Abstract
Although quantitative evaluation of myocardial blood flow (MBF) and myocardial flow reserve (MFR) has been perceived as an attractive advantage of positron emission tomography (PET) over other cardiac imaging technologies, application of the information to specific coronary lesions is a difficult task for nuclear cardiologists. We hypothesized that changes in MBF and MFR over a coronary lesion could be identified by use of a hybrid technology of CT coronary angiography (CTCA) and N-13 ammonia PET. To evaluate this hypothesis, we measured the gradient of MBF and MFR through coronary stenosis in seven patients (M:F=3:4, median age 56 years) with coronary artery disease who underwent N-13 ammonia PET, CTCA, and interventional coronary angiography. Two patients had proximal left anterior descending (LAD) coronary artery disease and five patients had mid to distal LAD disease. Mean global stress and rest MBF were 2.62±0.58 and 1.03±0.19 ml/min/g, respectively. Mean global MFR was 2.6±0.73. Regional stress and rest MBF in the LAD territory were 2.36±0.75 and 0.96±0.21 ml/min/g, respectively. Regional MFR in the LAD territory was 2.55±0.83 ml/min/g. Stress MBF changed dramatically according to the location of coronary stenosis. It dropped acutely in proximal lesions, whereas it diminished gradually in mid to distal lesions. In conclusion, by use of a hybrid technology of CTCA and PET, it was feasible to make a direct correlation of coronary lesions with the gradient of MFR and CFR through coronary stenosis, which indicated the severity of the coronary lesion. We named this technique indirect radionuclide coronary angiography.
Noninvasive evaluation of coronary stenosis severity remains a challenge despite vast achievements in developing new technologies of positron emission tomography with computed tomography (PET/CT), CT coronary angiography (CTCA), magnetic resonance imaging (MRI), and echocardiography. Quantitation of myocardial blood flow (MBF) and flow reserve (MFR) by use of PET confers advantages over other modalities related to the functional evaluation of the severity of coronary stenosis1; however, the exact localization of the culprit lesion is difficult because of the difference in imaging formats. The evaluation of blood flow from the epicardial coronary artery down to the microcirculation is possible only by using MFR, which therefore provides functional information on the whole myocardium including the endocardium.2 Single photon emission computed tomography provides relative MBF or flow heterogeneity induced by hyperemic stress, which sometimes underestimates the extent of coronary artery disease (CAD) because it depends on the most severely underperfused territory.3 Absolute measurement of MBF and MFR can indicate the true extent of CAD, which helps in the early diagnosis of coronary atherosclerosis. Recent publications have shown the added value of MFR using either N-13 ammonia4,5 or Rb-82.6,7 Although many studies have revealed the value of MBF and MFR of the global left ventricle (LV), values of diseased artery territories have not been assessed as compared with the whole LV values. PET/CT provides information on both coronary anatomy as well as MBF and MFR. Therefore, evaluation of changes in MBF and MFR are possible through coronary arteries and each specific lesion. We hypothesized that change in MBF and MFR over a coronary lesion could be identified by use of the hybrid technology of CTCA and N-13 ammonia PET. To evaluate this hypothesis, we measured the gradient of MBF and MFR through coronary stenosis in patients with CAD who underwent N-13 ammonia PET, CTCA, and interventional coronary angiography (CAG).
The subjects of this study were seven consecutive patients (Table 1) who underwent N-13 ammonia PET, CTCA, and CAG. The study was approved by the institutional review board and written informed consent was received from all subjects.
The image acquisition protocol for N-13 ammonia PET/CT was described previously.8,9 Briefly, the patients were instructed to fast for at least 4 hours and to stop methylxanthine derivatives at least 24 hours before image acquisition. Vasodilator medications such as nitrates, beta blockers, or calcium channel blockers were also stopped for 24 hours before image acquisition. Appropriate medical history was obtained for all subjects.
After a low-dose CT scan (120 kVp, 30 mA) for attenuation correction, 11 MBq/kg (0.3 mCi/kg) of N-13 ammonia was injected as a bolus within 15 s. A dynamic scan for 6 minutes and electrocardiogram (ECG)-gated scan for 13 minutes followed for rest imaging data. After 1 hour, 0.14 mg/kg/min of adenosine was intravenously infused for 6 minutes into the opposite arm vein for vasodilator stress. N-13 ammonia was injected at peak stress (3 minutes after the infusion had started). The image acquisition was performed by a dedicated PET scanner with a BGO crystal and 8-slice CT scanner (Discovery ST, GE Healthcare, USA), and image reconstruction was performed by using ordered subset expectation maximization iterative reconstruction.
Quantitative MBF was assessed by using PMOD software (version 3.2; PMOD Technologies Ltd., Zurich, Switzerland).4 Briefly, a spheric region of interest was set into the blood pool of the LV. Blood pool and myocardial time-activity curves were determined from dynamic frames and corrected for radioisotope decay. MBF was assessed by model fitting of the blood pool and myocardial time-activity curves,10 with correction for partial volume and spillover as previously reported.11 MFR was determined as the ratio of hyperemic MBF to resting MBF, and a value of less than 2.0 was considered abnormal as previously suggested2 and supported by prognostic data5.
The CT acquisitions were performed with a two-phase, contrast-enhanced, ECG-gated, multi-detector CT scanner (Sensation Cardiac 64, Siemens, Forchheim, Germany). Section thickness was 0.75 mm, gantry rotation time was 330 ms, and the tube current was 800 mAs at 120 kVp. The pitch was determined as 0.2. Serial CT scanning in the axial plane was performed from the level of the left ventricular apex after a bolus injection of 60 ml of nonionic contrast media (Ultravist 370®; Bayer Schering Pharma, Berlin, Germany) followed by a 60-ml saline bolus injection, both of which were injected at 4 ml/s. The axial images were reconstructed at multiple phases that covered the cardiac cycle in increments of 10% of the RR interval between 5% and 95%. Multiphase reconstruction was performed by using short axis slices from the base to the apex of the heart with the use of the commercially available software Argus® (Siemens).
The ECG-gated CT images were post-processed with the GE Advantage Volume Share Workstation and interpreted by expert observers blinded to all clinical data. The borderline of proximal and mid LAD was the origin of the first diagonal branch, and obstructive CAD was defined as a diameter stenosis>50%.12-14
Integration of PET perfusion findings with CTA images was performed on a designated workstation (Advantage Workstation 4.3, GE Healthcare) by using the CardIQ Fusion software package. This software allows the overlay of functional information over the CT anatomy of a patient's heart.15 On the fusion QC protocol, which is the first step of the image fusion workflow, the images were aligned on axial, sagittal, and coronal planes. Following image alignments, epicardium QC was performed to display a view containing the segmented CT LV epicardium by use of a conventional volume-rendering technique. We added or removed epicardial structures comparing the outline of uptake of N-13 ammonia and the anatomical information on CT. The Hybrid Display, which is the last step of image fusion, was performed to display the coronary artery on fused myocardial images by growing the coronary arteries from the ascending aorta by use of automatic vessel tracking or manual segmentation. We segmented the LAD into three parts from base to apex (proximal, mid, and distal), drew four regions of interest (ROIs) for each segment (12 ROIs totally), and measured MBF and MFR.
CAG was performed by experienced interventional cardiologists according to clinical standards. A coronary stenosis was defined as a luminal narrowing of 50% or more by 2 independent interventional cardiologist observers. In cases of discrepancy, the decision was made by consensus on the basis of visual assessment, reflecting clinical routine in most catheterization laboratories.16
Quantitative variables were expressed as means±SDs, and categorical variables as percentages. SPSS 18 for Windows (SPSS Inc., Chicago, IL, USA) was used for statistical analysis. Comparison of MBF and CFR between diseased and normal coronary artery territories was done by use of Students' t-test. Comparison of MBF and MFR before and after double therapy was done by paired t-test. A value of p<0.05 was considered statistically significant.
Three male and four female patients with chest pain were included in this study (Table 1). The median age of the patients was 56 years (range, 51-72 years). All patients underwent CTCA, N-13 ammonia PET/CT, and CAG.
Two patients had proximal LAD disease and five patients had mid to distal LAD disease. One patient who showed a diffuse narrowing from proximal to distal was grouped as mid to distal LAD disease according to the severity of the disease. Mean global stress and rest MBF were 2.62±0.58 and 1.03±0.19 ml/min/g, respectively. Mean global MFR was 2.6±0.73. Regional stress and rest MBF in the LAD territory were 2.36±0.75 and 0.96±0.21 ml/min/g, respectively. Regional MFR in the LAD territory was 2.55±0.83. Stress MBF showed a higher correlation coefficient to TIMI flow grade of CAG (r=0.33) than to rest MBF (r=0.11), neither of which reached statistical significance.
Stress MBF changed dramatically according to the location of coronary stenosis. It dropped acutely in proximal lesions while it diminished gradually in mid to distal lesions as shown in Fig. 1. Case illustrations of proximal LAD disease (Fig. 2) and mid to distal LAD disease (Fig. 3) were given. Stress MBF was diminished, whereas rest MBF was maintained. MFR was diminished in both cases.
This article has demonstrated a new concept: the severity of coronary artery stenosis can be illustrated by changing patterns of MBF through a diseased LAD artery, which indicates the functional severity of the specific coronary lesion. We have termed this method indirect radionuclide coronary angiography (IRCA). An acute drop of stress MBF was a typical finding of proximal LAD disease, whereas a gradual decrease of stress MBF was the finding in mid to distal LAD disease.
The extent and degree of the perfusion defect measured by myocardial perfusion imaging (MPI) were regarded as indicators of the coronary lesion. The localization of coronary lesions is presumed from the location of the perfusion defect, which is frequently not matched to real coronary lesions because of variations in coronary anatomy. Although the hybrid technology of CTCA and PET opened a possibility of pinpointing culprit coronary lesions, there are several barriers to overcome. First, coronary lesions with intermediate severity detected by CTCA are false-positive in almost half of cases. Second, defining the perfusion defect from the 3D image of MPI is frequently confusing. Third, correlation of anatomical and functional lesions is arbitrary.
Recently, fractional flow reserve (FFR) with the use of hyperemic coronary pressure measurement during CAG has been introduced as an accurate methodology for discriminating ischemic from nonischemic conditions. FFR has become an important index for decision making for revascularization of coronary artery lesions.17 An advantage of FFR measurement is that coronary intervention can be done within the same session. A disadvantage of FFR is its invasiveness. We hypothesized that tracing blood flow changes through the coronary artery by using a hybrid technique of CTCA and PET would provide information on perfusion change in the same way as FFR, which traces pressure changes through the coronary artery lesion. We named this method indirect coronary angiography because it shows the coronary blood flow in an indirect way, and we added the term radionuclide because we used it. Thus, the name of the method became IRCA.
IRCA has several advantages for clinical use. Most of all, it is noninvasive. It shows the severity of the coronary artery lesion. It can pinpoint the location of the culprit lesion. The clinical usefulness of IRCA should be verified by a gold standard. Therefore, a direct comparison of FFR with IRCA may be a future and promising research theme.
The ability of cardiac PET to measure MBF and MFR has been documented by numerous reports that showed an inverse relationship between the severity of coronary luminal narrowing and MFR. A clinical diagnostic added value of MFR over MPI was also reported.4 Adding functional data improves the interpretation of angiographic findings, and adding MFR improves the interpretation of MPI. Normal MPI findings can be reclassified by as much as one third by adding MFR data.4 MFR improves accuracy to above the range of sensitivity reported in a metaanalysis.18
The present study showed that regional MFR in the LAD territory shows valuable information for discriminating proximal from mid to distal LAD lesions. There are numerous reports supporting the usefulness of global MFR.19-24 Global MFR reflects coronary disease beyond the epicardial section of the coronary tree involving the microcirculation, including endothelial dysfunction. This supports recent findings in patients with diabetes mellitus19 and helps to explain the increased sensitivity in detecting CAD when global MFR is used as an adjunct to PET MPI. Use of global MFR seems preferable over regional MFR practically because the reproducibility and the repeatability of global MFR is considerably superior to that of regional MFR.20,21 This observation is based not only on technical issues and count statistics of small sample sizes but also on biological variability, as a profound spatial heterogeneity has been observed in regional MBF at rest and in response to vasodilator stress.22,23 The anatomical variability of the coronary tree is another source that might contribute to the lower per-segment performance, because the measurements of accuracy are based on assumptions of standard myocardial distribution territories that correspond in only 50% to 60% with the real anatomical coronary tree.24 Nevertheless, we believe that regional MFR provides valuable information if it is measured according to coronary artery territory.
This study had several limitations. First, the number of subjects was small. The purpose of this paper was to introduce a concept. Despite the small sample size, the results were informative. Second, we analyzed only LAD lesions. LAD is most appropriate for demonstrating perfusion changes because it is long and covers a large area. Application of this technique to other coronary arteries can be a next research topic. Third, we did not measured FFR in this study. FFR is expensive and is not yet covered by medical insurance. Direct comparison of IRCA with FFR is definitely an important research area.
In conclusion, by use of a hybrid technology of CTCA and PET, it was possible to make a direct correlation between the coronary lesion and the gradient of MFR and CFR through coronary stenosis, and this indicated the severity of the coronary lesion. We named this technique indirect radionuclide coronary angiography.
ACKNOWLEDGEMENTS
This study was supported by a grant (A070001) from the Korea National Enterprise for Clinical Trials.
References
1. Ghosh N, Rimoldi OE, Beanlands RS, Camici PG. Assessment of myocardial ischaemia and viability: role of positron emission tomography. Eur Heart J. 2010; 31:2984–2995. PMID: 20965888.
2. Kaufmann PA, Camici PG. Myocardial blood flow measurement by PET: technical aspects and clinical applications. J Nucl Med. 2005; 46:75–88. PMID: 15632037.
3. Lima RS, Watson DD, Goode AR, Siadaty MS, Ragosta M, Beller GA, et al. Incremental value of combined perfusion and function over perfusion alone by gated SPECT myocardial perfusion imaging for detection of severe three-vessel coronary artery disease. J Am Coll Cardiol. 2003; 42:64–70. PMID: 12849661.
4. Fiechter M, Ghadri JR, Gebhard C, Fuchs TA, Pazhenkottil AP, Nkoulou RN, et al. Diagnostic value of 13N-ammonia myocardial perfusion PET: added value of myocardial flow reserve. J Nucl Med. 2012; 53:1230–1234. PMID: 22776752.
5. Herzog BA, Husmann L, Valenta I, Gaemperli O, Siegrist PT, Tay FM, et al. Long-term prognostic value of 13N-ammonia myocardial perfusion positron emission tomography added value of coronary flow reserve. J Am Coll Cardiol. 2009; 54:150–156. PMID: 19573732.
6. Fukushima K, Javadi MS, Higuchi T, Lautamäki R, Merrill J, Nekolla SG, et al. Prediction of short-term cardiovascular events using quantification of global myocardial flow reserve in patients referred for clinical 82Rb PET perfusion imaging. J Nucl Med. 2011; 52:726–732. PMID: 21498538.
7. Ziadi MC, Dekemp RA, Williams KA, Guo A, Chow BJ, Renaud JM, et al. Impaired myocardial flow reserve on rubidium-82 positron emission tomography imaging predicts adverse outcomes in patients assessed for myocardial ischemia. J Am Coll Cardiol. 2011; 58:740–748. PMID: 21816311.
8. Henzlova MJ, Cerqueira MD, Mahmarian JJ, Yao SS. Quality Assurance Committee of the American Society of Nuclear Cardiology. Stress protocols and tracers. J Nucl Cardiol. 2006; 13:e80–e90. PMID: 17174798.
9. Lee BI, Kim KH, Kim JY, Kim SJ, Lee JS, Min JJ, et al. Correlation between semiquantitative myocardial perfusion score and absolute myocardial blood flow in 13N-ammonia PET. Nucl Med Mol Imaging. 2007; 41:194–200.
10. Muzik O, Beanlands RS, Hutchins GD, Mangner TJ, Nguyen N, Schwaiger M. Validation of nitrogen-13-ammonia tracer kinetic model for quantification of myocardial blood flow using PET. J Nucl Med. 1993; 34:83–91. PMID: 8418276.
11. Hutchins GD, Schwaiger M, Rosenspire KC, Krivokapich J, Schelbert H, Kuhl DE. Noninvasive quantification of regional blood flow in the human heart using N-13 ammonia and dynamic positron emission tomographic imaging. J Am Coll Cardiol. 1990; 15:1032–1042. PMID: 2312957.
12. Chow BJ, Abraham A, Wells GA, Chen L, Ruddy TD, Yam Y, et al. Diagnostic accuracy and impact of computed tomographic coronary angiography on utilization of invasive coronary angiography. Circ Cardiovasc Imaging. 2009; 2:16–23. PMID: 19808560.
13. Chow BJ, Wells GA, Chen L, Yam Y, Galiwango P, Abraham A, et al. Prognostic value of 64-slice cardiac computed tomography severity of coronary artery disease, coronary atherosclerosis, and left ventricular ejection fraction. J Am Coll Cardiol. 2010; 55:1017–1028. PMID: 20202518.
14. Hoffmann U, Moselewski F, Cury RC, Ferencik M, Jang IK, Diaz LJ, et al. Predictive value of 16-slice multidetector spiral computed tomography to detect significant obstructive coronary artery disease in patients at high risk for coronary artery disease: patient-versus segment-based analysis. Circulation. 2004; 110:2638–2643. PMID: 15492297.
15. Gaemperli O, Schepis T, Kalff V, Namdar M, Valenta I, Stefani L, et al. Validation of a new cardiac image fusion software for three-dimensional integration of myocardial perfusion SPECT and stand-alone 64-slice CT angiography. Eur J Nucl Med Mol Imaging. 2007; 34:1097–1106. PMID: 17245532.
16. Tonino PA, De Bruyne B, Pijls NH, Siebert U, Ikeno F, van' t Veer M, et al. FAME Study Investigators. Fractional flow reserve versus angiography for guiding percutaneous coronary intervention. N Engl J Med. 2009; 360:213–224. PMID: 19144937.
17. Pijls NH. Fractional flow reserve to guide coronary revascularization. Circ J. 2013; 77:561–569. PMID: 23420635.
18. Nandalur KR, Dwamena BA, Choudhri AF, Nandalur SR, Reddy P, Carlos RC. Diagnostic performance of positron emission tomography in the detection of coronary artery disease: a meta-analysis. Acad Radiol. 2008; 15:444–451. PMID: 18342769.
19. Schindler TH, Facta AD, Prior JO, Cadenas J, Zhang XL, Li Y, et al. Structural alterations of the coronary arterial wall are associated with myocardial flow heterogeneity in type 2 diabetes mellitus. Eur J Nucl Med Mol Imaging. 2009; 36:219–229. PMID: 18704406.
20. Kaufmann PA, Gnecchi-Ruscone T, Yap JT, Rimoldi O, Camici PG. Assessment of the reproducibility of baseline and hyperemic myocardial blood flow measurements with 15O-labeled water and PET. J Nucl Med. 1999; 40:1848–1856. PMID: 10565780.
21. Wyss CA, Koepfli P, Mikolajczyk K, Burger C, von Schulthess GK, Kaufmann PA. Bicycle exercise stress in PET for assessment of coronary flow reserve: repeatability and comparison with adenosine stress. J Nucl Med. 2003; 44:146–154. PMID: 12571202.
22. Austin RE Jr, Aldea GS, Coggins DL, Flynn AE, Hoffman JI. Profound spatial heterogeneity of coronary reserve. Discordance between patterns of resting and maximal myocardial blood flow. Circ Res. 1990; 67:319–331. PMID: 2376074.
23. Chareonthaitawee P, Kaufmann PA, Rimoldi O, Camici PG. Heterogeneity of resting and hyperemic myocardial blood flow in healthy humans. Cardiovasc Res. 2001; 50:151–161. PMID: 11282088.
24. Javadi MS, Lautamäki R, Merrill J, Voicu C, Epley W, McBride G, et al. Definition of vascular territories on myocardial perfusion images by integration with true coronary anatomy: a hybrid PET/CT analysis. J Nucl Med. 2010; 51:198–203. PMID: 20080895.
FIG. 1
Gradient of myocardial blood flow during adenosine infusion in patients with proximal (A) or mid to distal (B) left anterior descending artery (LAD) disease. Perfusion drop was significant only in the basal portion of the myocardium in the proximal LAD disease group, whereas it was gradually diminished in the apical portion in the mid to distal LAD disease group.
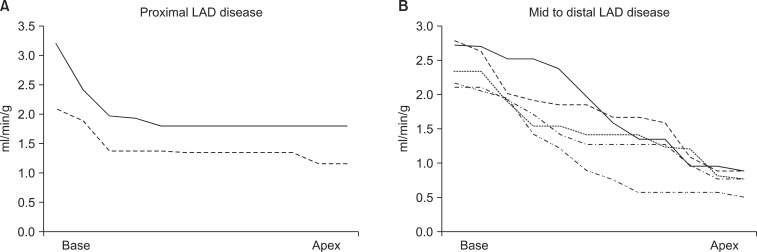
FIG. 2
(A) Stress perfusion graph of a 59-year-old male patient with unstable angina showing an acute perfusion drop in the base of the left ventricle, the proximal LAD territory, whereas the rest perfusion graph shows a steady state of myocardial blood flow. (B) Myocardial flow reserve (MFR) diminished from the left ventricular base to the apex. The reference cutoff value of MFR is 2.0 at the authors' institute. (C) Interventional coronary angiography showing a discrete stenotic lesion (arrow) in the proximal LAD. (D) Hybrid image of CT angiography and stress perfusion image of N-13 ammonia PET showing cutting of proximal left anterior descending artery (LAD), which correlated with the large perfusion defect in the LAD territory.
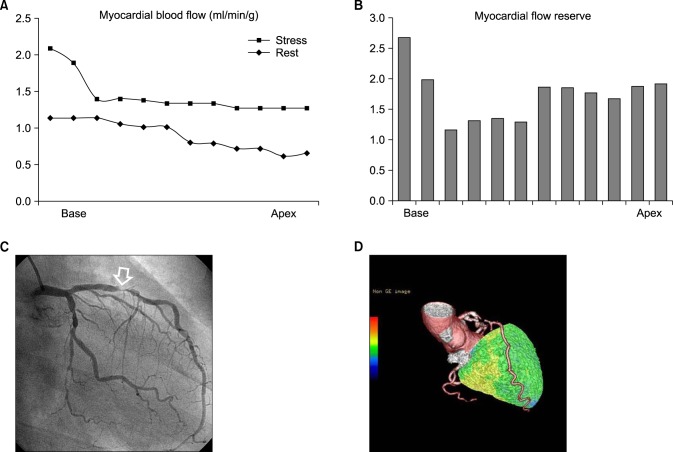
FIG. 3
(A) Stress perfusion graph in a 72-year-old male patient with typical angina showing a gradual decrease of perfusion through the left ventricle from the base to the apex, whereas the rest perfusion graph shows a steady state of myocardial blood flow. (B) Myocardial flow reserve (MFR) was lower than 2.0 from the mid portion to the apex of the left ventricle, whereas the basal portion maintained normal MFR. (C) Interventional coronary angiography showing a long stenotic lesion in the mid portion of the left anterior descending artery (mLAD, arrow). (D) Hybrid image of CT angiography and stress perfusion image of N-13 ammonia PET showing a narrowing in mLAD that was correlated with a perfusion defect in the apicoseptal area.
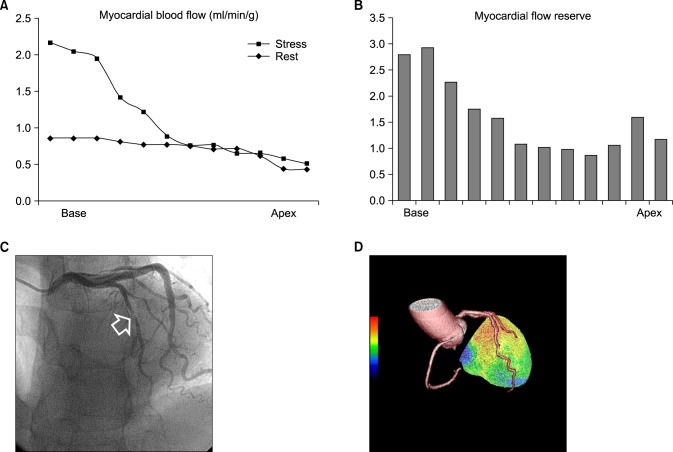