Abstract
Over the past three decades, radionuclide myocardial perfusion scintigraphy (MPS) has become established as the main functional cardiac imaging technique for ischemic heart disease. It is currently appropriate for all aspects of detecting and managing ischemic heart disease, including diagnosis, risk assessment and stratification, assessment of myocardial viability, and evaluation of left ventricular function. The purpose of this article was to review recent trends in nuclear cardiology practice, excluding positron emission tomography. The past few years have brought several rapid developments that have increased photon sensitivity in nuclear cardiology scanner hardware. Additionally, software applying new methods of single photon emission tomography (SPECT) reconstruction on conventional and dedicated systems has preserved or even improved SPECT image quality with lower count statistics. On the other hand, much interest has been shown in lowering the radiation dose by the stakeholders of MPS.
Over the past three decades, radionuclide myocardial perfusion scintigraphy (MPS) has become established as the main functional cardiac imaging technique for measuring and assessing the manifestations of ischemic heart disease (IHD). Cardiac single photon emission tomography (SPECT) is a widely used noninvasive nuclear image acquisition technique for investigating IHD. SPECT is currently appropriate for all aspects of detecting and managing IHD, including diagnosis, risk assessment and stratification, assessment of myocardial viability, and evaluation of left ventricular function.1 More recently, hybrid images combining the functional imaging of SPECT and computed tomography (CT) coronary angiography have enabled improved diagnosis, risk stratification, and treatment planning for patients with suspected coronary artery disease.2
The field of nuclear cardiology is ever expanding with newer indications for imaging the heart. Technical advancements in instrumentation together with better radiopharmaceuticals have revolutionized the field. On the other hand, much interest has been paid to lowering the radiation dose by the stakeholders of MPS. In this article, recent developments and trends in nuclear cardiology practice, with the exception of positron emission tomography, are reviewed.
In current practice, SPECT imaging has superseded planar perfusion imaging. SPECT is defined as tomographic scintigraphy from three-dimensional images of radioactive tracer distribution that are produced by the detection of single photons (γ-rays) from acquired multiple planar images. The cross-sectional images have high resolution and can delineate myocardial perfusion in various regions. It is widely used to evaluate myocardial perfusion as well as ventricular function in patients with suspected IHD. Since its introduction for routine clinical practice in the late 1980s, the principle has remained the same.
Although MPS is widely used in current clinical practice, it has some fundamental limitations, including a long image acquisition time, low image resolution, and the dose of patient radiation exposure. Therefore, reducing patient radiation exposure, as well as shortening acquisition time, has become an important issue in MPS. The radiation dose and acquisition time are intrinsically linked, because longer acquisition times can be used with lower injected doses and higher doses can be used to shorten acquisition times. High-sensitivity dedicated cardiac camera systems provide an opportunity to lower the injected doses for SPECT MPS. The past few years have brought several rapid developments that have increased photon sensitivity in nuclear cardiology scanner hardware (Figs. 1 and 2). Several new dedicated camera systems with optimized acquisition geometry and collimator design resulting in increased count sensitivity have been introduced by vendors.3-9 As a result, acquisition times for MPS can be significantly shortened without a significant sacrifice of accuracy. In particular, a recent study on dedicated cardiac scanners with cadmium-zinc-telluride detectors found that a 10-min stress scan could be performed with an effective average radiation dose of less than 1 mSv.10
Attenuation correction to reduce soft-tissue attenuation artifacts was introduced by using external beam (transmission) images with the use of either a γ-emitting source or, more commonly, low-dose CT in conjunction with SPECT imaging (SPECT/CT). This has inherent problems, but it is generally accepted that attenuation correction helps to improve the accuracy in reporting.11 The practice of combining MPS with other modalities such as coronary CT angiography for better diagnostic certainty12,13 has intensified concerns regarding the total radiation dose delivered to the patient.14
There are now several camera systems that combine the fast acquisition gamma cameras with high-resolution cardiac CT (hybrid cardiac cameras). Image co-registration using these hybrid systems enables more informed and better interpretation of both coronary CT findings as well as nuclear medicine perfusion imaging.15,16 Combined images enable better diagnosis, stratification, and treatment planning for patients with suspected coronary artery disease.
Backprojection reconstruction of images has now been mostly replaced by iterative reconstruction. Iterative reconstruction requires high computing power because the calculation is intensive. Although it has been routinely available in nuclear medicine since the 1990s, its use has been widespread only in the last decade. This method of reconstruction has reduced the artifacts from adjacent structures with high signal intensity such as the liver and gut.
Software development for image acquisition and reconstruction has led to faster image acquisition and improved image quality while decreasing radiation exposure to the patient. Several manufacturers have introduced software applications that incorporate iterative reconstruction, resolution recovery, and noise reduction, and these solutions have provided good-quality SPECT images despite reduced count statistics. Owing to the new methods of SPECT reconstruction applied in the software, SPECT image quality has been preserved or even improved with lower count statistics.17-24
Reliable automatic normalization of myocardial activity for rest and stress images is necessary for comparison and interpretation of MPS. For example, extracardiac hot spots such as gallbladder or gastrointestinal tract activity can seriously disturb the cardiac image quality. The development of cardiac packages has enabled reliable automatic normalization of myocardial activity.25 In addition, comparison with normal libraries can help to identify artifacts due to breast or diaphragm and aid in correct interpretation of images.26
The reporting aid software has enabled more accurate and objective assessment of the heart. Several new software packages are now available for displaying images. The new display software packages have better edge detection algorithms with better display of normalized images. The introduction of extensive normal libraries has enabled more accurate semiquantitative assessment of left ventricular perfusion.
There is now a growing literature on the uses of MPS in heart failure. In addition to providing information on perfusion, MPS can give an accurate assessment of both systolic and diastolic left ventricular function, including phase and movement asynchrony, which, in conjunction with CT anatomy information from hybrid imaging, can be used in planning for positioning of various intracardiac devices.27-29 With new developments in cameras and software, these studies can be performed in a few minutes without the need for the patient to be in a supine position for long periods, which can be difficult for heart failure patients.
Patient motion is a common cause of artifacts on MPS. Motion on SPECT studies can be detected in several ways. Breaks or irregularities in the sinogram indicate motion. This display method is not always easy to interpret, however. Furthermore, sinograms do not show horizontal motion. A simple and more commonly used method for detecting patient motion involves inspection of the cine display of all planar projection images, i.e., rotating images. If motion is detected, a motion-correction program can be applied.30
Various radiopharmaceuticals are available for imaging the myocardium (Table 1). They all play an important role in the diagnosis and management of ischemic heart disease. The choice of radiopharmaceuticals should be based on the clinical questions to be answered. The choice of compound depends on a weighing of the advantages and disadvantages of each tracer and a clinical decision as to which will give the best information.
Tl-201 chloride was the first radiopharmaceutical to be widely used clinically for imaging myocardial perfusion.31 Tl-201 is a cyclotron-produced radionuclide with a half-life of 73 hours. Extensive research with cell culture, isolated heart, and intact animal models showed that thallous ion has a relatively high myocardial extraction, in part via Na+-K+ ATPase, that allows for noninvasive assessment of relative regional myocardial blood flow,32 and is redistributed fairly rapidly, starting 20 min after injection.33 First used for myocardial scintigraphy in the mid-1970s, Tl-201 remained the only perfusion agent available until 1990. Tl-201 is an excellent agent for imaging cardiac perfusion and is still used in many centers. However, because of its relatively long half-life (73 hours) and low-energy X-ray emission (69 to 83 keV; 95% abundant), it is not an ideal agent for MPS, giving a relatively large radiation dose with lower image quality than technetium-based agents.
In the late 1980s, Tc-99m labeled perfusion agents were studied as myocardial perfusion agents in several experimental models and in human subjects.34 Two technetium-based agents, namely sestamibi and tetrofosmin, are now both widely used. Production of Tc-99m (extracted from the generator system) is more convenient compared with Tl-201, and there is no or minimal redistribution. Thus, MPS with Tc-99m-labeled perfusion agents can be obtained many hours later with no loss of diagnostic accuracy. This makes the planning and timing of imaging more convenient. Also, if the images are unsatisfactory (movement or gut activity), rescanning can be performed. Because of the better photon characteristics, lower radiation dose to the patient, and higher injection activity (5-10 times that of thallium), which more than compensates for the relatively lower myocardial extraction percentage, better images can be acquired in a shorter time.
Faster imaging acquisition has been enabled by new technological advances. Therefore, faster redistributing agents such as Tc-99m teboroxime34 and Tc-99m 15C5-1-PNP,35 which were impractical because of redistribution that was too rapid for standard 20-min imaging using standard gamma cameras, may be reintroduced for MPS with the new advanced cardiac scanners.
Metaiodobenzyl guanidine (MIBG) is an analogue of guanethidine and behaves in a manner that is qualitatively similar to that of norepinephrine, a transmitter of the adrenergic system in the heart. MIBG is labelled with I-123 and its half-life and energy are 13.3 hours and 159 keV (83%), respectively. I-123 MIBG is the most commonly used tracer for cardiac innervation imaging. After MIBG injection, the tracer is internalized by presynaptic nerve endings of postganglionic neuronal cells through the energy-dependent uptake-1 system. In addition, MIBG enters the presynaptic nerve endings through the uptake-2 system. After neuronal depolarization, MIBG is released in the synapse cleft but is not metabolized by enzymes as norepinephrine.
The ratio of uptake in the myocardium (heart) to the mediastinum is used to semiquantitatively assess sympathetic innervation of the myocardium.36,37 This measurement has incremental value for the assessment of symptoms and left ventricular ejection fraction in the risk stratification and prognostication of patients with cardiac failure and the assessment of sympathetic innervation in various cardiac diseases.38,39 I-123 MIBG cardiac scan has been shown to predict response to β-adrenergic blockade and to be useful in better selection of patients for implantable cardioverter defibrillators.36
15-(p-Iodophenyl)3-R, S-Methylpentadecanoic acid (BMIPP) is a beta-methyl fatty acid analogue in which methyl branching is introduced to inhibit beta-oxidation. BMIPP undergoes slower oxidation and clearance by incorporation into the endogenous lipid pool, which results in prolonged residence time. Because the agent is retained in the myocardium for some time, it is more useful for SPECT imaging with a conventional gamma camera. BMIPP is usually injected under fasting conditions and imaging is performed 20 to 30 minutes after administration. BMIPP is a sensitive indicator of metabolic alterations in ischemic myocardium. The areas with reduced uptake of BMIPP are felt to represent ischemic but viable myocardium, and these areas have been shown to demonstrate a metabolic-perfusion mismatch on PET studies.40
During ischemia, fatty acid utilization is reduced as the myocardial metabolism shifts from fatty acid to glucose utilization for the production of energy. This shift persists for up to 12 h after the ischemic event has subsided; thus, BMIPP injection after the ischemic event can be used to image the past ischemic event (ischemic memory imaging).41,42 Fatty acid imaging together with rest perfusion imaging can be used to diagnose ischemia in suspected acute coronary syndrome presenting in emergency departments. A recent study demonstrated a sensitivity of 74% and a specificity of 92% in patients presenting to emergency units with acute coronary syndrome.43
Patient safety is of paramount importance when contemplating any diagnostic or therapeutic medical option. For myocardial perfusion imaging (MPI), this approach includes not only the risk of the stress protocol but also the "risk" of performing unnecessary additional procedures or administering inappropriate therapy because of a suboptimally performed test. High-quality imaging limits the latter risk through improved diagnostic sensitivity and specificity, enhanced risk stratification, and less intra- and interobserver variability when interpreting clinically significant changes in serial images.
Once the safety concerns are addressed, it is critical to ensure that the proper imaging protocol is used to best answer the clinical question at hand (Fig. 3). Meeting this priority requires close communication between the referring physician and those performing the test.44
Many MPI protocols exist (Table 2), all of which have unique advantages and disadvantages. One size does not fit all. The most appropriate protocol for the patient should be pursued.
In a recent American Society of Nuclear Cardiology (ASNC) information statement, stress-only imaging was recommended when applied to properly selected patients.45 Stress-only imaging has several advantages. These are improved patient convenience and satisfaction, improved laboratory efficiency, marked reduction in patient radiation exposure by eliminating the higher rest Tc-99m dose, and reduced cost by eliminating injection of a second Tc-99m dose and the subsequent rest imaging. There are no specific disadvantages to a stress-only imaging approach.
Several factors may influence the type of stressor selected for an individual patient. The most practical issue is the patient's ability to exercise. A patient's ability to adequately exercise can be assessed informally on the basis of the activity level described in the patient's history or in a more formal manner by applying a physical activity questionnaire.46
An advantage of exercise as a stressor is the additional prognostic information gained from the stress test. The most important prognostic variables are exercise duration and the extent of exercise-induced ST-segment depression. Other important variables include exercise-induced angina, hypotension, ventricular ectopy, chronotropic incompetence, and impaired heart rate recovery after exercise.47 Another useful application of the exercise test is to observe whether the patient's chest pain is reproduced during stress to determine whether its etiology is cardiac or noncardiac. For these reasons, exercise stress is preferred over pharmacologic testing when an adequate exercise effort can be achieved.
The most commonly used type of exercise in the United States is treadmill testing. The Bruce protocol is most commonly used in which most patients complete a workload of 7-13 METs over 6-12 minutes. Several other protocols (modified Bruce, Naughton, Balke) increase workload in smaller increments, which is an advantage in elderly or frail patients. Bicycle ergometry is widely applied in Europe. Other forms of exercise testing, such as arm ergometry, are rarely used.
Beyond the inability to exercise, additional indications for vasodilator stress are the presence of left bundle branch block (LBBB) or a paced ventricular rhythm. Another unique application of vasodilator stress testing is very early risk stratification of stable patients with AMI. In the Adenosine Sestamibi SPECT Post-Infarction Evaluation (INSPIRE) study, which enrolled patients with both ST- and non-ST-segment elevation AMI, vasodilator stress imaging was safely performed as early as 12 hours after presentation.48
Three pharmacologic agents are currently FDA approved in the United States: dipyridamole, adenosine, and regadenoson. These agents demonstrate similar diagnostic accuracy.49 Adenosine is a direct nonselective adenosine receptor agonist. The major advantage of adenosine over dipyridamole and regadenoson is its very short half-life (<10 s). For this reason, adenosine is the preferred agent in patients who are at greatest risk for developing side effects (e.g., a patient with borderline low blood pressure). Dipyridamole is an indirect coronary artery vasodilator. It blocks the intracellular reuptake and deamination of adenosine and thereby boosts adenosine levels in the intravascular space. Intravenous dipyridamole can be safely administered to a patient taking oral dipyridamole. Regadenoson is the first selective adenosine A2A receptor agonist to be approved for pharmacologic stress testing. Its affinity for the adenosine A1 receptor is at least 10-fold lower than for the A2 receptor, minimizing the risk for AV block. In the phase 3 Adenoscan Versus Regadenoson Comparative Evaluation for MPI (ADVANCE MPI) 2 trials, regadenoson had a lower composite side effect profile than did adenosine but with comparable imaging results.49 The half-life of regadenoson is triphasic, and aminophylline can be used to treat side effects. Physiologic effects of this medication can persist for up to 30 minutes after administration. Regadenoson is logistically easier to administer than adenosine or dipyridamole because it is given as an intravenous bolus injection over 10 s without the need for an infusion pump.
Dobutamine is the most commonly used synthetic catecholamine for pharmacologic stress testing in the United States. The half-life of dobutamine is approximately 2 minutes, but its physiologic effect may persist for 5 to 20 minutes and may require the administration of a short-acting beta-blocker for reversal. Given the variable physiologic response to dobutamine in individual patients and the more labor-intensive requirements of administering this agent, dobutamine is designated as a secondary pharmacologic stress agent in nuclear cardiology. Its use is reserved for patients who cannot adequately exercise and who have a contraindication to performing vasodilator stress testing.
The administration of anti-ischemic medications (i.e., nitrates, beta blockers, and calcium channel antagonists) either alone or in combination can reduce the diagnostic sensitivity of stress SPECT or PET MPI, irrespective of the stressor modality utilized.50
Over the past few years, major cardiac societies such as the American College of Cardiology/American Heart Association in collaboration with many other imaging societies have taken proactive steps to guide the use of cardiac imaging. Appropriate use criteria for nuclear cardiology have been published to ensure that clinicians in most instances are "doing the right test for the right patient at the right time."51 The recent comprehensive ASNC preferred practice statement "Patient Centered Imaging" by Depuey et al.44 outlines various MPI protocols with existing isotopes and their advantages and disadvantages and best use in daily clinical practice. This document reiterates an important point that physicians practicing nuclear cardiology should embrace: "One size does not fit all."
An appropriate study, by definition, provides incremental information, combined with clinical judgment, which exceeds the expected negative consequences, such as the risk of ionizing radiation from the procedure. Thus, when appropriately utilized, the clinical benefits of MPI far outweigh the lifetime potential risk of ionizing radiation exposure.
In patients with inappropriate indications for MPI (Table 3), the estimated life-attributable risk of cancer from radiation exposure, although minimal, should still be considered to be unacceptable. Hence, the discussion regarding the use of low-level ionizing radiation for MPI should focus on the appropriate selection of patients and the application of the As Low As Reasonably Achievable (ALARA) principle for the administration of a radiotracer to obtain high-quality, clinically interpretable images.52-54
In conclusion, these past few years have brought several rapid developments that have increased photon sensitivity in nuclear cardiology scanner hardware. Additionally, software applying new methods of SPECT reconstruction on conventional and dedicated systems has preserved or even improved SPECT image quality with lower count statistics. Several new dedicated camera systems with optimized acquisition geometry and collimator design resulting in increased count sensitivity have been introduced by vendors. Although it is convenient for patients to have imaging completed in 2 to 4 min, the efficiency of these systems provides the opportunity to reduce both the duration of the acquisition and the radiation dose to the patient.
MPI protocols should be optimized to achieve the lowest possible radiation exposure while maintaining diagnostic performance. Key aspects of this optimization include the use of radionuclides with shorter half-lives (such as Tc-99m and PET tracers), stress-only imaging when feasible, and weight-based dosing. Supporting research and development of camera technology, image reconstruction and acquisition techniques, and approaches to collimation are crucial to continued progress in the field of nuclear cardiology.
Figures and Tables
FIG. 1
D-SPECT cardiac system and detector-head configuration. (A) D-SPECT cardiac scanner with seated patient. (B) Each detector column is composed of CZT sensor (39×39×5 mm) with four 16×16 pixel detectors and tungsten collimator with 0.2-mm septa and square opening (pitch, 2.46 mm; length, 21.7 mm). (C) Nine detector columns, each capable of rotation and translation, are used to scan the myocardium. From Ref. 3.
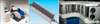
FIG. 2
Case example of MPI of a patient with 70% stenosis of the proximal midleft anterior descending coronary artery. (A) Perfusion images by conventional (top) and high-speed (bottom) SPECT demonstrating a moderate reversible perfusion defect in the left anterior descending coronary artery territory. Total acquisition time is indicated on the left-hand side. (B) Quantitative analysis yielded similar results for high-speed and conventional SPECT. From Ref. 5.
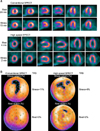
FIG. 3
Proposed algorithm for maximal reduction in patient radiation exposure is modified from ref. 54.
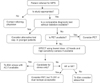
TABLE 2
SPECT myocardial perfusion imaging protocols and patient radiation dose
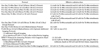
Modified from Ref. 44.
References
1. Health Quality Ontario. Single photon emission computed tomography for the diagnosis of coronary artery disease: an evidence-based analysis. Ont Health Technol Assess Ser. 2010; 10:1–64.
2. 2006 image of the year: focus on cardiac SPECT/CT. J Nucl Med. 2006; 47:14N–15N.
3. Gambhir SS, Berman DS, Ziffer J, Nagler M, Sandler M, Patton J, et al. A novel high-sensitivity rapid-acquisition single-photon cardiac imaging camera. J Nucl Med. 2009; 50:635–643.


4. Garcia EV, Faber TL, Esteves FP. Cardiac dedicated ultrafast SPECT cameras: new designs and clinical implications. J Nucl Med. 2011; 52:210–217.


5. Sharir T, Slomka PJ, Hayes SW, DiCarli MF, Ziffer JA, Martin WH, et al. Multicenter trial of high-speed versus conventional single-photon emission computed tomography imaging: quantitative results of myocardial perfusion and left ventricular function. J Am Coll Cardiol. 2010; 55:1965–1974.


6. Sharir T, Ben-Haim S, Merzon K, Prochorov V, Dickman D, Ben-Haim S, et al. High-speed myocardial perfusion imaging initial clinical comparison with conventional dual detector anger camera imaging. JACC Cardiovasc Imaging. 2008; 1:156–163.
7. Herzog BA, Buechel RR, Katz R, Brueckner M, Husmann L, Burger IA, et al. Nuclear myocardial perfusion imaging with a cadmium-zinc-telluride detector technique: optimized protocol for scan time reduction. J Nucl Med. 2010; 51:46–51.


8. Buechel RR, Herzog BA, Husmann L, Burger IA, Pazhenkottil AP, Treyer V, et al. Ultrafast nuclear myocardial perfusion imaging on a new gamma camera with semiconductor detector technique: first clinical validation. Eur J Nucl Med Mol Imaging. 2010; 37:773–778.


9. Esteves FP, Raggi P, Folks RD, Keidar Z, Askew JW, Rispler S, et al. Novel solid-state-detector dedicated cardiac camera for fast myocardial perfusion imaging: multicenter comparison with standard dual detector cameras. J Nucl Cardiol. 2009; 16:927–934.


10. Nakazato R, Berman DS, Hayes SW, Fish M, Padgett R, Xu Y, et al. Myocardial perfusion imaging with a solid-state camera: simulation of a very low dose imaging protocol. J Nucl Med. 2013; 54:373–379.


11. Garcia EV. SPECT attenuation correction: an essential tool to realize nuclear cardiology's manifest destiny. J Nucl Cardiol. 2007; 14:16–24.


12. Araujo LI, Jimenez-Hoyuela JM, McClellan JR, Lin E, Viggiano J, Alavi A. Improved uniformity in tomographic myocardial perfusion imaging with attenuation correction and enhanced acquisition and processing. J Nucl Med. 2000; 41:1139–1144.
13. Slomka PJ, Berman DS, Germano G. Applications and software techniques for integrated cardiac multimodality imaging. Expert Rev Cardiovasc Ther. 2008; 6:27–41.


14. Einstein AJ, Henzlova MJ, Rajagopalan S. Estimating risk of cancer associated with radiation exposure from 64-slice computed tomography coronary angiography. JAMA. 2007; 298:317–323.


15. Schaap J, Kauling RM, Boekholdt SM, Nieman K, Meijboom WB, Post MC, et al. Incremental diagnostic accuracy of hybrid SPECT/CT coronary angiography in a population with an intermediate to high pre-test likelihood of coronary artery disease. Eur Heart J Cardiovasc Imaging. 2013; 14:642–649.


16. Di Carli MF, Dorbala S, Meserve J, El Fakhri G, Sitek A, Moore SC. Clinical myocardial perfusion PET/CT. J Nucl Med. 2007; 48:783–793.


17. DePuey EG, Gadiraju R, Clark J, Thompson L, Anstett F, Shwartz SC. Ordered subset expectation maximization and wide beam reconstruction "half-time" gated myocardial perfusion SPECT functional imaging: a comparison to "full-time" filtered backprojection. J Nucl Cardiol. 2008; 15:547–563.


18. Borges-Neto S, Pagnanelli RA, Shaw LK, Honeycutt E, Shwartz SC, Adams GL, et al. Clinical results of a novel wide beam reconstruction method for shortening scan time of Tc-99m cardiac SPECT perfusion studies. J Nucl Cardiol. 2007; 14:555–565.


19. Venero CV, Heller GV, Bateman TM, McGhie AI, Ahlberg AW, Katten D, et al. A multicenter evaluation of a new post-processing method with depth-dependent collimator resolution applied to full-time and half-time acquisitions without and with simultaneously acquired attenuation correction. J Nucl Cardiol. 2009; 16:714–725.


20. Maddahi J, Mendez R, Mahmarian JJ, Thomas G, Babla H, Bai C, et al. Prospective multicenter evaluation of rapid, gated SPECT myocardial perfusion upright imaging. J Nucl Cardiol. 2009; 16:351–357.


21. Druz RS, Phillips LM, Chugkowski M, Boutis L, Rutkin B, Katz S. Wide-beam reconstruction half-time SPECT improves diagnostic certainty and preserves normalcy and accuracy: a quantitative perfusion analysis. J Nucl Cardiol. 2011; 18:52–61.


22. DePuey EG, Bommireddipalli S, Clark J, Leykekhman A, Thompson LB, Friedman M. A comparison of the image quality of full-time myocardial perfusion SPECT vs wide beam reconstruction half-time and half-dose SPECT. J Nucl Cardiol. 2011; 18:273–280.


23. DePuey EG, Ata P, Wray R, Friedman M. Very low-activity stress/high-activity rest, single-day myocardial perfusion SPECT with a conventional sodium iodide camera and wide beam reconstruction processing. J Nucl Cardiol. 2012; 19:931–944.


24. DePuey EG, Bommireddipalli S, Clark J, Thompson L, Srour Y. Wide beam reconstruction "quarter-time" gated myocardial perfusion SPECT functional imaging: a comparison to "full-time" ordered subset expectation maximum. J Nucl Cardiol. 2009; 16:736–752.


25. Burrell S, MacDonald A. Artifacts and pitfalls in myocardial perfusion imaging. J Nucl Med Technol. 2006; 34:193–211. quiz 212-4.
26. Garcia E, Maddahi J, Berman D, Waxman A. Space/time quantitation of thallium-201 myocardial scintigraphy. J Nucl Med. 1981; 22:309–317.
27. Chen J, Boogers MJ, Bax JJ, Soman P, Garcia EV. The use of nuclear imaging for cardiac resynchronization therapy. Curr Cardiol Rep. 2010; 12:185–191.


28. Boogers MJ, Chen J, van Bommel RJ, Borleffs CJ, Dibbets-Schneider P, van der Hiel B, et al. Optimal left ventricular lead position assessed with phase analysis on gated myocardial perfusion SPECT. Eur J Nucl Med Mol Imaging. 2011; 38:230–238.


29. Schindler TH, Quercioli A. Left ventricular dyssynchrony assessment by phase analysis from gated myocardial perfusion SPECT: moving beyond conventional criteria. Heart. 2011; 97:4–5.


30. Niu X, Yang Y, Jin M, Wernick MN, King MA. Effects of motion, attenuation, and scatter corrections on gated cardiac SPECT reconstruction. Med Phys. 2011; 38:6571–6584.


31. Lebowitz E, Greene MW, Fairchild R, Bradley-Moore PR, Atkins HL, Ansari AN, et al. Thallium-201 for medical use I. J Nucl Med. 1975; 16:151–155.
32. Nielsen AP, Morris KG, Murdock R, Bruno FP, Cobb FR. Linear relationship between the distribution of thallium-201 and blood flow in ischemic and nonischemic myocardium during exercise. Circulation. 1980; 61:797–801.


33. Beller GA, Watson DD, Ackell P, Pohost GM. Time course of thallium-201 redistribution after transient myocardial ischemia. Circulation. 1980; 61:791–797.


34. Dahlberg ST. Assessment of myocardial perfusion with Tc-99m: image is everything. J Nucl Cardiol. 2009; 16:493–496.


35. Liu Z, Chen L, Liu S, Barber C, Stevenson GD, Furenlid LR, et al. Kinetic characterization of a novel cationic (99m)Tc(I)-tricarbonyl complex, (99m)Tc-15C5-PNP, for myocardial perfusion imaging. J Nucl Cardiol. 2010; 17:858–867.


37. Agostini D, Verberne HJ, Burchert W, Knuuti J, Povinec P, Sambuceti G, et al. I-123-mIBG myocardial imaging for assessment of risk for a major cardiac event in heart failure patients: insights from a retrospective European multicenter study. Eur J Nucl Med Mol Imaging. 2008; 35:535–546.


39. Jacobson AF, Senior R, Cerqueira MD, Wong ND, Thomas GS, Lopez VA, et al. ADMIRE-HF Investigators. Myocardial iodine-123 meta-iodobenzylguanidine imaging and cardiac events in heart failure. Results of the prospective ADMIRE-HF (AdreView Myocardial Imaging for Risk Evaluation in Heart Failure) study. J Am Coll Cardiol. 2010; 55:2212–2221.


40. Shi CQ, Young LH, Daher E, DiBella EV, Liu YH, Heller EN, et al. Correlation of myocardial p-(123)I-iodophenylpentadecanoic acid retention with (18)F-FDG accumulation during experimental low-flow ischemia. J Nucl Med. 2002; 43:421–431.
42. Dilsizian V, Bateman TM, Bergmann SR, Des Prez R, Magram MY, Goodbody AE, et al. Metabolic imaging with beta-methylp-[(123)I]-iodophenyl-pentadecanoic acid identifies ischemic memory after demand ischemia. Circulation. 2005; 112:2169–2174.


43. Kawai Y, Tsukamoto E, Nozaki Y, Morita K, Sakurai M, Tamaki N. Significance of reduced uptake of iodinated fatty acid analogue for the evaluation of patients with acute chest pain. J Am Coll Cardiol. 2001; 38:1888–1894.


44. Depuey EG, Mahmarian JJ, Miller TD, Einstein AJ, Hansen CL, Holly TA, et al. Patient-centered imaging. J Nucl Cardiol. 2012; 19:185–215.


45. Cerqueira MD, Allman KC, Ficaro EP, Hansen CL, Nichols KJ, Thompson RC, et al. Recommendations for reducing radiation exposure in myocardial perfusion imaging. J Nucl Cardiol. 2010; 17:709–718.


46. Hlatky MA, Boineau RE, Higginbotham MB, Lee KL, Mark DB, Califf RM, et al. A brief self-administered questionnaire to determine functional capacity (the Duke Activity Status Index). Am J Cardiol. 1989; 64:651–654.


47. Kligfield P, Lauer MS. Exercise electrocardiogram testing: beyond the ST segment. Circulation. 2006; 114:2070–2082.
48. Mahmarian JJ, Shaw LJ, Filipchuk NG, Dakik HA, Iskander SS, Ruddy TD, et al. INSPIRE Investigators. A multinational study to establish the value of early adenosine technetium-99m sestamibi myocardial perfusion imaging in identifying a low-risk group for early hospital discharge after acute myocardial infarction. J Am Coll Cardiol. 2006; 48:2448–2457.


49. Cerqueira MD, Nguyen P, Staehr P, Underwood SR, Iskandrian AE. ADVANCE-MPI Trial Investigators. Effects of age, gender, obesity, and diabetes on the efficacy and safety of the selective A2A agonist regadenoson versus adenosine in myocardial perfusion imaging integrated ADVANCE-MPI trial results. JACC Cardiovasc Imaging. 2008; 1:307–316.


50. Mahmarian JJ. Monitoring medical therapy: the role of noninvasive imaging. In : Dilsizian V, Narula J, Braunwald E, editors. Atlas of nuclear cardiology. 2nd ed. Philadelphia, PA: Current Medicine;2006. p. 191–210.
51. Hendel RC, Berman DS, Di Carli MF, Heidenreich PA, Henkin RE, Pellikka PA, et al. American College of Cardiology Foundation Appropriate Use Criteria Task Force. American Society of Nuclear Cardiology. American College of Radiology. American Heart Association. American Society of Echocardiology. Society of Cardiovascular Computed Tomography. Society for Cardiovascular Magnetic Resonance. Society of Nuclear Medicine. ACCF/ASNC/ACR/AHA/ASE/SCCT/SCMR/SNM 2009 appropriate use criteria for cardiac radionuclide imaging: a report of the American college of cardiology foundation appropriate use criteria task force, the American society of nuclear cardiology, the American college of radiology, the American heart association, the American society of echocardiography, the society of cardiovascular computed tomography, the society for cardiovascular magnetic resonance, and the society of nuclear medicine. J Am Coll Cardiol. 2009; 53:2201–2229.
52. Shaw LJ, Achenbach S, Chandrashekhar Y, Dilsizian V, Hundley WG, Kern MJ, et al. Imaging modalities and radiation: benefit has its risks. JACC Cardiovasc Imaging. 2010; 3:550–552.

