Abstract
Purpose
Eupatilin is an antioxidative flavone and a phytopharmaceutical derived from Artemisia asiatica. It has been reported to possess anti-tumor activity in some types of cancer including gastric cancer. Eupatilin may modulate the angiogenesis pathway which is part of anti-inflammatory effect demonstrated in gastric mucosal injury models. Here we investigated the anti-tumor effects of eupatilin on gastric cancer cells and elucidated the potential underlying mechanism whereby eupatilin suppresses angiogenesis and tumor growth.
Materials and Methods
The impact of eupatilin on the expression of angiogenesis pathway proteins was assessed using western blots in MKN45 cells. Using a chromatin immunoprecipitation assay, we tested whether eupatilin affects the recruitment of signal transducer and activator of transcription 3 (STAT3), aryl hydrocarbon receptor nuclear translocator (ARNT) and hypoxia-inducible factor-1α (HIF-1α) to the human VEGF promoter. To investigate the effect of eupatilin on vasculogenesis, tube formation assays were conducted using human umbilical vein endothelial cells (HUVECs). The effect of eupatilin on tumor suppression in mouse xenografts was assessed.
Results
Eupatilin significantly reduced VEGF, ARNT and STAT3 expression prominently under hypoxic conditions. The recruitment of STAT3, ARNT and HIF-1α to the VEGF promoter was inhibited by eupatilin treatment. HUVECs produced much foreshortened and severely broken tubes with eupatilin treatment. In addition, eupatilin effectively reduced tumor growth in a mouse xenograft model.
It has been well demonstrated that neovascularization, or angiogenesis, is required for successful tumor growth and metastasis.(1) In addition vascular endothelial growth factor (VEGF) is known to be one of the most important and well characterized inducers of angiogenesis.(2-4)
VEGF expression and angiogenesis could be induced as a consequence of microenvironmental alterations, particularly hypoxia,(4) or genetic aberrations,(5,6) and including the activation of oncogenic kinases.(7,8)
Hypoxia-inducible factor (HIF) is a transcription factor that is stabilized under reduced oxygen tension and plays a key role in the cellular response to hypoxia. HIF is a heterodimer consisting of two subunits, oxygen-sensitive HIF-α and constitutively expressed HIF-β [also known as aryl hydrocarbon receptor nuclear translocator (ARNT), the heterodimeric partner of aryl hydrocarbon receptor (AHR)].(9) Upon hypoxia, HIF-1α heterodimerizes with the constitutively expressed HIF-1β subunit, and together they bind to DNA to increase the transcription of target genes including VEGF, erythropoietin, transferrin, endothelin 1, inducible nitric oxide synthase, and insulin-like growth factor II.(10-12)
Constitutive activation of protein kinases is highly prevalent in a wide range of cancers and their role in VEGF induction and angiogenesis has been well documented.(7,8) Although diverse kinases transduce signals through multiple routes, signal transducers and activators of transcription 3 (STAT3) comprises a convergence point of many signaling pathways(13,14) and transmits signals to the nucleus, where it binds to specific DNA promoter sequences and thereby regulate gene expression.(15) STAT proteins participate in tumorigenesis through up-regulation of genes encoding apoptosis inhibitors (myeloid cell leukemia sequence 1 (MCL1), BCL2-like 1 (BCL2L1)) and cell-cycle regulators (cyclin D1/D2, MYC). (16) STAT3 is also involved in tumor progression through inducing angiogenic factors, such as VEGF.(17) Aberrant activation of STAT3 is commonly observed in tumors and is strongly associated with tumor development and progression.
While searching for an antiangiogenic agent that would inhibit HIF-1 activity, we identified a novel pharmacologic activity of eupatilin. Eupatilin, a phytopharmaceutical derived from Artemisia asiatica, has been reported to possess antioxidative and cytoprotective functions in various models of gastric mucosal damage.(18-20)
We found that eupatilin inhibits HIF-1 activity in vitro. Eupatilin completely blocks HIF-1α expression at the post-transcriptional level and consequently inhibits the transcription factor activity of HIF-1 in cancer cells cultured under hypoxic conditions.
In this study, we demonstrated that eupatilin inhibits STAT3 activation in hypoxia-stimulated cancer cells. Further, the transcriptional activation of the VEGF promoter was mediated by active STAT3. Furthermore, active STAT3 interacted with HIF-1 and increased HIF-1 accumulation in the hypoxic cells.
The human gastric cancer cell line MKN45 was maintained in Dulbecco's modified Eagle's medium (DMEM) supplemented with 10% heat-inactivated fetal bovine serum (FBS) and 1% antibiotics. Human umbilical vein endothelial cells (HUVECs) were obtained from Angiolab Corp. (Daejeon, Korea) and grown in M199 medium (GIBCO BRL, Gaithersburg, MD, USA) supplemented with 10% FBS, 50 µg/ml endothelial cell growth supplement (Sigma, St louis, MO, USA), heparin, and antibiotics. HUVECs were used between passages 5 and 8. Both MKN45 and HUVEC cells were cultured at 37℃ in a humidified atmosphere containing 5% CO2. For hypoxic conditions, cells were incubated at 5% CO2 levels with 1% O2 balanced with N2 in a hypoxic GasPak (BD bioscience, San Jose, CA, USA; 260683).
Eupatilin was generously provided by Dong-A Pharmaceutical Co. Ltd. (Yongin, Korea), dissolved in dimethysulfoxide (DMSO) as a 10 mM stock solution, stored at -20℃, and then diluted into Hanks' balanced salt solution (HBSS; GIBCO, Rockville, MD, USA) for use in experiments. Cells were treated with various concentrations of eupatilin (0~150 mM) or with DMSO vehicle. Otherwise, cells were preincubated with 100 mM of eupatilin or under hypoxic conditions for 5~6 hr.
After treatment with eupatilin, cells were washed in PBS, and cell lysates were prepared by adding 1 ml of RIPA modified buffer (50 mM Tris-Cl, pH 7.5, 150 mM NaCl, 1% Nonidet P-40, 1 mM EDTA, 1 mM PMSF) supplemented with protease inhibitors (Roche Molecular Biochemicals, Mannheim, Germany). Agarose beads precleared with protein A/G (Santa Cruz Biotechnology, Santa Cruz, CA, USA) were then incubated with lysates at 4℃ for 1 hr and a 1 : 200 dilution of anti-HIF-1α monoclonal antibody (BD Biosciences, San Jose, CA, USA) was added. After incubation at 4℃ for 2 hr, the beads were washed one time with RIPA buffer and twice with PBS, and the immune complexes were released from the beads by boiling in sample buffer for 5 min. Following electrophoresis on 10% SDS-polyacrylamide gels, immunoprecipitates were analyzed by Western blotting using rabbit anti-STAT3 polyclonal antibody (Cell Signaling Technology, Danvers, MA, USA) (1 : 1,000), rabbit anti-ARNT polyclonal antibody (Santa Cruz Biotechnology, Santa Crue, CA, USA; sc-5580) (1 : 1,000), and rabbit anti-VEGF polyclonal antibody (Santa Cruz Biotechnology, CA, USA; sc-507) (1 : 500).
Cells were treated with eupatilin for the indicated times followed by treatment with the cross-linking reagent formaldehyde (1% final concentration) for 10 min at 37℃. They were then rinsed twice with cold PBS, and swollen on ice in SDS lysis buffer (1% SDS, 10 mM EDTA, 50 mM Tris-HCl, pH 8.1) for 10 min. Nuclei were collected and sonicated on ice. Supernatants were obtained by centrifugation for 10 min and diluted 10-fold in chromatin immunoprecipitation (ChIP) dilution buffer (0.01% SDS, 1.1% Triton X-100, 1.2 mM EDTA, 16.7 mM Tris-HCl, pH 8.1, 167 mM NaCl). The mixture (fragmented chromatin) was then incubated with 2 ml of anti-HIF-1α, anti-STAT3, or anti-ARNT antibody on a rotator at 4℃ for 4 hr. Protein A/G plus-agarose beads (20 ml) were added followed by incubation for 1 hr at 4℃ with rotation to collect the antibody/chromatin complexes. Cross-linked, precipitated chromatin complexes were recovered and cross-links were reversed according to Upstate's protocol (Upstate, Chicago, IL, USA). Final DNA pellets were recovered and analyzed by PCR using a pair of primers that encompass the VEGF promoter region (235 bp). The primers used were forward 5'-AGACTCCACAGTGCATACGTG-3' and reverse 5'-AGTGTGTCCCTCTGACAATG-3'.
Growth factor-reduced Matrigel (BD Biosciences, San Jose) was placed in the well of a pre-chilled 24-well cell culture plate and incubated at 37℃ for 1 hr to allow polymerization. HUVECs, at concentrations of 4×104 per well, were plated into the growth factor-reduced Matrigel coated wells, and incubated at 37℃ in 5% CO2 in the conditioned media. After 16-h incubation, the plates were photographed. Tube formation was quantified by counting the number of connected cells in five randomly selected fields at ×200 magnification. All experiments were performed in triplicates. Statistical significance was determined using independent sample two-sided Student t-test at the 5% level of significance using SPSS for Windows (version 12.0, SPSS Inc., Chicago, IL, USA).
Animal experiments were carried out in accordance with the policies of the animal research committee of the Yonsei University College of Medicine. Tumor models were generated by direct subcutaneous injection of 1×106 MKN45 cells into 8-week-old female nude mice. When tumors were approximately 50 mm3 in size (7 days), the animals were randomly divided into two groups (five mice per group) for no treatment (vehicle only) and treatment with eupatilin (EPT), respectively. EPT (10 mg/kg) in 200 µl HBSS or 200 µl of HBSS only were administered three times a week for 2 weeks by intraperitoneal injection. The total amount of EPT injected to the mice in the treatment group was 60 mg/kg for each mouse.
Mice were weighed and tumor measurements were taken in three coordinates using digital calipers two to three times weekly. Tumor measurements were converted to tumor volume using the formula L×S2/2 (where L = ongest diameter; S = shortest diameter). Mice were sacrificed when the longest diameter of all tumors exceeded 15 mm in the control group. At the time of sacrifice, mice were weighed, and tumors excised and ex vivo tumor weight was measured. Statistical significance was determined using Student's t-test.
To investigate the effects of eupatilin on the expression of STAT3 and VEGF in hypoxic condition, we examined HIF-1α, ARNT, STAT3, p-STAT3 and VEGF by Western blot. Eupatilin strongly inhibited the expression of HIF-1α which is stimulated by hypoxia (Fig. 1). We found that total STAT3 expression was increased by hypoxic conditions. Interestingly, active STAT3, the phosphorylated form, was more significantly reduced with eupatilin treatment in hypoxia than in normoxia. As shown in Fig. 1, eupatilin coordinately inhibited the expression of HIF-1α, phosphorylated STAT3 and VEGF at a level of 100 µM in MKN45 cells cultured in hypoxia. These data indicate that eupatilin was capable of down modulating the expression of proteins in angiogenesis pathways in gastric cancer cells under hypoxic conditions.
Our data showed that STAT3 protein expression was greater in hypoxic conditions compared to normoxia, suggesting the important role of STAT3 in regulating VEGF expression in hypoxia (Fig. 1). We thus hypothesized that VEGF expression is cooperatively regulated by HIF-1α and ARNT, as well as by STAT3. To test this hypothesis, we first investigated possible interaction between HIF-1α, STAT3, ARNT and VEGF using the co-immunoprecipitation assays. MKN45 cells grown under either normoxic or hypoxic conditions were lysed and immunoprecipitated with an anti-HIF-1α antibody, followed by Western blotting with anti-STAT3, ARNT, or VEGF antibodies. We found that STAT3 and VEGF were co-precipitated with HIF-1α in normoxic or hypoxic cells (Fig. 2A). Interestingly, for reasons yet to be explained, ARNT was co-precipitated with HIF-1α only in hypoxic conditions.
To investigate whether STAT3, HIF-1α, and ARNT might be recruited to the VEGF promoter and whether eupatilin may be inhibiting the interaction between the angiogenic proteins and the VEGF promoter, we performed ChIP assays on chromatin samples from normoxic and hypoxic cells with eupatilin treatment. As expected, a slight increased in the interaction of HIF-1α with the VEGF promoter was observed for hypoxia (Fig. 2B).
In light of the role of eupatilin in suppressing the angiogenic pathway as suggested above, we next investigated the effect of eupatilin on vascular endothelial cells under hypoxic conditions. In vitro angiogenesis assays were conducted using HUVECs. During angiogenesis, endothelial cells must break and traverse through their basement membrane to form new blood vessels. Hypoxia can stimulate endothelial cell invasion and tube formation. Eupatilin was administered to HUVECs seeded on Matrigel beds (10 mg/ml) and incubated for 16 hr under hypoxic conditions. Eupatilin strongly inhibited the hypoxia-stimulated capillary network formation. With increasing doses of eupatilin, vasculogenesis was significantly inhibited as evidenced by the production of considerably foreshortened and severely broken tubes (Fig. 3).
Based on the observed in vitro effects of eupatilin on angiogenesis, we investigated whether eupatilin might inhibit tumor growth in vivo. Tumor models were generated by direct, subcutaneous injection of 1×106 MKN45 cells resuspended in 200 µl conditioned medium into 8-week-old female nude mice. After a tumor was established, mice were randomly divided into two groups (five mice per group) for no treatment (vehicle only) and treatment with eupatilin (EPT), respectively. EPT (10 mg/kg) in 200 ml HBSS or 200 µl of HBSS only were administered three times a week by intraperitoneal injection.
Tumors in eupatilin-treated mice were significantly smaller than those in vehicle-treated mice (Fig. 4A). The changes in tumor size was measured and plotted as average tumor size versus time (data not shown). When ex vivo tumor weight was measured upon sacrifice, there was a significant difference in tumor weight between the control (vehicle only) and EPT groups (Fig 4B). These results indicated that eupatilin effectively inhibited tumor growth in a xenograft tumor model.
Angiogenesis is essential for the growth and metastasis of solid tumors, and the inhibition of angiogenesis is emerging as a promising strategy for cancer treatment.(21) VEGF is one of the most important and well characterized inducers of angiogenesis.(2-4)
HIF-1α is stabilized in hypoxic conditions and is a transcriptional regulator of VEGF synthesis.(22) Previous studies have demonstrated that STAT3 is a direct transcriptional activator of the VEGF gene, and that it forms a transcriptional complex with HIF-1α.(23) Many studies have concentrated on STAT3 as a potential target for cancer therapy and have found that STAT3 inhibition effectively blocks production of VEGF and tumor angiogenesis. (24) In this study, we observed that eupatilin down-regulated the protein levels of HIF-1α and STAT3, which are upregulated in hypoxia in MKN 45 cells. Of note, for reasons yet to be determined, expression of phosphorylated STAT3 and ARNT was only significantly reduced by eupatilin under hypoxic conditions (Fig. 1).
Increasing evidence has indicated that STAT3 activation is necessary for the malignant phenotype of many tumors.(25) Some previous studies have emphasized that STAT3 is a critical requirement for HIF-1α expression and that HIF-1α expression is blocked by STAT3 inhibitors.(24) In this study, we found that eupatilin inhibited STAT3 expression and markedly suppressed the activation of STAT3. Furthermore, we identified an interaction between HIF-1α and STAT3 at the VEGF promoter region using co-immunoprecipitation and ChIP assays, suggesting that both HIF-1α and STAT3 serve as transcriptional factors binding to the VEGF promoter. Indeed, increased interaction of HIF-1α to the VEGF promoter was observed in the hypoxia as expected (Fig. 2B). However, for reasons yet to be determined, the binding of ARNT and STAT3 to the promoter region was not significantly enhanced under hypoxic conditions compared to normoxia. Regardless, eupatilin was able to inhibit the recruitment of STAT3 to the VEGF promoter region in a dose-dependent manner. Interestingly, the magnitude of inhibition between hypoxic and normoxic conditions was comparable, indicating that eupatilin could inhibit nuclear translocation of the angiogenic pathway proteins regardless of oxygen concentration. Furthermore, it also implies that eupatilin could havedual functions in suppressing the angiogenesis pathway by direct inhibition of protein expression and by intervening with nuclear localization of these proteins. In order to properly respond to hypoxia thereby inducing VEGF expression, HIF-1α is required to interact with multiple proteins to modulate its stability and become activated.(23) In this respect, our data suggest that STAT3 should be considered as a new regulator of VEGF expression by participating in a transcriptional unit with HIF-1α and ARNT in gastric cancer cells. Importantly, this co-regulatory effect was inhibited by eupatilin.
In light of the results presented in this study, STAT3 inhibition and the subsequent reduction in VEGF expression could represent a potential mechanism for eupatilin-mediated suppression of the angiogenesis pathway. In addition, eupatilin treatment also inhibited xenograft tumor growth in mice. Taken together, the overall findings of our study indicate that the STAT3/HIF-1 pathway of VEGF expression is likely to be a main target of eupatilin action. Therefore, the inhibitory action of eupatilin on the expression of STAT3-mediated VEGF genes in tumors probably inhibits cell survival under hypoxia and may promote cell death in hypoxic areas by blocking angiogenesis.
In conclusion, the antioxidative flavone eupatilin significantly down-regulates STAT3 protein levels and activity under hypoxic conditions, eventually leading to the inhibition of angiogenesis and tumor suppression in gastric cancer cells. The therapeutic potential of phytopharmaceutical eupatilin should warrants future preclinical and clinical studies to realize a promise of anti-cancer effect by preventing tumor angiogenesis.
Acknowledgments
This study was supported by a faculty research grant of Yonsei University College of Medicine for 2005 (6-2005-0053). We thank Tae Young Oh at Dong-A Pharmaceutical Co. Ltd. (Yongin, Korea) for his generous gift of eupatilin and scientific discussion.
References
1. Folkman J. Clinical applications of research on angiogenesis. N Engl J Med. 1995; 333:1757–1763. PMID: 7491141.


2. Grunstein J, Roberts WG, Mathieu-Costello O, Hanahan D, Johnson RS. Tumor-derived expression of vascular endothelial growth factor is a critical factor in tumor expansion and vascular function. Cancer Res. 1999; 59:1592–1598. PMID: 10197634.
3. Millauer B, Shawver LK, Plate KH, Risau W, Ullrich A. Gliobalstoma growth inhibited in vivo by a dominant-negative Flk-1 mutant. Nature. 1994; 367:576–579. PMID: 8107827.
4. Shweiki D, Itin A, Soffer D, Keshet E. Vascular endothelial growth factor induced by hypoxia may mediate hypoxia-initiated angiogenesis. Nature. 1992; 359:843–845. PMID: 1279431.


5. Jiang BH, Zheng JZ, Aoki M, Vogt PK. Phosphatidylinositol 3-kinase signaling mediates angiogenesis and expression of vascular endothelial growth factor in endothelial cells. Proc Natl Acad Sci U S A. 2000; 97:1749–1753. PMID: 10677529.


6. Rak J, Yu JL, Klement G, Kerbel RS. Oncogenes and angiogenesis: signaling three-dimensional tumor growth. J Investig Dermatol Symp Proc. 2000; 5:24–33.


7. Ellis LM, Staley CA, Liu W, Fleming RY, Parikh NU, Bucana CD, et al. Down-regulation of vascular endothelial growth factor in a human colon carcinoma cell line transfected with an antisense expression vector specific for c-src. J Biol Chem. 1998; 273:1052–1057. PMID: 9422768.


8. Wiener JR, Nakano K, Kruzelock RP, Bucana CD, Bast RC Jr, Gallick GE. Decreased Src tyrosine kinase activity inhibits malignant human ovarian cancer tumor growth in a nude mouse model. Clin Cancer Res. 1999; 5:2164–2170. PMID: 10473101.
9. Wang GL, Jiang BH, Rue EA, Semenza GL. Hypoxia-inducible factor 1 is a basic-helix-loop-helix-PAS heterodimer regulated by cellular O2 tension. Proc Natl Acad Sci U S A. 1995; 92:5510–5514. PMID: 7539918.


10. Feldser D, Agani F, Iyer NV, Pak B, Ferreira G, Semenza GL. Reciprocal positive regulation of hypoxia-inducible factor 1alpha and insulin-like growth factor 2. Cancer Res. 1999; 59:3915–3918. PMID: 10463582.
11. Semenza GL. Regulation of mammalian O2 homeostasis by hypoxia-inducible factor 1. Annu Rev Cell Dev Biol. 1999; 15:551–578. PMID: 10611972.


12. Elson DA, Ryan HE, Snow JW, Johnson R, Arbeit JM. Coordinate up-regulation of hypoxia inducible factor (HIF)-1alpha and HIF-1 target genes during multi-stage epidermal carcinogenesis and wound healing. Cancer Res. 2000; 60:6189–6195. PMID: 11085544.
13. Bowman T, Garcia R, Turkson J, Jove R. STATs in oncogenesis. Oncogene. 2000; 19:2474–2488. PMID: 10851046.


14. Catlett-Falcone R, Dalton WS, Jove R. STAT proteins as novel targets for cancer therapy. Signal transducer an activator of transcription. Curr Opin Oncol. 1999; 11:490–496. PMID: 10550013.
16. Sinibaldi D, Wharton W, Turkson J, Bowman T, Pledger WJ, Jove R. Induction of p21WAF1/CIP1 and cyclin D1 expression by the Src oncoprotein in mouse fibroblasts: role of activated STAT3 signaling. Oncogene. 2000; 19:5419–5427. PMID: 11114718.


17. Niu G, Wright KL, Huang M, Song L, Haura E, Turkson J, et al. Constitutive Stat3 activity up-regulates VEGF expression and tumor angiogenesis. Oncogene. 2002; 21:2000–2008. PMID: 11960372.


18. Oh TY, Ryu BK, Ko JI, Ahn BO, Kim SH, Kim WB, et al. Protective effect of DA-9601, an extract of Artemisiae Herba, against naproxen-induced gastric damage in arthritic rats. Arch Pharm Res. 1997; 20:414–419. PMID: 18982482.
19. Oh TY, Lee JS, Ahn BO, Cho H, Kim WB, Kim YB, et al. Oxidative stress is more important than acid in the pathogenesis of reflux oesophagitis in rats. Gut. 2001; 49:364–371. PMID: 11511558.


20. Oh TY, Lee JS, Ahn BO, Cho H, Kim WB, Kim YB, et al. Oxidative damages are critical in pathogenesis of reflux esophagitis: implication of antioxidants in its treatment. Free Radic Biol Med. 2001; 30:905–915. PMID: 11295533.


21. Folkman J. Tumor angiogenesis: therapeutic implications. N Engl J Med. 1971; 285:1182–1186. PMID: 4938153.


22. Semenza GL. Hypoxia-inducible factor 1: oxygen homeostasis and disease pathophysiology. Trends Mol Med. 2001; 7:345–350. PMID: 11516994.


23. Gray MJ, Zhang J, Ellis LM, Semenza GL, Evans DB, Watowich SS, et al. HIF-1alpha, STAT3, CBP/p300 and Ref-1/APE are components of a transcriptional complex that regulates Src-dependent hypoxia-induced expression of VEGF in pancreatic and prostate carcinomas. Oncogene. 2005; 24:3110–3120. PMID: 15735682.
24. Xu Q, Briggs J, Park S, Niu G, Kortylewski M, Zhang S, et al. Targeting Stat3 blocks both HIF-1 and VEGF expression induced by multiple oncogenic growth signaling pathways. Oncogene. 2005; 24:5552–5560. PMID: 16007214.


25. Frank DA. STAT3 as a central mediator of neoplastic cellular transformation. Cancer Lett. 2007; 251:199–210. PMID: 17129668.


Fig. 1
Eupatilin inhibits the expression of angiogenesis gene products, HIF-1α, ARNT, STAT3 and VEGF. MKN45 cells were treated with indicated concentration of eupatilin before being cultured for 6 hr under normoxic (20% O2 v/v) or hypoxic (1% O2 v/v) conditions. Expression levels of HIF-1α, ARNT, STAT3, phospho-STAT3 were analyzed by immunoblotting. β-actin was used for loading control. Proteins were visualized by enhanced chemiluminescence. HIF-1α = hypoxia-inducible factor-1α; ARNT = aryl hydrocarbon receptor nuclear translocator; STAT3 = signal transducer and activator of transcription 3; VEGF = vascular endothelial growth factor.
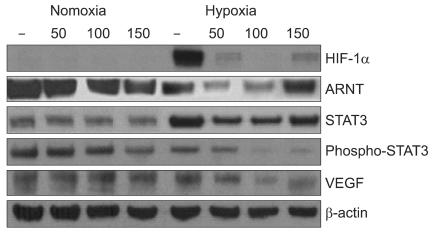
Fig. 2
STAT3 interacts with HIF-1 α and eupatilin inhibits STAT3 recruitment to the VEGF promoter. (A) MKN45 cells were incubated under normoxic or hypoxic conditions and cell lysates were immunoprecipitated with an anti-HIF-1α antibody, followed by Western blotting with an anti-ARNT, STAT3, and VEGF antibodies. STAT3, ARNT and VEGF co-precipitated with HIF-1α in hypoxic cells. (B) HIF-1α, STAT3 and ARNT are recruited to the VEGF promoter. Cross-linked, sheared chromatin was prepared from MKN45 cells grown in the absence or presence of eupatilin overnight. Chromatin samples were then immunoprecipitated with the antibodies indicated on the right. The precipitates were subjected to PCR analysis using primer pairs spanning the human VEGF promoter. The control was the PCR product of chromatin obtained before immunoprecipitation. The recruitment of HIF-1α, STAT3 and ARNT was greater under hypoxic conditions. Eupatilin treatment significantly inhibited the recruitment of STAT3, ARNT, and HIF-1α to the VEGF promoter region. IP = immunoprecipitation; HIF-1α = hypoxia-inducible factor-1α; ARNT = aryl hydrocarbon receptor nuclear translocator; STAT3 = signal transducer and activator of transcription 3; VEGF = vascular endothelial growth factor.
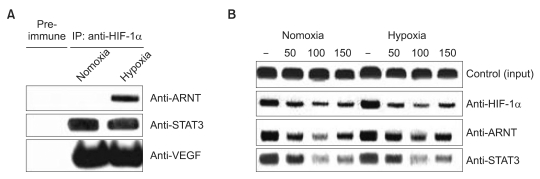
Fig. 3
Eupatilin inhibits in vitro capillary tube formation of HUVECs. HUVECs were seeded into 24-well plates coated with Matrigel at a density of 4×104 per well and cultured in the conditioned media either untreated or treated with eupatilin (50 or 100 µM). Aft er 16-h of incubation, photography was taken. (A) The representative photographs were shown (original magnification, ×40). (B) Summary of in vitro vasculogenesis assay reveals that eupatilin reduced the hypoxia-induced vascular formation in dose dependent manner. Each value represents mean±SD of 3 independent experiments (*P<0.001). All experiments were performed in triplicates. HUVEcs = human umbilical vein endothelial cells.
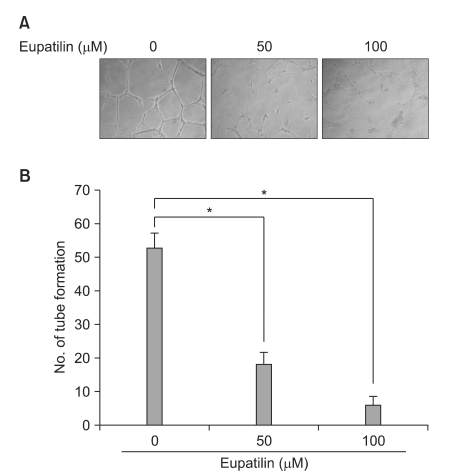
Fig. 4
The effect of eupatilin on the growth of xenografted human gastric cancer. MKN45 xenograft s on the flanks of mice treated with vehicle only (T) or with eupatilin (EPT) for 2 weeks. EPT (10 mg/kg) in 200 µl HBSS or 200 µl of HBSS only were administered three times a week by intraperitoneal injection. (A) Representative photograph of mice treated with either vehicle only (T) or EPT. The arrows indicate MKN45 tumors on mouse flank. (B) Ex vivo tumor weight of tumors from 4A. Each value represents mean±SD (*P<0.05).
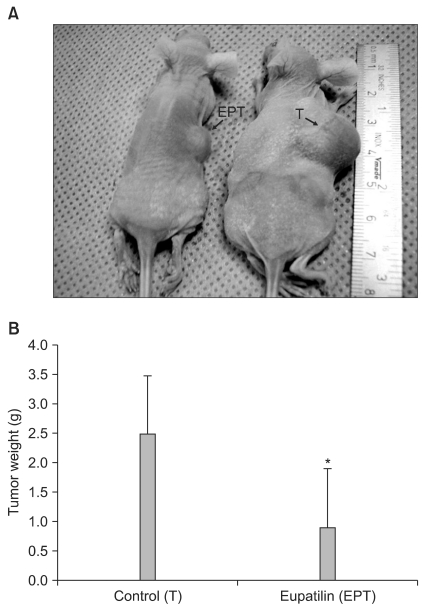