine (MNNG) has been used to induce stomach tumors in rats. For example, Schoental et al.12 treated rats with MNNG using a stomach tube to induce formation of squamous cell carcinoma in the rat forestomach. Additionally, Sugimura and Fujimura13 generated antropyloric adenocarcinomas with high frequency by administering MNNG orally to rats in drinking water. MNNG was found to be a very potent gastric carcinogen in Mongolian gerbils.14,15 Treatment with 400 ppm MNNG in drinking water for 50 weeks resulted in the development of gastric adenocarcinomas in 63.6% of gerbils.15 However, because of the lack of genetic models using these animals, rats and gerbils have limited applications as model systems, and therefore, the effects of oral administration of nitrosamines has been investigated in inbred strains of mice. However, mice have been shown to have resistance to MNNG-induced gastric carcinogenesis. Indeed, when Balb/c mice were infected with H. heilmannii and administered MNNG in drinking water for 38 weeks, squamous cell carcinomas were found in the mouth and forestomach, but adenocarcinoma was not observed in the glandular stomach.16 The ability of N-methyl-N-nitrosourea (MNU) to induce gastric carcinogenesis in mouse models has also been explored. Biweekly intragastric intubation with 0.5 mg MNU resulted in death of most Balb/c mice due to squamous cell carcinoma in the forestomach. Operative removal of the forestomach prior to MNU treatment helped to promote the development of well-differentiated adenocarcinoma in the glandular stomach, with a 100% incidence rate after 40 weeks of treatment.17
Therefore, while glandular stomach is sensitive to the carcinogenic effects of MNU, this phenotype was not the result of the greater sensitivity of the forestomach to MNU under the investigated treatment conditions (dose and route of administration). Tatematsu et al.18 demonstrated that low-dose MNU (30-120 ppm) given in drinking water was effective without the induction of tumors in the forestomach. The efficiency of tumor induction by MNU depends on its concentration rather than the total intake,19 and MNU in the drinking water at 240 ppm for 5 weeks (every other week) has been shown to induce gastric cancer in six strains of mice.20 Consequently, this protocol is used currently as a standard method for induction of gastric carcinogenesis in mice. MNU-induced tumors in mice are located mainly in the gastric antrum and are uniformly well-differentiated adenocarcinomas.21
The MNU mouse model of gastric cancer has been used for studying various signaling pathways in gastric carcinogenesis, including the roles of p53,22 nuclear factor-kappaB (NF-κB),23 the mitogen-activated protein kinase (MAPK) pathway,24,25 Cox-2,26,27 β-catenin,26 E-cadherin,28 and Kruppel-like factor 4 (KLF4).29 MNU is known to modify amino acids in histone proteins, especially histone H3 lysine residues, leading to chromatin remodeling.30 MNU treatment in mice induces altered expression of Trefoil factor 1 (TFF1), a gastric-specific tumor suppressor gene, through epigenetic modifications similar to those observed in human gastric cancer.31 This suggests that epigenetic effects are likely to constitute a key mechanism of NOC-induced carcinogenesis. While the MNU model does not progress through a classical atrophy-metaplasia-dysplasia sequence, this latter H. pylori-dependent pathway results in achlorhydria with subsequent bacterial overgrowth. NOCs may be generated from nitrates and nitrites in this setting, and thus the argument can be made that the generation of NOCs may play a role in Helicobacter-associated carcinogenesis. Recent studies used a combination of MNU and H. felis infection achieved a very rapid induction of antral gastric cancer31 and induced a high frequency of gastric cancer in H. pylori-infected Mongolian gerbils compared to gerbils receiving MNU only.32,33 Thus, the combination of Helicobacter infection and MNU treatment may, in some ways, mimic the proposed pathogenesis of human antral carcinogenesis.
H. pylori are thought to be the main cause of chronic gastritis. The first animal models of Helicobacter infection-induced carcinogenesis in the gastric mucosa was the ferret model.34,35,36 Ferrets with H. mustalae infection exposed to 100 mg/kg MNNG developed gastric cancer, while H. mustalae-infected ferrets did not,34 although MNNG-induced gastric cancer from H. mustalae-free SPF ferrets was not proven because these animals were not available. H. mustelae-infected ferrets have been shown to develop gastritis, dysplasia, and gastric adenocarcinoma with aging.37 Several H. pylori strains, including G1.1,38 TN2,39 and B128,40 have the ability to colonize Mongolian gerbils and induce gastric adenocarcinoma.
The mouse C57BL/6 strain is remarkably resistant to colonization with various H. pylori strains.41,42 Thus, alternative mouse models of gastric Helicobacter infection have been established using H. felis, a close relative of H. pylori. This strain was isolated from the cat stomach and was shown to readily colonize the mouse stomach.43 Several papers have reported that H. felis can induce severe gastritis and atrophy in mice.43,44,45 H. felis-infected mice show gastric SPEM, dysplasia, and invasive cancer with long observation periods.46,47 Extensive dysplastic lesions are observed in the gastric corpus at the squamocolumnar junction (SCJ) along the lesser curvature, and large polypoidantral tumors develop.48,49
In the H. felis infection model in mice, eradication studies have revealed that inflammation, metaplasia, and dysplasia are reversible when early eradication therapy is applied and that progression to dysplasia can be restricted with eradication therapy at a later time point.50,51 This phenomenon is linked to an epidemiological decrease in the incidence of gastric adenocarcinoma in humans.52,53 Gerbils and INS-GAS mice treated with antibiotics to eradicate H. pylori exhibit arrested progression of gastric lesions.43,54
The Sydney strain of H. pylori (SS1) has been adopted as a useful strain in murine model systems.55 High levels of colonization have been achieved in inbred C57BL/6 mice, while colonization levels in Balb/c, DBA/2, and C3H/He strains were lower. Active gastritis and severe atrophy have been observed with detectable levels of bacteria after 8 months of infection. In a 2-year follow-up, infection with SS1 or other strains (i.e., 119p and G50) did not progress to gastric cancer, although some mice developed gastric lymphoma.56 Infection with H. pylori SS1 did result in the development of carcinomas in situ in C57BL/129 mice after 15 months of infection.49 Moreover, H. pylori infection can also cause gastric cancer in genetically modified mice, as shown in a study of INS-GAS mice.57
Vacuolating cytotoxin (Vac), encoded by the vacA gene, facilitates the pathogenesis of stomach dysplasia. Infection with an adapted H. pylori strain lacking VacA reduces the incidence of gastric carcinoma in a Mongolian gerbil model. While CagA deprivation protects against cancer development by enhancing inflammation, ablation of VacA does not affect inflammation.58 The genetic loci cag and vacA are thought to be related to crucial virulence factors in gastritis. In H. pylori, a 40-kb genomic fragment known as the cag pathogenicity island (cag-PAI) encodes a type IV secretion (TFSS) apparatus for the bacterial protein CagA, particularly in high-risk intestinal-type adenocarcinoma.49 After invasion of CagA into host cells, CagA phosphorylation by host cell kinases induces activation of SHP-2 tyrosine phosphatase, NF-κB signaling, and MAPK signaling.59,60,61
CagA and/or the cag-PAI may play a key role in gastric carcinogenesis. Nevertheless, in mice, cag-negative strains, such as H. felis, display carcinogenic abilities similar to cag-positive H. pylori strains. Moreover, while inactivation of the cagE gene encoding TFSS delays the progression to carcinoma in an H. pylori strain, neoplasia ultimately develops in all INS-GAS mice infected with H. pylori mutant.57 Thus, these results suggest that gastric preneoplasia occurs in mice through host-related factors, such as inflammation or other genetic factors. For example, the C57BL/6 strain is more sensitive to H. felis-induced gastric atrophy than the Balb/c strain because C57BL/6 mice show much higher levels of pro-inflammatory cytokines, such as interferon (IFN)-γ due to increased T-helper-1 (Th1)-dependent immune responses in C57BL/6 mice compared to the Th2-dominant immune response in Balb/c mice.47
Mouse models of Helicobacter infection have been studied to determine the effects of other cofactors in gastric carcinogenesis, such as gender, diet, and co-infection. Gender may be an important factor, as gastric cancer is much more prevalent in men than in women. However, C57BL/6 mice infected with H. felis did not exhibit significant gender-related differences in the incidence of gastric carcinoma.46,57 These data suggested that these models have distinct mechanisms of carcinogenesis. High-salt diets and diets rich in nitrates and nitrites have been associated with an increased risk of gastric cancer. Treatment with MNU prior to H. pylori infection induces more severe preneoplastic changes and increased incidence of gastric cancer.62,63 C57BL/6 mice with SS1 infections and consuming a high-salt diet develop more pronounced gastric atrophy and hyperplasia.64 Concurrent parasitic infection may alter the effects of Helicobacter infection. Indeed, co-infection of C57BL/6 mice with H. felis and the helminth Heligmosomoides polygyrus reduces the severity of gastric atrophy and preneoplastic lesions observed following infection with H. felis alone.65 This response has been shown to be associated with a switch from the usual Th1 immune response to a polarized Th2 response.
Murine models of chronic Helicobacter infection are definitive and reproducible models that can be used to investigate the molecular mechanism of gastric carcinogenesis. However, there are limitations to Helicobacter mouse models, including the limited number of H. pylori strains available, the slow time course for the progression of tumors, the low incidence rate of advanced gastric cancer, and the anatomical differences between humans and mice (Table 1).17,18,19,20,28,31,46,66,67,68,69,70,71
Most genetic models in mice have been established on the C57BL/6 or mixed C57BL/6/129SvJ background. In the models discussed below, there are differences in tumor progression and phenotypes that are dependent on genetic background and gender. As expected for inbred mouse populations, genetic variability in mouse strains produces different susceptibilities to the development of gastric cancers. Therefore, when using particular genetic models of gastric cancer, the use of wild-type control mice with genetic backgrounds identical to the mutant mice is crucial.
The epithelium of the mouse stomach comprises the proximal fundus and the distal antrum, and these tissues have distinct functions. The fundus produces acid for digestive enzymes, while the antrum has an endocrine and mucus-secretory role. Gastric tumors progress independently in separate regions under the control of different genetic triggers. Most of the models summarized in Table 257,70,72,73,74,75,76,77,78,79,80,81,82,83,84,85,86,87,88,89,90,91,92,93,94,95,96,97,98,99,100,101,102,103,104,105,106,107,108,109,110,111,112,113,114,115,116,117,118,119,120,121,122,123,124,125,126,127,128,129,130,131,132,133,134,135,136,137,138,139,140,141 produce various stages of tumors in either the fundus or the antrum, while some model produce tumors in both tissues.
TFF1 (also called pS2) is a member of the trefoil domain peptide family, which also includes TFF2 and TFF3. These peptides are highly expressed in the gut, and TFF1 and TFF2 are synthesized and secreted by surface/pit mucus and mucus neck/astral gland cells, respectively. TFF1-/- mice have gastric astral/pylorus-specific hyperplasia by 1 week of age, and one-third of mice develop dysplasia and multifocal intra-epithelial carcinomas by 20 weeks.72
TFF1-/- tumors have two phenotypes. Tumors with the first phenotype are located in the distal stomach, supporting a role for TFF1 as a stomach-specific tumor-suppressor gene.73 Secondary phenotypes show increased lengths of small intestinal villi with associated lymphocytic infiltrate72 and the loss of neutral glycoprotein from surface and pit cells of the stomach. This phenotype suggests a role for TFF1 in regulating gastric differentiation pathways.
The genes exhibiting the highest levels of overexpression in the stomachs of TFF1-/- mice are claudin 7 (encoding a tight junction protein), early growth response 1 (encoding a nuclear transcription factor), and epithelial membrane protein 1 (encoding a junctional membrane protein).74 Upregulation of claudin 7 has also been observed in preneoplastic lesions in human stomachs and in gastric adenocarcinoma, thus underscoring the utility of the TFF1-/- mouse model in the discovery of genes related to gastric cancer progression.
gp130757F757F mice were generated by knock-in mutation of the SHP2/SOCS3 binding site on the interleukin (IL)-6 family coreceptor gp130 in order to genetically dissect the independent contribution of the two proteins downstream of the signal transducing receptor.75 The tyrosine (Y) residue at position 757 in the intracellular domain of gp130 was changed to a phenylalanine residue in both alleles (757F757F), thereby preventing SHP2 (and SOCS3) docking after ligand binding with the receptor complex and blocking signal transduction through the Ras/extracellular signal-regulated kinase (ERK)/AP-1 signaling pathway. Inhibition of this pathway prevents activation of target genes by AP-1 and promotes signaling via an alternate pathway including IL-6 cytokines, which involves the transcription factor STAT3. Thus, loss of feedback inhibition of STAT3 activation by SHP-2/Ras/ERK and SOCS3 results in constitutive oncogenic signaling by STAT3 dimers. The resulting phenotype is characterized by splenomegaly and rapid gastric tumorigenesis, with downregulation of genes regulated by IL-6 via the SHP-2/Ras/ERK/AP-1 pathway and upregulation of genes mediated by STAT3, including growth factors like Reg176 and anti-apoptotic, pro-angiogenic, and cytostatic genes.77
A principle feature of this model is the phenotypic changes in the intestine characteristic of human gastric adenocarcinoma, including gastritis, atrophy, intestinal-type mucus metaplasia and SPEM, dysplasia, and submucosal invasion, but without metastasis. This tumorigenesis is independent of H. pylori infection, hypergastrinemia (mice are hypogastrinemic), and constitutive activation of epidermal growth factor receptor,76 as required for many other stomach cancer models, and highlights the importance of IL-6 signaling in the maintenance of gastric homeostasis. The timing and site of tumor initiation and dysplastic changes are consistent in all mice; development is rapid, such that initiation of antral tumors with transmural gastritis is observed by 4 weeks of age, and tumor growth then progresses rapidly along the lesser curvature of the stomach to encompass the entire secretory mucosa by 20 weeks of age.76
Cdx1 and Cdx2 are adult intestine/colon-specific transcription factors that play roles as caudal-related homeobox genes during development. These proteins are involved in IM, the intestinalization of the gastric mucosa associated with progression to intestinal-type gastric adenocarcinoma in both mice and humans. When housed under specific pathogen-free (SPF) conditions, Cdx2 transgenic mice in the C57BL/6 background exhibit incomplete IM throughout gastric fundic glands at 12 weeks of age,78 coincident with hypergastrinemia, achlorhydria, and SPEM.79,80 The stomachs from Cdx1 transgenic mice show rapidly expanding IM, similar to that observed in Cdx2 transgenic mice, but with a variety of differentiated cell types, including Paneth cells and hormone-expressing endocrine cells.79,80
Gastrin, which is produced by G cells in the antral mucosa, is a crucial regulatory hormone in the gastric mucosa and can regulate cell division, invasion, angiogenesis, and anti-apoptotic activity at the transcriptional level.142,143 It functions to regulate acid secretion in response to feeding and in maintaining developmental epithelial cell homeostasis in the fundic and antral mucosa. A failure to strictly regulate gastrin expression will induce perturbations in gastric epithelial cell dynamics and potentially promote gastric cancer. Such dysregulation of gastrin has been used in various mouse models of stomach cancer.
Expression of the human gastrin transgene is induced by a mouse insulin promoter, and processed forms of gastrin are found in the pancreas, stomach, and colon.144,145 In 1-year-old INS-GAS mice, marked thickening of the fundic mucosa and multifocal hyperplasia are observed in the stomach in the context of gastrin overexpression,145 and these mice spontaneously develop atrophy and cancer by 2 years of age.81 INS-GAS mice can be used in combination with other agents as a model of gastric cancer development due to the lower threshold for carcinogenesis. At 7 months after infection with H. pylori or H. felis, male INS-GAS mice develop atrophy, IM, dysplasia, and finally, gastric adenocarcinoma.57,81 Cancers develop in situ or intramucosal carcinoma57 by reactivation of sonic hedgehog expression.82 Inhibition of the gastrin/CCK2 and histamine H2 receptors limits the development of gastric cancer in these mice.146
The actin promoter has been used to drive gastrin expression in the Act-Gas transgenic model. Mutation of the gastrin gene allows the expression of processed forms of gastrin by nonendocrine cells.83 By 16 weeks of age, mice develop mucosal hypertrophy, consisting mainly of foveolar hyperplasia, accompanied by parietal cell atrophy.84 Similar to the TFF1-/- model, treatment with a selective COX-2 inhibitor reduces cell proliferation and foveolar thickness, suggesting that COX-2 and prostaglandin E2 (PGE2) might function downstream of gastrin.84
Gastrin-deficient mice are hypochlorhydric due to the absence of the gastrin hydrochloric acid secretory pathway.85,86 The absence of gastric acid provides permissive conditions for bacterial overgrowth in hypochlorhydric mice.87 This overgrowth with inflammation was recovered by treatment with antibiotics. By 12 months of age, hypochlorhydric mice develop chronic gastritis, atrophy, metaplasia, dysplasia, and intramucosal carcinoma in the antral mucosa,88 dependent on mucosal inflammation. The metaplasia that develops in this model is not a true IM (marked by the presence of goblet cells), but is instead a naturalization caused by inflammation-dependent expansion of a mucous cell lineage often seen in mouse gastric metaplasia (SPEM).89,90 Moreover, in this model, the development of carcinomas is independent of gastrin, but is related to increases in the amount of activated STAT3 and loss of RUNX3 expression.88
Parietal cells of the fundic stomach secrete hydrochloric acid to sterilize gastric contents and promote the activation of stomach enzymes for protein digestion. Physical loss of parietal cells or their acid synthetic function promotes bacterial colonization of the gastric lumen, and this, along with the constitutive inflammatory response of the host, likely predisposes the cells to gastric pathology, including cancer. Therefore, it follows that a variety of mutations affecting the acid secretion function of parietal cells will contribute to improving our understanding of the mechanisms predisposing the gastric tissue to metaplasia and cancer in mouse models.
Gastric acid plays a role in minimizing infection and the subsequent inflammatory response in the stomach. The H+/K+-ATPase expressed by fundic parietal cells is responsible for acidification of gastric contents. The mice deficient for the H+/K+-ATPase α subunit exhibit progression of fundic hypertrophy to hyaline transformation, mucocystic and ciliated metaplasia, and chronic gastritis at 20 months of age, particularly in female mice.91
Na+/H+ exchangers (NHEs) are proteins in the basolateral membrane of gastric epithelial cells, particularly parietal cells, and are known to be involved in mediating acid secretion and in maintaining epithelial cell viability. Knockout of NHE292 or NHE493 induces fundic atrophy, parietal cell loss, achlorhydria, hypergastrinemia, and glandular hyperplasia.
Due to the high activity of the H+/K+-ATPase in gastric parietal cells, potassium channels have important roles in the maintenance of ion homeostasis. The fundic mucosas of these mice morphologically resemble those of the H+/K+-ATPase knockout mice because these mice develop achlorhydria, hypergastrinemia, and hyperplasia, although the mechanism has not been directly tested.94 Targeted mutation of Kcnq1 in mice leads to an expanded fundic proliferation zone with severe hyperplasia, achlorhydria, and hypergastrinemia. Several mouse lines with a defective Kcnq1 locus (14Gso) have been generated using random mutagenesis induced by X-ray irradiation of spermatogonia.95 The products of these mutations are similar to those in H+/K+ ATPase α- or β-null mutant mice.
The histamine histamine receptor (H2R) is expressed on acid-secreting parietal cells of the gastric mucosa and functions to stimulate gastric acid secretion. H2R-knockout mice are viable, fertile, and have normal basal gastric acid secretion, maintained by muscarinic receptors.96 Fundic hyperplasia occurs as a direct product of increased numbers of parietal and enterochromaffin-like cell until 17 months of age, after which the pathology worsens to include mucocystic metaplasia, with a proportion of the mice developing herniation of the epithelium penetrating the muscularis mucosa and producing a phenotype closely mimicking Ménétrier's disease in humans.97
Parietal cells in the gastric mucosa show subcellular reorganization upon activation, leading to secretion of gastric acid. Through its F-actin-binding function, IQGAP1 is involved in this subcellular reorganization event, which is dependent on the precise formation of F-actin structures by the Rho family of Ras-related GTPases.98
There are three isoforms of transforming growth factor beta (TGF β): TGF β1, -2, and -3, and all three isoforms can bind to TGF β receptor II, although TGF β1 is most frequently altered in tumorigenesis. Upon ligand binding to TGF β receptor II, heterodimerization and activation of TGF β receptor I occur. Activation of the SMAD complexes via activation of TGF β receptor I can activate TGF β-responsive genes in the nucleus. Many of the downstream components of the TGF β signaling pathway are thought to act as tumor-suppressor proteins, including TGF β receptor I, TGF β receptor II, SMAD2, and SMAD4. Changes in TGF β signaling can stimulate tumor growth, invasion, and metastasis.147,148
TGF β1 suppresses cell growth and tumor development by reducing the expression or activity of TGF β receptors and by altering downstream signaling pathways. This resistance to TGF β1 signaling may represent a significant step in the process of carcinogenesis.99,100 TGF β1 also functions to control the production and degradation of extracellular matrix proteins, as well as cellular differentiation. About 20 days after birth, mice homozygous for the TGF β1-null mutation develop a severe wasting syndrome resulting from multifocal, mixed inflammatory cell infiltration in a variety of tissues, including the stomach. In particular, ulceration, hyperplasia, and nodule formation have been observed in the mucosa of the stomach.99,100 Because this mutation results in early lethality, the effects of TGF β1 knockdown on gastric carcinogenesis have not yet been assessed.
A dominant-negative transgene of the TGF β type II receptor was produced under control of the TFF1 promoter to direct stomach-specific expression.149 These transgenic mice did not respond to TGF β ligands in the stomach, but also did not exhibit gastric abnormalities. However, infection with H. pylori induced the acquisition of a more severe phenotype in the fundus and antrum including greater hyperplasia, inflammation, and dysplasia, as well as intramucosal carcinoma.
SMAD4 belongs to a family of proteins involved in the TGF β signaling cascade. Homozygous SMAD4-knockout mice exhibit embryonic lethality, whereas heterozygotes appear normal up to 1 year of age. SMAD4 mutations have been observed in 50% of patients with familial juvenile polyposis. Moreover, mice in which SMAD4 is conditionally ablated in T cells or epithelia, including the intestinal epithelium, begin to display signs of illness by 3 months of age and exhibit shortened lifespans as a result of pathological changes initiated in the small and large intestine, which eventually develop into invasive and metastatic epithelial cancer.101 However, specific deletion of SMAD4 in epithelial cells is not sufficient to induce these cancers in the gut. SMAD4-deficient T cells exhibit increased IL-6 receptor α expression in the gastric epithelium. In some epithelial cancers, the control of TGF β signaling in the inflammatory cells is crucial for regulating tumor development.
Embryonic liver fodrin (ELF), a novel β-spectrin, is a membrane-associated cytoskeletal component in cellular differentiation. ELF plays a role as an adaptor for SMAD proteins. Loss of ELF disrupts nuclear translocation of SMAD3 and SMAD4. Mice with homozygous ELF4-deficiency die during embryonic development, and mice with heterozygous deletions for both SMAD4 and ELF show a higher incidence of severe gastric lesions than those with mutations in SMAD4 alone.102
Runx proteins act as regulators of gene expression in developmental pathways. Runx3-knockout mice on a C57BL/6 background show reduced viability and do not survive beyond 10 days of age.103 Runx3-knockout mice exhibit thickened gastric mucosa with increased proliferation and decreased apoptosis in the fundic and antral mucosa. In contrast, Runx3-knockout mice in an ICRxMF1 background survive for several months and do not develop gastric hypertrophy or carcinogenesis,104 while mice on either a C57BL/6 or BALB/c background do not survive after birth.105 At 8 months of age, Runx3 mice on an ICRxMF1 background develop marked hyperplasia, glandular atrophy, hyaline degeneration, hyperproliferation, and gastritis in the fundic mucosa.
Wnt signaling plays a major role in determining cell fate and morphogenesis during embryogenesis and maintaining homeostatic control of rapidly repaired tissues in adults. Transgenic overexpression of activated β-catenin, which is stabilized and translocated to the nucleus upon activation of Wnt ligands, is involved in tumorigenesis.106,107 These models have shown that β-catenin is overexpressed in a large number of cells. In order to mimic the process of human carcinogenesis, in which tumors arise from a mutation in single cell, a transgenic model with overexpression of the activated form of β-catenin occurring in sporadic cells was established. However, in this model, few mice developed discrete multifocal dysplastic lesions in the gastric mucosa.
Loss of the APC tumor-suppressor gene is involved in the initiation of colorectal cancer in humans and mice via nuclear accumulation and activation of β-catenin/Tcf target genes. APC mutant mice (codon 1638) develop gastric tumors with low frequency during the aging process.109,110 Gastric pathology associated with the APCmin/+/C57BL/6 background may be infrequently observed because of the shortened lifespan of these mice. Recently, APCmin/+/C57BL/6 mice were shown to develop gastric adenomas of the antrum by 20 weeks of age when maintained under SPF conditions. Microbial infection seems to play a role in the development of some types of pathologies in these mice. Moreover, antral adenomas show hyperplasia and nuclear atypia acquired from the strong expression of Myc, cyclin D1, and β-catenin.110
MTH1 acts as a tumor suppressor by inhibiting the incorporation of 8-oxodGTPase residues into DNA, thereby promoting nucleotide oxidation and transversion during DNA synthesis and inducing carcinogenesis in susceptible tissues. Assessment of organ pathology using 18-month-old MTH1-knockout mice and littermate controls, revealed the presence of lung and liver tumors, stomach adenomatous polyps, and adenocarcinomas in 14% of MTH1-/- mice, but only 4% of wild-type controls.111 Additionally, tumors were more prevalent in males than in females. Cai et al.112 demonstrated that MTH2 has a similar activity profile as MTH1, suggesting the possibility that this gene family may contribute to the inhibition of tumorigenesis by mediating local oxidative damage.
K19-C2mE mice exhibit overexpression of COX-2 and microsomal PGE synthase 1 genes under the control of the cytokeratin 19 gene promoter.113 In the context of PGE2 upregulation, this mouse model develops gastric hyperplasia in a macrophage-dependent manner. Although hyperplasia appears by 12 weeks of age, maximal tumorigenesis is not found until about 50 weeks of age or more. Loss of the pro-inflammatory cytokine IL-1b or the adaptive immune response (Rag2-/-) in the K19-C2mE transgene does not affect tumorigenesis. However, depletion of tumor necrosis factor causes severe delays in inflammation, hyperplasia, and development of TFF2-associated mucous cell SPEM,114 suggesting that pro-inflammatory cytokines are important in gastric metaplasia and oncogenesis. Compared with K19-C2mE mice alone, K19-C2mE×K19-Wnt1 transgenic mice exhibit accelerated formation of gastric tumors, concurrent with severe dysplasia, hyperplasia, inflammation, and submucosal invasion by 20 weeks of age.115
Thrombospondin (TSP) proteins are extracellular calcium-binding proteins that regulate cellular attachment, migration, differentiation, and proliferation.116 Due to the TSP-1-dependent activation of TGF β1, TGF β1-/- and TSP-1-/- mice have similar phenotypes in various tissues, including IM and increased mitosis and hyperplasia of the gastric epithelium after postnatal days 17 to 21.99 Compound TSP-1×aVb6 integrin-null mice develop stomach hyperplasia (21%), gastric papillomas, and squamous cell carcinomas.117
TGF α transgenic mice with transgene overexpression in fundic stomach mucus cells (MT-TGF α) exhibit a phenotype in which giant fundic mucosal folds form as a result of massive cellular hyperplasia and glandular cystic dilation.118,119 This phenotype is reminiscent of the rare human condition Ménétrier's disease, which exhibits elevated TGF α expression.118 In adult TGF α transgenic mice, the surface mucous cell population increases at the expense of both parietal and chief (zymogenic) cells, with the isthmus-located stem cell zone nearer to the base of the glands,120,121,122 and the mucosa become much more fibrotic.123 Thus, the fundic mucosa phenocopies the antralization observed in precancerous metaplasia accompanying antral expression of Pdx1.124 However, invasive gastric tumors with IM are not observed.
Activation of aryl hydrocarbon receptor (AhR) by environmental stimuli, such as dioxins and biphenyls, results in transcriptional activation of genes encoding xenobiotic metabolizing enzymes.125 Endogenous expression of AhR is observed predominantly in the lungs, although mice transgenic for AhR also show expression of the transgene in the thymus, spleen, liver, skin, and stomach. In AhR transgenic mice, cysts are grossly apparent in the fundic gastric mucosa at 3 months of age and develop into dysplastic structures that penetrated the muscularis mucosa into the submucosa and subserosa by 12 months of age, at which point transgene expression results in lethality. The penetrating mucosal cells exhibit a well-differentiated, benign appearance and seem to be invasive rather than a result of herniation.125 Similar lesions have also been observed in laboratory animals following treatment with AhR ligands.125 As cause of lesions, decreased expression of osteopontin has been detected in these fundic lesions in differential gene expression analyses. Because osteopontin is involved in tissue remodeling, invasive lesions may be caused by its altered expression in this mouse model.126 In contrast, H+/K+-ATPase α subunit-knockout mice have elevated levels of osteopontin compared with wild-type mice and exhibit reduced invasion of lesions into the gastric muscularis.93
Kruppel-like factor 4 (Klf4) is an epithelial-specific, zinc finger transcription factor that plays important roles in the regulation of cellular proliferation and differentiation. Klf4-knockout mice exhibit early lethality,127 and mice with Klf4 deletions specifically in the glandular gastric mucosa show epithelial hypertrophy, hyperproliferation, mucous metaplasia, atrophy, and polypoid lesions in the fundus and antrum, but no inflammation, hypergastrinemia, dysplasia, or malignancies.128
The p27Kip1 protein inhibits cyclin-dependent kinases to block cell cycle progression, playing vital roles in cell migration, apoptosis, differentiation, and inflammatory responses. p27Kip1-knockout mice develop mild epithelial hyperplasia at approximately 1 year of age, mucous cell metaplasia, and low-grade dysplasia. After H. pylori infection, these preneoplastic conditions facilitate the development of high-grade dysplasia or intramucosal carcinoma in p27Kip1 mice due to increased cell turnover and an exaggerated inflammatory response.129
Major histocompatibility complex (MHC) class II molecules have major roles in regulating the CD4+ arm of the adaptive immune response. MHC class II-deficient mice are unable to produce a functional CD4+-mediated immune response because MHC class II protein is required for the maturation of CD4+ T cells in the thymus. At 6 months of age, these mice have fundic stomachs with gastrin-dependent mild hyperplasia, including infiltration of granulocytes and macrophages, but no epithelial cell atrophy.130 Thus, these data have shown that persistent activation of the innate immune system can produce hyperplastic changes in the fundic mucosa.
Carbonic anhydrases (CAs) are metalloenzymes containing zinc that are involved in pH regulation. CA IX has tumor-related expression and high catalytic activity and has been shown to function as an adhesion molecule. Moreover, CA IX may function as a pH regulator in the hypoxic tumor mass. CA IX-deficient mice have nonprogressive glandular expansion, restricted to foveolar hyperplasia in the fundus.131 CA IX-knockout mice in the C57BL/6 or BALB/c background or feeding of a high-salt diet produces variations in the observed pathological changes.132 However, deletion of CA IX does not affect gastric acid secretion, serum pH, electrolytes, or gastrin, suggesting that CA IX contributes to the hyperplastic phenotype.
p53 is a transcription factor that acts as a regulator of proliferation, apoptosis, and genomic repair. p53 hemizygous knockout mice have been shown to exhibit a low incidence of spontaneous carcinogenesis (2%) in organs. Infection with H. felis in hemizygous p53-knockout mice leads to an increased proliferative index and growth advantage compared with wild-type mice, but no obvious neoplasia was observed.132 Moreover, wild-type C57BL/6 mice develop early invasive adenocarcinomas at 15 months after infection, with associated metaplasia and a greater inflammatory response. Therefore, hemizygosity of p53 appears to result in depressed Th1 immune responsiveness.150
The SV40 T antigen from the simian virus is a potent transforming agent and oncogene. Its aberrant expression has been used to generate transformations in a number of different cells lines and tissues.
Carcinoembryonic antigen (CEA) is expressed during embryonic development and in various tumors, including colorectal, breast, lung, and pancreatic carcinomas. The CEA promoter has been used to drive the transgenic expression of the SV40 T antigen. Despite detectable levels of SV40 T antigen transgene expression only in the stomach, these animals develop carcinomas, lymphomas, and sarcomas with varying frequencies. Only one transgenic line has been shown to reproducibly develop tumors in the antral stomach; these tumors were poorly differentiated adenocarcinomas that had lost gastric mucin expression. Additionally, these tumors were visible macroscopically from 5 weeks of age, and penetration of tumors in all tissue layers of the stomach invaded and blocked the duodenum, causing death of the mice at approximately 20 weeks of age.70 A mouse adenocarcinoma cell line generated from these gastric adenocarcinomas is currently available.133
When the promoter of the H+/K+-ATPase β subunit is used to direct transgenic expression of the SV40 T antigen, a dramatic increase in number of rare preparietal cells was observed by 12 weeks of age. However, such changes did not lead to differentiation into mature parietal cells.134 Mice with this transgene exhibit hyperplasia in the stomach, accompanied by a reduction in the numbers of mature zymogenic cells and parietal cells. By 40 weeks of age, these mice exhibit various abnormal gastric phenotypes, with progressive hyperplasia, cystic dilations, and focal dysplasia. Moreover, by 1 year of age, all mice have invasive gastric cancer with lymphatic-vascular invasion and associated lymph node and hepatic metastasis. Invasive tumor cells were only weakly positive for SV40 T antigen and negative for the H+/K+-ATPase β subunit. However, typical mucous-glandular structures used as histopathological diagnostic criteria in adenocarcinomas of the human stomach were not maintained. Using transcriptome analysis, researchers have shown that invasive tumor cells can transdifferentiate into neuroendocrine cells based on their expression of dopa decarboxylase, chromogranin A, and tryptophan hydroxylase, as well as increased expression of Sox2, Hey1, and Neuro D1.135 Moreover, use of the H+/K+-ATPase β subunit promoter to induce cultured progenitor cells into mature parietal cells at the nonpermissive temperature for the SV40 T antigen resulted in development of fundic hypertrophy in transgenic mice at 12 weeks of age.136
Forkhead homolog 6 (Fkh6) is expressed in the gastrointestinal tract in the mesenchyme directly adjacent to the endoderm-derived epithelium. Fkh6-knockout mice have progressively worsening gastric pathology from 3 days of age. Stomachs from these mice display epithelial hyperplasia, cyst formation, mucous cell metaplasia, increased cell proliferation, and a diffuse submucosal mesenchyme, as well as a significant reduction in BMP4, which has been implicated in epithelial signaling processes. Thus, this result suggests that BMP4 may be a downstream target of Fkh6.137 The dramatic and rapid changes in phenotype in this knockout mouse highlight the important role of mesenchymal-epithelial cell interactions in the growth and differentiation of the gastric mucosa.
Sonic hedgehog (Shh)-knockout mice die at or shortly after birth. Therefore, analysis must be performed before birth. Such analyses have shown that at 18.5 days of embryonic life, Shh-knockout mice exhibit hyperplastic gastric epithelium with no increase in cell proliferation and occlusion of the duodenum caused by overgrowth of villi. Additionally the stomachs of these mice show evidence of intestinalization due to increased expression of intestinal alkaline phosphatase, a marker of the brush border of enterocytes.138
Occludin is a functional component of tight junctions, which are involved in cell-cell adhesion and maintaining the integrity of intercellular spaces. Occludin-knockout mice do not exhibit changes in viability, but have significant reductions in body weight. While deletion of occludin does not affect the morphology, protein content, or function of tight junctions in intestinal epithelial cells, occludin-knockout mice exhibit atrophy of the fundic mucosa by 3 to 6 weeks of age.139 Moreover, gastritis develops progressively, and the fundic mucosa becomes hyperplastic with mucous cell metaplasia by 40 weeks of age.
CCR7 is a chemokine receptor that regulates the trafficking and retention of leucocytes in secondary lymphoid organs. Loss of expression of CCR7 leads to a number of phenotypes, including accumulation of functional lymphoid follicles in the stomachs of mice at 8 to 10 week of age, with concomitant development of the gastric mucosa, including accumulation of cells in the mucous neck region.139 At 12 month of age, profound hyperplasia is observed, with cystic dilatation reminiscent of Ménétrier's disease. As a result, differentiation and proliferation of fundic tissue are affected by the presence of nonspecific, noninflammatory lymphoid aggregates in the gastric mucosa.
Supporting the role of the inflammatory system in regulating the gastric mucosa, loss of the COOH terminus of NF-κB2, an important transcription factor mediating inflammatory signals, has been shown to stimulate activation of Rel/NF-κB transcription factors.141 These mice have antral epithelial tissue with severe hyperplasia at 3 weeks of age, resulting in premature death.151,152,153,154,155,156,157,158,159,160,161,162,163,164,165,166,167,168 It is unclear which genes are affected by the increased activation of NF-κB, leading to this gastric phenotype. Additional studies are required to elucidate the details of these mechanisms.
H. pylori-related gastric cancer in humans is preceded by chronic gastritis, gastric atrophy, IM, and dysplasia. Thus, in addition to mouse models of gastric cancer, there are a number of genetically engineered models exhibiting decreased numbers of parietal cells or gastric atrophy, along with metaplasia. Several models of atrophy and metaplasia can be considered for use in experimental studies (Table 3).49,80,94,121,123,124,132,151,152,153,154,155,156,157,158,159,160,161,162 However, most of these models do not show the progression to neoplasia and most have not been examined to assess susceptibility to cancer in response to carcinogens.
Numerous mouse models with various gastric phenotypes are now available for studies of gastric carcinogenesis. These include transgenic mice, knockout mice, Helicobacter infection, and carcinogen (MNU) models. These models have demonstrated that gender, diet, bacterial flora, inflammatory cytokines, T helper immune response, acid secretion, virulence, colonization properties of H. pylori strains, and host genetic background may all have roles in mediating the development of gastric cancer.
Unfortunately, genetic models of metastatic gastric cancer similar to those developed for pancreatic cancer, comprising two or three mutations targeted to specific cell lineages, are not available. The major limitations of these models are minimal and include dispersion of promoter activity in the stomach and the lack of stomach-specific promoters that target antral progenitors only (Table 4).72,73,74,79,160,163,164,165,166,167,168
Reasonable mouse models of gastric cancer are available for studies of early-stage pathogenesis and cancer therapy, which have distinct mechanisms and different tumor phenotypes, with variations in the time course, location, and pathology of the disease. Thus, researchers are able to utilize appropriate mouse models for their studies. Newly suggested research methods, including lineage tracing or genome-wide analysis, should prove valuable for understanding the causes of gastric cancer, and thereby facilitating the discovery of a cure for this disease.
Figures and Tables
Table 1
Mouse models of gastric cancer by chemical treatment or/and infections of helicobacter and viruses
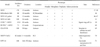
Table 2
Pathologic development in the stomach examplified by various genetic models of gastric hyperplasia or tumoriogenesis in mice
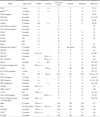
TFF1 = Trefoil factor 1; NHE = Na+/H+ exchanger; TGF = transforming growth factor; TSP = thrombospondin; AhR = aryl hydrocarbon receptor; Klf4 = Kruppel-like factor 4; MHC = major histocompatibility complex; CA = carbonic anhydrase; CEA = carcinoembryonic antigen; Fkh6 = forkhead homolog 6; Shh = Sonic hedgehog; NF-κB2 = nuclear factor-kappa B2; ND = not detectable; Y = yes; N = no.
Acknowledgments
This study was supported by a faculty research grant from Yonsei University College of Medicine for 2013 (6-2013-0061), a new faculty research seed money grant from Yonsei University College of Medicine for 2013 (2013-32-0031) to Ki Taek Nam, and the Brain Korea 21 PLUS Project for Medical Science from Yonsei University.
This research was supported by the Bio & Medical Technology Development Program of the National Research Foundation (NRF) funded by the Ministry of Science, ICT & Future Planning (2013072551).
References
1. Ferlay J, Shin HR, Bray F, Forman D, Mathers C, Parkin DM. Estimates of worldwide burden of cancer in 2008: GLOBOCAN 2008. Int J Cancer. 2010; 127:2893–2917.


2. Jemal A, Bray F, Center MM, Ferlay J, Ward E, Forman D. Global cancer statistics. CA Cancer J Clin. 2011; 61:69–90.


3. Lauren P. The two histological main types of gastric carcinoma: diffuse and so-called intestinal-type carcinoma. An attempt at a histo-clinical classification. Acta Pathol Microbiol Scand. 1965; 64:31–49.
4. Correa P. Human gastric carcinogenesis: a multistep and multifactorial process--First American Cancer Society Award Lecture on Cancer Epidemiology and Prevention. Cancer Res. 1992; 52:6735–6740.
5. Yuasa Y. Control of gut differentiation and intestinal-type gastric carcinogenesis. Nat Rev Cancer. 2003; 3:592–600.


6. Abate-Shen C. Deregulated homeobox gene expression in cancer: cause or consequence? Nat Rev Cancer. 2002; 2:777–785.


7. Kawase S, Ishikura H. Female-predominant occurrence of spontaneous gastric adenocarcinoma in cotton rats. Lab Anim Sci. 1995; 45:244–248.
8. Waldum HL, Rørvik H, Falkmer S, Kawase S. Neuroendocrine (ECL cell) differentiation of spontaneous gastric carcinomas of cotton rats (Sigmodon hispidus). Lab Anim Sci. 1999; 49:241–247.
9. Cui G, Qvigstad G, Falkmer S, Sandvik AK, Kawase S, Waldum HL. Spontaneous ECLomas in cotton rats (Sigmodon hispidus): tumours occurring in hypoacidic/hypergastrinaemic animals with normal parietal cells. Carcinogenesis. 2000; 21:23–27.


10. Koga T, Takahashi K, Sato K, Kikuchi I, Okazaki Y, Miura T, et al. The effect of colonisation by Helicobacter pylori in Praomys (Mastomys) natalensis on the incidence of carcinoids. J Med Microbiol. 2002; 51:777–785.


11. Kumazawa H, Takagi H, Sudo K, Nakamura W, Hosoda S. Adenocarcinoma and carcinoid developing spontaneously in the stomach of mutant strains of mastomys natalensis. Virchows Arch A Pathol Anat Histopathol. 1989; 416:141–151.


12. Schoental R. Carcinogenic activity of N-methyl-N-nitroso-N'-nitroguanidine. Nature. 1966; 209:726–727.


13. Sugimura T, Fujimura S. Tumour production in glandular stomach of rat by N-methyl-N'-nitro-N-nitrosoguanidine. Nature. 1967; 216:943–944.


14. Ohgaki H, Kawachi T, Matsukura N, Morino K, Miyamoto M, Sugimura T. Genetic control of susceptibility of rats to gastric carcinoma. Cancer Res. 1983; 43:3663–3667.
15. Tatematsu M, Yamamoto M, Shimizu N, Yoshikawa A, Fukami H, Kaminishi M, et al. Induction of glandular stomach cancers in Helicobacter pylori-sensitive Mongolian gerbils treated with N-methyl-N-nitrosourea and N-methyl-N'-nitro-N-nitrosoguanidine in drinking water. Jpn J Cancer Res. 1998; 89:97–104.


16. Danon SJ, Eaton KA. The role of gastric Helicobacter and N-methyl-N'-nitro- N-nitrosoguanidine in carcinogenesis of mice. Helicobacter. 1998; 3:260–268.


17. Tatematsu M, Ogawa K, Hoshiya T, Shichino Y, Kato T, Imaida K, et al. Induction of adenocarcinomas in the glandular stomach of BALB/c mice treated with N-methyl-N-nitrosourea. Jpn J Cancer Res. 1992; 83:915–918.


18. Tatematsu M, Yamamoto M, Iwata H, Fukami H, Yuasa H, Tezuka N, et al. Induction of glandular stomach cancers in C3H mice treated with N-methyl-N-nitrosourea in the drinking water. Jpn J Cancer Res. 1993; 84:1258–1264.


19. Yamachika T, Nakanishi H, Inada K, Tsukamoto T, Shimizu N, Kobayashi K, et al. N-methyl-N-nitrosourea concentration-dependent, rather than total intake-dependent, induction of adenocarcinomas in the glandular stomach of BALB/c mice. Jpn J Cancer Res. 1998; 89:385–391.


20. Yamamoto M, Furihata C, Ogiu T, Tsukamoto T, Inada Ki, Hirano K, et al. Independent variation in susceptibilities of six different mouse strains to induction of pepsinogen-altered pyloric glands and gastric tumor intestinalization by N-methyl-N-nitrosourea. Cancer Lett. 2002; 179:121–132.


21. Hayakawa Y, Fox JG, Gonda T, Worthley DL, Muthupalani S, Wang TC. Mouse models of gastric cancer. Cancers (Basel). 2013; 5:92–130.


22. Yamamoto M, Tsukamoto T, Sakai H, Shirai N, Ohgaki H, Furihata C, et al. p53 knockout mice (-/-) are more susceptible than (+/-) or (+/+) mice to N-methyl-N-nitrosourea stomach carcinogenesis. Carcinogenesis. 2000; 21:1891–1897.


23. Sakamoto K, Hikiba Y, Nakagawa H, Hayakawa Y, Yanai A, Akanuma M, et al. Inhibitor of kappaB kinase beta regulates gastric carcinogenesis via interleukin-1alpha expression. Gastroenterology. 2010; 139:226–238.


24. Shibata W, Maeda S, Hikiba Y, Yanai A, Sakamoto K, Nakagawa H, et al. c-Jun NH2-terminal kinase 1 is a critical regulator for the development of gastric cancer in mice. Cancer Res. 2008; 68:5031–5039.


25. Hayakawa Y, Hirata Y, Nakagawa H, Sakamoto K, Hikiba Y, Kinoshita H, et al. Apoptosis signal-regulating kinase 1 and cyclin D1 compose a positive feedback loop contributing to tumor growth in gastric cancer. Proc Natl Acad Sci U S A. 2011; 108:780–785.


26. Takasu S, Tsukamoto T, Cao XY, Toyoda T, Hirata A, Ban H, et al. Roles of cyclooxygenase-2 and microsomal prostaglandin E synthase-1 expression and beta-catenin activation in gastric carcinogenesis in N-methyl-N-nitrosourea-treated K19-C2mE transgenic mice. Cancer Sci. 2008; 99:2356–2364.


27. Leung WK, Wu KC, Wong CY, Cheng AS, Ching AK, Chan AW, et al. Transgenic cyclooxygenase-2 expression and high salt enhanced susceptibility to chemical-induced gastric cancer development in mice. Carcinogenesis. 2008; 29:1648–1654.


28. Humar B, Blair V, Charlton A, More H, Martin I, Guilford P. E-cadherin deficiency initiates gastric signet-ring cell carcinoma in mice and man. Cancer Res. 2009; 69:2050–2056.


29. Li Q, Jia Z, Wang L, Kong X, Li Q, Guo K, et al. Disruption of Klf4 in villin-positive gastric progenitor cells promotes formation and progression of tumors of the antrum in mice. Gastroenterology. 2012; 142:531–542.


30. Boffa LC, Bolognesi C. Methylating agents: their target amino acids in nuclear proteins. Carcinogenesis. 1985; 6:1399–1401.


31. Tomita H, Takaishi S, Menheniott TR, Yang X, Shibata W, Jin G, et al. Inhibition of gastric carcinogenesis by the hormone gastrin is mediated by suppression of TFF1 epigenetic silencing. Gastroenterology. 2011; 140:879–891.


32. Cao X, Tsukamoto T, Nozaki K, Tanaka H, Shimizu N, Kaminishi M, et al. Earlier Helicobacter pylori infection increases the risk for the N-methyl-N-nitrosourea-induced stomach carcinogenesis in Mongolian gerbils. Jpn J Cancer Res. 2002; 93:1293–1298.


33. Maruta F, Sugiyama A, Ishida K, Ikeno T, Murakami M, Kawasaki S, et al. Timing of N-methyl-N-nitrosourea administration affects gastric carcinogenesis in Mongolian gerbils infected with Helicobacter pylori. Cancer Lett. 2000; 160:99–105.


34. Fox JG, Wishnok JS, Murphy JC, Tannenbaum SR, Correa P. MNNG-induced gastric carcinoma in ferrets infected with Helicobacter mustelae. Carcinogenesis. 1993; 14:1957–1961.


35. Fox JG. Gastric disease in ferrets: effects of Helicobacter mustelae, nitrosamines and reconstructive gastric surgery. Eur J Gastroenterol Hepatol. 1994; 6:Suppl 1. S57–S65.
36. Fox JG, Dangler CA, Sager W, Borkowski R, Gliatto JM. Helicobacter mustelae-associated gastric adenocarcinoma in ferrets (Mustela putorius furo). Vet Pathol. 1997; 34:225–229.


37. Fox JG, Correa P, Taylor NS, Lee A, Otto G, Murphy JC, et al. Helicobacter mustelae-associated gastritis in ferrets. An animal model of Helicobacter pylori gastritis in humans. Gastroenterology. 1990; 99:352–361.


38. Wirth HP, Beins MH, Yang M, Tham KT, Blaser MJ. Experimental infection of Mongolian gerbils with wild-type and mutant Helicobacter pylori strains. Infect Immun. 1998; 66:4856–4866.


39. Ogura K, Maeda S, Nakao M, Watanabe T, Tada M, Kyutoku T, et al. Virulence factors of Helicobacter pylori responsible for gastric diseases in Mongolian gerbil. J Exp Med. 2000; 192:1601–1610.


40. Israel DA, Salama N, Arnold CN, Moss SF, Ando T, Wirth HP, et al. Helicobacter pylori strain-specific differences in genetic content, identified by microarray, influence host inflammatory responses. J Clin Invest. 2001; 107:611–620.


41. Ehlers S, Warrelmann M, Hahn H. In search of an animal model for experimental Campylobacter pylori infection: administration of Campylobacter pylori to rodents. Zentralbl Bakteriol Mikrobiol Hyg A. 1988; 268:341–346.


42. Cantorna MT, Balish E. Inability of human clinical strains of Helicobacter pylori to colonize the alimentary tract of germfree rodents. Can J Microbiol. 1990; 36:237–241.


43. Lee A, Fox JG, Otto G, Murphy J. A small animal model of human Helicobacter pylori active chronic gastritis. Gastroenterology. 1990; 99:1315–1323.


44. Lee A, Chen M, Coltro N, O'Rourke J, Hazell S, Hu P, et al. Long term infection of the gastric mucosa with Helicobacter species does induce atrophic gastritis in an animal model of Helicobacter pylori infection. Zentralbl Bakteriol. 1993; 280:38–50.


45. Sakagami T, Dixon M, O'Rourke J, Howlett R, Alderuccio F, Vella J, et al. Atrophic gastric changes in both Helicobacter felis and Helicobacter pylori infected mice are host dependent and separate from antral gastritis. Gut. 1996; 39:639–648.


46. Fox JG, Sheppard BJ, Dangler CA, Whary MT, Ihrig M, Wang TC. Germ-line p53-targeted disruption inhibits helicobacter-induced premalignant lesions and invasive gastric carcinoma through down-regulation of Th1 proinflammatory responses. Cancer Res. 2002; 62:696–702.
47. Wang TC, Goldenring JR, Dangler C, Ito S, Mueller A, Jeon WK, et al. Mice lacking secretory phospholipase A2 show altered apoptosis and differentiation with Helicobacter felis infection. Gastroenterology. 1998; 114:675–689.


48. Stoicov C, Saffari R, Cai X, Hasyagar C, Houghton J. Molecular biology of gastric cancer: Helicobacter infection and gastric adenocarcinoma: bacterial and host factors responsible for altered growth signaling. Gene. 2004; 341:1–17.


49. Rogers AB, Taylor NS, Whary MT, Stefanich ED, Wang TC, Fox JG. Helicobacter pylori but not high salt induces gastric intraepithelial neoplasia in B6129 mice. Cancer Res. 2005; 65:10709–10715.


50. Cai X, Carlson J, Stoicov C, Li H, Wang TC, Houghton J. Helicobacter felis eradication restores normal architecture and inhibits gastric cancer progression in C57BL/6 mice. Gastroenterology. 2005; 128:1937–1952.


51. Lee CW, Rickman B, Rogers AB, Ge Z, Wang TC, Fox JG. Helicobacter pylori eradication prevents progression of gastric cancer in hypergastrinemic INS-GAS mice. Cancer Res. 2008; 68:3540–3548.


52. Sepulveda AR, Coelho LG. Helicobacter pylori and gastric malignancies. Helicobacter. 2002; 7:Suppl 1. 37–42.


53. Ley C, Mohar A, Guarner J, Herrera-Goepfert R, Figueroa LS, Halperin D, et al. Helicobacter pylori eradication and gastric preneoplastic conditions: a randomized, double-blind, placebo-controlled trial. Cancer Epidemiol Biomarkers Prev. 2004; 13:4–10.


54. Lee CW, Rickman B, Rogers AB, Muthupalani S, Takaishi S, Yang P, et al. Combination of sulindac and antimicrobial eradication of Helicobacter pylori prevents progression of gastric cancer in hypergastrinemic INS-GAS mice. Cancer Res. 2009; 69:8166–8174.


55. Lee A, O'Rourke J, De Ungria MC, Robertson B, Daskalopoulos G, Dixon MF. A standardized mouse model of Helicobacter pylori infection: introducing the Sydney strain. Gastroenterology. 1997; 112:1386–1397.


56. Wang X, Willén R, Svensson M, Ljungh A, Wadström T. Two-year follow-up of Helicobacter pylori infection in C57BL/6 and Balb/cA mice. APMIS. 2003; 111:514–522.


57. Fox JG, Wang TC, Rogers AB, Poutahidis T, Ge Z, Taylor N, et al. Host and microbial constituents influence Helicobacter pylori-induced cancer in a murine model of hypergastrinemia. Gastroenterology. 2003; 124:1879–1890.


58. Lee K, Hwang H, Nam KT. Immune response and the tumor microenvironment: how they communicate to regulate gastric cancer. Gut Liver. 2014; 8:131–139.


59. Higashi H, Tsutsumi R, Muto S, Sugiyama T, Azuma T, Asaka M, et al. SHP-2 tyrosine phosphatase as an intracellular target of Helicobacter pylori CagA protein. Science. 2002; 295:683–686.
60. Maeda S, Yoshida H, Ogura K, Mitsuno Y, Hirata Y, Yamaji Y, et al. H. pylori activates NF-kappaB through a signaling pathway involving IkappaB kinases, NF-kappaB-inducing kinase, TRAF2, and TRAF6 in gastric cancer cells. Gastroenterology. 2000; 119:97–108.
61. Mitsuno Y, Yoshida H, Maeda S, Ogura K, Hirata Y, Kawabe T, et al. Helicobacter pylori induced transactivation of SRE and AP-1 through the ERK signalling pathway in gastric cancer cells. Gut. 2001; 49:18–22.
62. Shimizu N, Kaminishi M, Tatematsu M, Tsuji E, Yoshikawa A, Yamaguchi H, et al. Helicobacter pylori promotes development of pepsinogen-altered pyloric glands, a preneoplastic lesion of glandular stomach of BALB/c mice pretreated with N-methyl-N-nitrosourea. Cancer Lett. 1998; 123:63–69.
63. Han SU, Kim YB, Joo HJ, Hahm KB, Lee WH, Cho YK, et al. Helicobacter pylori infection promotes gastric carcinogenesis in a mice model. J Gastroenterol Hepatol. 2002; 17:253–261.
64. Fox JG, Dangler CA, Taylor NS, King A, Koh TJ, Wang TC. High-salt diet induces gastric epithelial hyperplasia and parietal cell loss, and enhances Helicobacter pylori colonization in C57BL/6 mice. Cancer Res. 1999; 59:4823–4828.
65. Fox JG, Beck P, Dangler CA, Whary MT, Wang TC, Shi HN, et al. Concurrent enteric helminth infection modulates inflammation and gastric immune responses and reduces helicobacter-induced gastric atrophy. Nat Med. 2000; 6:536–542.
66. Nam KT, Hahm KB, Oh SY, Yeo M, Han SU, Ahn B, et al. The selective cyclooxygenase-2 inhibitor nimesulide prevents Helicobacter pylori-associated gastric cancer development in a mouse model. Clin Cancer Res. 2004; 10:8105–8113.
67. Nam KT, Oh SY, Ahn B, Kim YB, Jang DD, Yang KH, et al. Decreased Helicobacter pylori associated gastric carcinogenesis in mice lacking inducible nitric oxide synthase. Gut. 2004; 53:1250–1255.
68. Ito K, Chuang LS, Ito T, Chang TL, Fukamachi H, Salto-Tellez M, et al. Loss of Runx3 is a key event in inducing precancerous state of the stomach. Gastroenterology. 2011; 140:1536–1546.
69. Thompson J, Epting T, Schwarzkopf G, Singhofen A, Eades-Perner AM, van Der Putten H, et al. A transgenic mouse line that develops early-onset invasive gastric carcinoma provides a model for carcinoembryonic antigen-targeted tumor therapy. Int J Cancer. 2000; 86:863–869.
70. Koike K, Hinrichs SH, Isselbacher KJ, Jay G. Transgenic mouse model for human gastric carcinoma. Proc Natl Acad Sci U S A. 1989; 86:5615–5619.
71. Searle PF, Thomas DP, Faulkner KB, Tinsley JM. Stomach cancer in transgenic mice expressing human papillomavirus type 16 early region genes from a keratin promoter. J Gen Virol. 1994; 75:1125–1137.
72. Lefebvre O, Chenard MP, Masson R, Linares J, Dierich A, LeMeur M, et al. Gastric mucosa abnormalities and tumorigenesis in mice lacking the pS2 trefoil protein. Science. 1996; 274:259–262.
73. Tomasetto C, Rio MC. Pleiotropic effects of Trefoil factor 1 deficiency. Cell Mol Life Sci. 2005; 62:2916–2920.
74. Johnson AH, Frierson HF, Zaika A, Powell SM, Roche J, Crowe S, et al. Expression of tight-junction protein claudin-7 is an early event in gastric tumorigenesis. Am J Pathol. 2005; 167:577–584.
75. Tebbutt NC, Giraud AS, Inglese M, Jenkins B, Waring P, Clay FJ, et al. Reciprocal regulation of gastrointestinal homeostasis by SHP2 and STAT-mediated trefoil gene activation in gp130 mutant mice. Nat Med. 2002; 8:1089–1097.
76. Judd LM, Alderman BM, Howlett M, Shulkes A, Dow C, Moverley J, et al. Gastric cancer development in mice lacking the SHP2 binding site on the IL-6 family co-receptor gp130. Gastroenterology. 2004; 126:196–207.
77. Jenkins BJ, Grail D, Nheu T, Najdovska M, Wang B, Waring P, et al. Hyperactivation of Stat3 in gp130 mutant mice promotes gastric hyperproliferation and desensitizes TGF-beta signaling. Nat Med. 2005; 11:845–852.
78. Mutoh H, Hakamata Y, Sato K, Eda A, Yanaka I, Honda S, et al. Conversion of gastric mucosa to intestinal metaplasia in Cdx2-expressing transgenic mice. Biochem Biophys Res Commun. 2002; 294:470–479.
79. Mutoh H, Sakurai S, Satoh K, Osawa H, Hakamata Y, Takeuchi T, et al. Cdx1 induced intestinal metaplasia in the transgenic mouse stomach: comparative study with Cdx2 transgenic mice. Gut. 2004; 53:1416–1423.
80. Mutoh H, Sakurai S, Satoh K, Tamada K, Kita H, Osawa H, et al. Development of gastric carcinoma from intestinal metaplasia in Cdx2-transgenic mice. Cancer Res. 2004; 64:7740–7747.
81. Wang TC, Dangler CA, Chen D, Goldenring JR, Koh T, Raychowdhury R, et al. Synergistic interaction between hypergastrinemia and Helicobacter infection in a mouse model of gastric cancer. Gastroenterology. 2000; 118:36–47.
82. El-Zaatari M, Tobias A, Grabowska AM, Kumari R, Scotting PJ, Kaye P, et al. De-regulation of the sonic hedgehog pathway in the InsGas mouse model of gastric carcinogenesis. Br J Cancer. 2007; 96:1855–1861.
83. Konda Y, Kamimura H, Yokota H, Hayashi N, Sugano K, Takeuchi T. Gastrin stimulates the growth of gastric pit with less-differentiated features. Am J Physiol. 1999; 277:G773–G784.
84. Kanda N, Seno H, Kawada M, Sawabu T, Uenoyoma Y, Nakajima T, et al. Involvement of cyclooxygenase-2 in gastric mucosal hypertrophy in gastrin transgenic mice. Am J Physiol Gastrointest Liver Physiol. 2006; 290:G519–G527.
85. Friis-Hansen L, Sundler F, Li Y, Gillespie PJ, Saunders TL, Greenson JK, et al. Impaired gastric acid secretion in gastrin-deficient mice. Am J Physiol. 1998; 274:G561–G568.
86. Koh TJ, Goldenring JR, Ito S, Mashimo H, Kopin AS, Varro A, et al. Gastrin deficiency results in altered gastric differentiation and decreased colonic proliferation in mice. Gastroenterology. 1997; 113:1015–1025.
87. Zavros Y, Rieder G, Ferguson A, Samuelson LC, Merchant JL. Genetic or chemical hypochlorhydria is associated with inflammation that modulates parietal and G-cell populations in mice. Gastroenterology. 2002; 122:119–133.
88. Zavros Y, Eaton KA, Kang W, Rathinavelu S, Katukuri V, Kao JY, et al. Chronic gastritis in the hypochlorhydric gastrin-deficient mouse progresses to adenocarcinoma. Oncogene. 2005; 24:2354–2366.
89. Goldenring JR, Nomura S. Differentiation of the gastric mucosa III. Animal models of oxyntic atrophy and metaplasia. Am J Physiol Gastrointest Liver Physiol. 2006; 291:G999–G1004.
90. Kang W, Rathinavelu S, Samuelson LC, Merchant JL. Interferon gamma induction of gastric mucous neck cell hypertrophy. Lab Invest. 2005; 85:702–715.
91. Judd LM, Andringa A, Rubio CA, Spicer Z, Shull GE, Miller ML. Gastric achlorhydria in H/K-ATPase-deficient (Atp4a(-/-)) mice causes severe hyperplasia, mucocystic metaplasia and upregulation of growth factors. J Gastroenterol Hepatol. 2005; 20:1266–1278.
92. Schultheis PJ, Clarke LL, Meneton P, Harline M, Boivin GP, Stemmermann G, et al. Targeted disruption of the murine Na+/H+ exchanger isoform 2 gene causes reduced viability of gastric parietal cells and loss of net acid secretion. J Clin Invest. 1998; 101:1243–1253.
93. Gawenis LR, Greeb JM, Prasad V, Grisham C, Sanford LP, Doetschman T, et al. Impaired gastric acid secretion in mice with a targeted disruption of the NHE4 Na+/H+ exchanger. J Biol Chem. 2005; 280:12781–12789.
94. Lee MP, Ravenel JD, Hu RJ, Lustig LR, Tomaselli G, Berger RD, et al. Targeted disruption of the Kvlqt1 gene causes deafness and gastric hyperplasia in mice. J Clin Invest. 2000; 106:1447–1455.
95. Elso CM, Lu X, Culiat CT, Rutledge JC, Cacheiro NL, Generoso WM, et al. Heightened susceptibility to chronic gastritis, hyperplasia and metaplasia in Kcnq1 mutant mice. Hum Mol Genet. 2004; 13:2813–2821.
96. Kobayashi T, Tonai S, Ishihara Y, Koga R, Okabe S, Watanabe T. Abnormal functional and morphological regulation of the gastric mucosa in histamine H2 receptor-deficient mice. J Clin Invest. 2000; 105:1741–1749.
97. Ogawa T, Maeda K, Tonai S, Kobayashi T, Watanabe T, Okabe S. Utilization of knockout mice to examine the potential role of gastric histamine H2-receptors in Menetrier's disease. J Pharmacol Sci. 2003; 91:61–70.
98. Li S, Wang Q, Chakladar A, Bronson RT, Bernards A. Gastric hyperplasia in mice lacking the putative Cdc42 effector IQGAP1. Mol Cell Biol. 2000; 20:697–701.
99. Crawford SE, Stellmach V, Murphy-Ullrich JE, Ribeiro SM, Lawler J, Hynes RO, et al. Thrombospondin-1 is a major activator of TGF-beta1 in vivo. Cell. 1998; 93:1159–1170.
100. Shull MM, Ormsby I, Kier AB, Pawlowski S, Diebold RJ, Yin M, et al. Targeted disruption of the mouse transforming growth factor-beta 1 gene results in multifocal inflammatory disease. Nature. 1992; 359:693–699.
101. Kim BG, Li C, Qiao W, Mamura M, Kasprzak B, Anver M, et al. Smad4 signalling in T cells is required for suppression of gastrointestinal cancer. Nature. 2006; 441:1015–1019.
102. Redman RS, Katuri V, Tang Y, Dillner A, Mishra B, Mishra L. Orofacial and gastrointestinal hyperplasia and neoplasia in smad4+/- and elf+/-/smad4+/- mutant mice. J Oral Pathol Med. 2005; 34:23–29.
103. Li QL, Ito K, Sakakura C, Fukamachi H, Inoue Ki, Chi XZ, et al. Causal relationship between the loss of RUNX3 expression and gastric cancer. Cell. 2002; 109:113–124.
104. Levanon D, Bettoun D, Harris-Cerruti C, Woolf E, Negreanu V, Eilam R, et al. The Runx3 transcription factor regulates development and survival of TrkC dorsal root ganglia neurons. EMBO J. 2002; 21:3454–3463.
105. Brenner O, Levanon D, Negreanu V, Golubkov O, Fainaru O, Woolf E, et al. Loss of Runx3 function in leukocytes is associated with spontaneously developed colitis and gastric mucosal hyperplasia. Proc Natl Acad Sci U S A. 2004; 101:16016–16021.
106. Harada N, Tamai Y, Ishikawa T, Sauer B, Takaku K, Oshima M, et al. Intestinal polyposis in mice with a dominant stable mutation of the beta-catenin gene. EMBO J. 1999; 18:5931–5942.
107. Romagnolo B, Berrebi D, Saadi-Keddoucci S, Porteu A, Pichard AL, Peuchmaur M, et al. Intestinal dysplasia and adenoma in transgenic mice after overexpression of an activated beta-catenin. Cancer Res. 1999; 59:3875–3879.
108. Yang K, Edelmann W, Fan K, Lau K, Kolli VR, Fodde R, et al. A mouse model of human familial adenomatous polyposis. J Exp Zool. 1997; 277:245–254.
109. Fox JG, Dangler CA, Whary MT, Edelman W, Kucherlapati R, Wang TC. Mice carrying a truncated Apc gene have diminished gastric epithelial proliferation, gastric inflammation, and humoral immunity in response to Helicobacter felis infection. Cancer Res. 1997; 57:3972–3978.
110. Tomita H, Yamada Y, Oyama T, Hata K, Hirose Y, Hara A, et al. Development of gastric tumors in Apc(Min/+) mice by the activation of the beta-catenin/Tcf signaling pathway. Cancer Res. 2007; 67:4079–4087.
111. Tsuzuki T, Egashira A, Igarashi H, Iwakuma T, Nakatsuru Y, Tominaga Y, et al. Spontaneous tumorigenesis in mice defective in the MTH1 gene encoding 8-oxo-dGTPase. Proc Natl Acad Sci U S A. 2001; 98:11456–11461.
112. Cai JP, Ishibashi T, Takagi Y, Hayakawa H, Sekiguchi M. Mouse MTH2 protein which prevents mutations caused by 8-oxoguanine nucleotides. Biochem Biophys Res Commun. 2003; 305:1073–1077.
113. Oshima H, Oshima M, Inaba K, Taketo MM. Hyperplastic gastric tumors induced by activated macrophages in COX-2/mPGES-1 transgenic mice. EMBO J. 2004; 23:1669–1678.
114. Oshima M, Oshima H, Matsunaga A, Taketo MM. Hyperplastic gastric tumors with spasmolytic polypeptide-expressing metaplasia caused by tumor necrosis factor-alpha-dependent inflammation in cyclooxygenase-2/microsomal prostaglandin E synthase-1 transgenic mice. Cancer Res. 2005; 65:9147–9151.
115. Oshima H, Matsunaga A, Fujimura T, Tsukamoto T, Taketo MM, Oshima M. Carcinogenesis in mouse stomach by simultaneous activation of the Wnt signaling and prostaglandin E2 pathway. Gastroenterology. 2006; 131:1086–1095.
116. Lawler J, Sunday M, Thibert V, Duquette M, George EL, Rayburn H, et al. Thrombospondin-1 is required for normal murine pulmonary homeostasis and its absence causes pneumonia. J Clin Invest. 1998; 101:982–992.
117. Ludlow A, Yee KO, Lipman R, Bronson R, Weinreb P, Huang X, et al. Characterization of integrin beta6 and thrombospondin-1 double-null mice. J Cell Mol Med. 2005; 9:421–437.
118. Dempsey PJ, Goldenring JR, Soroka CJ, Modlin IM, McClure RW, Lind CD, et al. Possible role of transforming growth factor alpha in the pathogenesis of Ménétrier's disease: supportive evidence form humans and transgenic mice. Gastroenterology. 1992; 103:1950–1963.
119. Takagi H, Jhappan C, Sharp R, Merlino G. Hypertrophic gastropathy resembling Ménétrier's disease in transgenic mice overexpressing transforming growth factor alpha in the stomach. J Clin Invest. 1992; 90:1161–1167.
120. Bockman DE, Sharp R, Merlino G. Regulation of terminal differentiation of zymogenic cells by transforming growth factor alpha in transgenic mice. Gastroenterology. 1995; 108:447–454.
121. Goldenring JR, Ray GS, Soroka CJ, Smith J, Modlin IM, Meise KS, et al. Overexpression of transforming growth factor-alpha alters differentiation of gastric cell lineages. Dig Dis Sci. 1996; 41:773–784.
122. Sharp R, Babyatsky MW, Takagi H, Tågerud S, Wang TC, Bockman DE, et al. Transforming growth factor alpha disrupts the normal program of cellular differentiation in the gastric mucosa of transgenic mice. Development. 1995; 121:149–161.
123. Takagi H, Fukusato T, Kawaharada U, Kuboyama S, Merlino G, Tsutsumi Y. Histochemical analysis of hyperplastic stomach of TGF-alpha transgenic mice. Dig Dis Sci. 1997; 42:91–98.
124. Nomura S, Settle SH, Leys CM, Means AL, Peek RM Jr, Leach SD, et al. Evidence for repatterning of the gastric fundic epithelium associated with Ménétrier's disease and TGFalpha overexpression. Gastroenterology. 2005; 128:1292–1305.
125. Andersson P, McGuire J, Rubio C, Gradin K, Whitelaw ML, Pettersson S, et al. A constitutively active dioxin/aryl hydrocarbon receptor induces stomach tumors. Proc Natl Acad Sci U S A. 2002; 99:9990–9995.
126. Kuznetsov NV, Andersson P, Gradin K, Stein PV, Dieckmann A, Pettersson S, et al. The dioxin/aryl hydrocarbon receptor mediates downregulation of osteopontin gene expression in a mouse model of gastric tumourigenesis. Oncogene. 2005; 24:3216–3222.
127. Segre JA, Bauer C, Fuchs E. Klf4 is a transcription factor required for establishing the barrier function of the skin. Nat Genet. 1999; 22:356–360.
128. Katz JP, Perreault N, Goldstein BG, Actman L, McNally SR, Silberg DG, et al. Loss of Klf4 in mice causes altered proliferation and differentiation and precancerous changes in the adult stomach. Gastroenterology. 2005; 128:935–945.
129. Kuzushita N, Rogers AB, Monti NA, Whary MT, Park MJ, Aswad BI, et al. p27kip1 deficiency confers susceptibility to gastric carcinogenesis in Helicobacter pylori-infected mice. Gastroenterology. 2005; 129:1544–1556.
130. Fukui T, Nishio A, Okazaki K, Uza N, Ueno S, Kido M, et al. Gastric mucosal hyperplasia via upregulation of gastrin induced by persistent activation of gastric innate immunity in major histocompatibility complex class II deficient mice. Gut. 2006; 55:607–615.
131. Gut MO, Parkkila S, Vernerová Z, Rohde E, Závada J, Höcker M, et al. Gastric hyperplasia in mice with targeted disruption of the carbonic anhydrase gene Car9. Gastroenterology. 2002; 123:1889–1903.
132. Leppilampi M, Karttunen TJ, Kivelä J, Gut MO, Pastoreková S, Pastorek J, et al. Gastric pit cell hyperplasia and glandular atrophy in carbonic anhydrase IX knockout mice: studies on two strains C57/BL6 and BALB/C. Transgenic Res. 2005; 14:655–663.
133. Nöckel J, van den Engel NK, Winter H, Hatz RA, Zimmermann W, Kammerer R. Characterization of gastric adenocarcinoma cell lines established from CEA424/SV40 T antigen-transgenic mice with or without a human CEA transgene. BMC Cancer. 2006; 6:57.
134. Li Q, Karam SM, Gordon JI. Simian virus 40 T antigen-induced amplification of pre-parietal cells in transgenic mice. Effects on other gastric epithelial cell lineages and evidence for a p53-independent apoptotic mechanism that operates in a committed progenitor. J Biol Chem. 1995; 270:15777–15788.
135. Syder AJ, Karam SM, Mills JC, Ippolito JE, Ansari HR, Farook V, et al. A transgenic mouse model of metastatic carcinoma involving transdifferentiation of a gastric epithelial lineage progenitor to a neuroendocrine phenotype. Proc Natl Acad Sci U S A. 2004; 101:4471–4476.
136. Stewart LA, van Driel IR, Gleeson PA. Perturbation of gastric mucosa in mice expressing the temperature-sensitive mutant of SV40 large T antigen. Potential for establishment of an immortalised parietal cell line. Eur J Cell Biol. 2002; 81:281–293.
137. Kaestner KH, Silberg DG, Traber PG, Schütz G. The mesenchymal winged helix transcription factor Fkh6 is required for the control of gastrointestinal proliferation and differentiation. Genes Dev. 1997; 11:1583–1595.
138. Ramalho-Santos M, Melton DA, McMahon AP. Hedgehog signals regulate multiple aspects of gastrointestinal development. Development. 2000; 127:2763–2772.
139. Saitou M, Furuse M, Sasaki H, Schulzke JD, Fromm M, Takano H, et al. Complex phenotype of mice lacking occludin, a component of tight junction strands. Mol Biol Cell. 2000; 11:4131–4142.
140. Höpken UE, Wengner AM, Loddenkemper C, Stein H, Heimesaat MM, Rehm A, et al. CCR7 deficiency causes ectopic lymphoid neogenesis and disturbed mucosal tissue integrity. Blood. 2007; 109:886–895.
141. Ishikawa H, Carrasco D, Claudio E, Ryseck RP, Bravo R. Gastric hyperplasia and increased proliferative responses of lymphocytes in mice lacking the COOH-terminal ankyrin domain of NF-kappaB2. J Exp Med. 1997; 186:999–1014.
142. Friis-Hansen L. Gastric functions in gastrin gene knock-out mice. Pharmacol Toxicol. 2002; 91:363–367.
143. Watson SA, Grabowska AM, El-Zaatari M, Takhar A. Gastrin-active participant or bystander in gastric carcinogenesis? Nat Rev Cancer. 2006; 6:936–946.
144. Wang TC, Bonner-Weir S, Oates PS, Chulak M, Simon B, Merlino GT, et al. Pancreatic gastrin stimulates islet differentiation of transforming growth factor alpha-induced ductular precursor cells. J Clin Invest. 1993; 92:1349–1356.
145. Wang TC, Koh TJ, Varro A, Cahill RJ, Dangler CA, Fox JG, et al. Processing and proliferative effects of human progastrin in transgenic mice. J Clin Invest. 1996; 98:1918–1929.
146. Takaishi S, Cui G, Frederick DM, Carlson JE, Houghton J, Varro A, et al. Synergistic inhibitory effects of gastrin and histamine receptor antagonists on Helicobacter-induced gastric cancer. Gastroenterology. 2005; 128:1965–1983.
147. Akhurst B, Croager EJ, Farley-Roche CA, Ong JK, Dumble ML, Knight B, et al. A modified choline-deficient, ethionine-supplemented diet protocol effectively induces oval cells in mouse liver. Hepatology. 2001; 34:519–522.
148. Derynck R, Akhurst RJ, Balmain A. TGF-beta signaling in tumor suppression and cancer progression. Nat Genet. 2001; 29:117–129.
149. Hahm KB, Lee KM, Kim YB, Hong WS, Lee WH, Han SU, et al. Conditional loss of TGF-beta signalling leads to increased susceptibility to gastrointestinal carcinogenesis in mice. Aliment Pharmacol Ther. 2002; 16:Suppl 2. 115–127.
150. Fox JG, Li X, Cahill RJ, Andrutis K, Rustgi AK, Odze R, et al. Hypertrophic gastropathy in Helicobacter felis-infected wild-type C57BL/6 mice and p53 hemizygous transgenic mice. Gastroenterology. 1996; 110:155–166.
151. Li Q, Karam SM, Gordon JI. Diphtheria toxin-mediated ablation of parietal cells in the stomach of transgenic mice. J Biol Chem. 1996; 271:3671–3676.
152. Canfield V, West AB, Goldenring JR, Levenson R. Genetic ablation of parietal cells in transgenic mice: a new model for analyzing cell lineage relationships in the gastric mucosa. Proc Natl Acad Sci U S A. 1996; 93:2431–2435.
153. Spicer Z, Miller ML, Andringa A, Riddle TM, Duffy JJ, Doetschman T, et al. Stomachs of mice lacking the gastric H,K-ATPase alpha -subunit have achlorhydria, abnormal parietal cells, and ciliated metaplasia. J Biol Chem. 2000; 275:21555–21565.
154. Scarff KL, Judd LM, Toh BH, Gleeson PA, Van Driel IR. Gastric H(+),K(+)-adenosine triphosphatase beta subunit is required for normal function, development, and membrane structure of mouse parietal cells. Gastroenterology. 1999; 117:605–618.
155. Franic TV, Judd LM, Robinson D, Barrett SP, Scarff KL, Gleeson PA, et al. Regulation of gastric epithelial cell development revealed in H(+)/K(+)-ATPase beta-subunit- and gastrin-deficient mice. Am J Physiol Gastrointest Liver Physiol. 2001; 281:G1502–G1511.
156. Nagata A, Ito M, Iwata N, Kuno J, Takano H, Minowa O, et al. G protein-coupled cholecystokinin-B/gastrin receptors are responsible for physiological cell growth of the stomach mucosa in vivo. Proc Natl Acad Sci U S A. 1996; 93:11825–11830.
157. Langhans N, Rindi G, Chiu M, Rehfeld JF, Ardman B, Beinborn M, et al. Abnormal gastric histology and decreased acid production in cholecystokinin-B/gastrin receptor-deficient mice. Gastroenterology. 1997; 112:280–286.
158. Xiao C, Ogle SA, Schumacher MA, Orr-Asman MA, Miller ML, Lertkowit N, et al. Loss of parietal cell expression of Sonic hedgehog induces hypergastrinemia and hyperproliferation of surface mucous cells. Gastroenterology. 2010; 138:550–561.e1-8.
159. Goldenring JR, Ray GS, Coffey RJ, Meunier PC, Haley PJ, Barnes TB, et al. Reversible drug-induced oxyntic atrophy in rats. Gastroenterology. 2000; 118:1080–1093.
160. Nam KT, Lee HJ, Sousa JF, Weis VG, O'Neal RL, Finke PE, et al. Mature chief cells are cryptic progenitors for metaplasia in the stomach. Gastroenterology. 2010; 139:2028–2037.e9.
161. Silberg DG, Sullivan J, Kang E, Swain GP, Moffett J, Sund NJ, et al. Cdx2 ectopic expression induces gastric intestinal metaplasia in transgenic mice. Gastroenterology. 2002; 122:689–696.
162. Mutoh H, Hakamata Y, Sato K, Eda A, Yanaka I, Honda S, et al. Conversion of gastric mucosa to intestinal metaplasia in Cdx2-expressing transgenic mice. Biochem Biophys Res Commun. 2002; 294:470–479.
163. Quante M, Marrache F, Goldenring JR, Wang TC. TFF2 mRNA transcript expression marks a gland progenitor cell of the gastric oxyntic mucosa. Gastroenterology. 2010; 139:2018–2027.e2.
164. Kim TH, Shivdasani RA. Notch signaling in stomach epithelial stem cell homeostasis. J Exp Med. 2011; 208:677–688.
165. Barker N, Huch M, Kujala P, van de Wetering M, Snippert HJ, van Es JH, et al. Lgr5(+ve) stem cells drive self-renewal in the stomach and build long-lived gastric units in vitro. Cell Stem Cell. 2010; 6:25–36.
166. Arnold K, Sarkar A, Yram MA, Polo JM, Bronson R, Sengupta S, et al. Sox2(+) adult stem and progenitor cells are important for tissue regeneration and survival of mice. Cell Stem Cell. 2011; 9:317–329.
167. Qiao XT, Ziel JW, McKimpson W, Madison BB, Todisco A, Merchant JL, et al. Prospective identification of a multilineage progenitor in murine stomach epithelium. Gastroenterology. 2007; 133:1989–1998.
168. Johnson LR, Ghishan FK, Kaunitz JD, Merchant JL, Said HM, Wood JD, editors. Physiology of the Gastrointestinal Tract. 5th ed. London: Academic Press;2012.