Abstract
The aquaporin (AQP) families of water channels are intrinsic membrane proteins that facilitate selective water and small solute movement across the plasma membrane. The purposes of this study were to determine the expression and localization of AQPs in benign prostatic hyperplasia and prostate cancer. Prostatic tissue was collected from patients with benign prostatic hyperplasia or prostate cancer by transurethral resection of the prostate. The expression and cellular localization of the AQPs were determined in the human prostate by Western blot and immunohistochemistry. AQP1, 3, and 9 were expressed in the human prostate. Western blot analysis revealed bands at 28-36 kDa for the AQP1, 3, and 9 proteins. Of these proteins, AQP3 and 9 were expressed in the epithelium. Immunolabeling showed that AQP1 was mainly expressed in the capillaries and venules of the prostate, AQP9 was expressed in the cytoplasm of the epithelium, and AQP3 was mainly associated with the plasma membrane of the prostatic epithelium. Only AQP3 expression was localized in the cell membrane, and expressed AQP3 was translocated to the cytoplasm in prostate cancer. The epithelium in the human prostate expresses AQP3 and 9 proteins, and the capillaries and venules of the prostate express AQP1. Characterizing or modifying the expression of AQP3 may lead to an understanding of the role of the AQPs in human prostatic disease.
Aquaporins (AQPs) are a family of small (approximately 30 kDa per monomer) membrane transport proteins that form aqueous channels through the lipid bilayer and that facilitate osmotically driven water transport across cell plasma membranes.1 To date, 13 AQP isoforms (AQPs 0-12) have been identified; 11 isoforms are expressed in the male and female reproductive systems.2,3 A subset of the AQP family, the aquaglyceroporins (AQP3, AQP7, AQP9), transport both water and glycerol. Studies, largely from knockout mice, have implicated the AQPs in many expected physiological functions, including urine concentration and exocrine gland secretion, as well as in several unanticipated functions, including brain swelling, neural signal transduction, skin moisturization, and fat metabolism.4,5 Compelling new evidence has supported the involvement of the AQPs in cell migration and proliferation, thus adding AQPs to an expanding list of effectors in tumor biology.6 Recently, the participation of AQPs during apoptosis has been studied.7,8
A considerable amount of work has been initiated during the past decade to elucidate the function of the AQPs in the male reproductive tract.9 However, the function and role of the AQPs have not yet been determined. Wang et al.10 investigated the expression of AQP3 in normal and cancerous prostate cells in vitro and in vivo. AQP3 protein was expressed at the membrane of normal prostate cells, whereas expression was observed at the cytoplasm of prostate cancer cells. Transporters should be expressed at the membrane to function. Therefore, AQP3 does not seem to play an important role in the growth of prostate cancer cells. Wang et al.10 mentioned the importance of AQPs, which function as selective pores to allow water, glycerol, and other small solutes to pass through the cell membrane. However, why those authors chose to examine AQP3 is unclear, and they could not adequately explain the role of the AQPs. The other AQPs should be examined in order to ascertain whether AQPs play an important role in the pathogenesis of prostate cancer.
Huang et al.3 reported that three AQPs (AQP1, 3, and 9) are expressed in mammalian prostate tissue. In the present study, we investigated the distribution of these AQPs in human benign prostatic hyperplasia (BPH) and the changes in AQP expression in cancer patients.
The study protocol was reviewed and approved by the institutional review board (HCRI 10 009-3) of Chonnam National University. Informed consent was obtained from all participants.
Prostate tissues were obtained for Western blot and immunohistochemistry by a transurethral resection of the prostate in 18 patients (age range, 54-80 years) who had been diagnosed with BPH (11 cases) or prostatic adenocarcinoma (Gleason score: 6 in one case, 7 in one case, and 8-10 in five cases) at Chonnam National University Hospital, Korea, between 2010 and 2011. The patients were divided into two groups: Group 1 (BPH group) and Group 2 (prostate cancer group). Patients who received hormonal treatment or any drugs that could affect sex steroid hormone levels were excluded from Group 1. In Group 2, grading of the primary tumor from each specimen was carried out by using serial sections stained with hematoxylin and eosin according to the Gleason system.
Tissue homogenates (20 µg protein) were separated by 10% sodium dodecyl sulfate-polyacrylamide gel electrophoresis, and the resolved proteins were transferred to a polyvinylidene difluoride membrane (Amersham Pharmacia Biotech, Buckinghamshire, UK). After the blots were washed with TBS-T (10 mM Tris-HCl [pH 7.6], 150 mM NaCl, and 0.05% Tween-20), the membranes were blocked with 5% skim milk for 1 h and incubated with the appropriate primary antibody. Polyclonal rabbit antibodies against AQP1, 3, and 9 (rabbit anti-AQP1, anti-AQP3, and anti-AQP9; Santa Cruz Biotechnology, Santa Cruz, CA, USA; all at a dilution of 1:1,000), and polyclonal rabbit antibody against glyceraldehyde 3-phosphate dehydrogenase (GAPDH; rabbit anti-GAPDH; Cell Signaling Technology, Danvers, MA, USA; 1:5,000) were used. The membranes were then washed, primary antibodies were detected with goat anti-rabbit IgG conjugated to horseradish peroxidase, and the bands were visualized by enhanced chemiluminescence (Amersham Pharmacia Biotech, Franklin Lakes, NJ, USA). GAPDH was used as an internal control. Separate blots from separate gels were used for the different blots.
An immunofluorescent study was performed on the prostate. For cryostat sections, tissues from the ventral prostate were fixed in 4% paraformaldehyde (4℃ for 12 h) before dehydration in 30% graded sucrose solutions (4℃ for 12 h), embedded in OCT compound (Sakura Finetek USA, Torrance, CA, USA), and frozen in liquid nitrogen. Following fixation, preparations were washed for 10 min in phosphate-buffered saline (0.01 M, pH 7.4). The tissues were then incubated in 10% chicken serum containing 0.3% Triton X-100 for 1 h at room temperature to reduce nonspecific antibody binding. For examination of AQPs, tissues were incubated overnight at 4℃ with antibody against AQP1 (Chemicon International, Temecula, CA, USA) and AQP9 (Santa Cruz Biotechnology). Immunoreactivity for AQPs was detected by using Alexa Fluor 488 chicken anti-rabbit IgG (H+L; Molecular Probes, Eugene, OR, USA). Tissues were mounted with mounting solution containing 4'-6-diamidino-2-phenylindole (DAPI; Vector Laboratories, Burlingame, CA, USA). Tissues were examined with an LSM 510 confocal microscope (Carl Zeiss, Jena, Germany) with an excitation wavelength appropriate for Alexa Fluor (488 nm). The confocal micrographs were digital composites of z-series scans of 10 to 30 optical sections through a depth of 10 to 30 µm.
AQP1 immunoreactivity was expressed in the subepithelial area of the prostate, and AQP3 and AQP9 were expressed in the prostate epithelium of three patients with BPH. Western blot analysis revealed that the bands at 28-36 kDa represented the nonglycosylated form of the AQPs (Fig. 1).
The immunolabeling study showed a distinct localization of AQP1, AQP3, and AQP9 with various cellular distribution patterns in the prostate of BPH patients (Fig. 2).
AQP1 was mainly expressed in capillaries and venules of the prostate. Immunolabeling showed that AQP1 was expressed along the vascular endothelial cells in the subepithelial area, including the small capillaries just beneath the subepithelial lamina propria (Fig. 2A).
AQP3 and AQP9 were expressed in the epithelial area of the prostate. AQP9 was expressed in the cytoplasm of the epithelial cells, and AQP3 was expressed in the plasma membrane of individual cells of the prostatic epithelium (Fig. 2B, C).
Immunolabeling revealed distinct localization of AQP1 and AQP3 with various cellular distribution patterns in prostate cancer patients (Fig. 3).
AQP1 was mainly expressed in capillaries and venules of the prostate. Immunolabeling showed that AQP1 was expressed along the vascular endothelial cells, including the small capillaries just beneath the subepithelial lamina propria (Fig. 3A).
AQP3 was expressed in the cytoplasm of the epithelial cells (Fig. 3B).
The transport of water in the male reproductive tract is essential for maintaining the fluid environment. AQPs are important in controlling water permeability. The present results demonstrate the presence and immunolocalization of AQP1, 3, and 9 in the human prostate. Immunolabeling revealed AQP1 in the subepithelial areas of the capillaries and venules of the prostate. In contrast, AQP3 and AQP9 were expressed in the prostatic epithelium.
Our findings of the distinct localization of AQP expression in the subepithelial (AQP1) and epithelial (AQP3 and AQP9) layers of the prostate may be meaningful. Within the epithelium, regional differences were found in the location of the AQPs (e.g., cytoplasm or plasma membrane). AQP9 was expressed within the epithelial cell cytoplasm, whereas AQP3 was expressed on the plasma membrane of prostatic epithelial cells. These findings may imply that AQPs have different functional roles depending on their type and cellular location. The AQP water channels are suggested to play functional roles in prostatic secretion. The specific functional role of each subtype of AQP should be clarified.
Mobasheri et al.11 described the expression of AQP3 in the human prostate, and AQP3 is also known to be expressed in various organs.12 In the present study, AQP3 seemed to be positioned circumferentially in the epithelial cell membrane, except on the luminal surface. The expression of AQP3 on the basolateral plasma membrane of secretory epithelial cells would serve to rapidly transport water from the cell into the intertubular space. In fact, AQP3 can also transport small molecules, such as glycerol and urea. As a result, AQP3 may act to allow urea to enter into prostatic fluid.13,14
An alteration in AQP expression results in a variety of pathological states.15 In the present study, AQP3 protein expression was detected in human prostate cancer. However, AQP3 immunoreactivity was not detected on cancer cell membranes of the prostate. The immunofluorescence staining in this study indicates that AQP3 dislocation may be involved in tumor initiation. This may result from an abundant replication or long-term survival of tumor cells without apoptosis. During the apoptotic process, the cell volume needs to decrease rapidly by apoptotic volume decrease (AVD). The AVD process has been correlated with the membrane expression of AQP.7,8 Tumor cells may need membrane expressing AQP3 to facilitate apoptosis. The change in the expression of AQP3 from the cell membrane to the cytoplasm may bestow advantages for survival.
Wang et al.10 showed that AQP3 is expressed in the cytoplasm of prostate cancer cells. However, transporter proteins need to be expressed in the plasma membrane to function. Ismail et al.16 investigated the expression and localization of AQP3 in prostate cancer cells in response to cryoinjury by use of immunofluorescence staining. Cryotherapy markedly redistributed AQP3 protein into the plasma membrane of prostate cancer cells. Ismail et al.16 also showed that cryoinjury results in the rearrangement of AQP3 from the cytoplasm to the plasma membrane, which may reflect the direct involvement of AQP3 in the intracellular osmotic changes associated with cryoinjury. These changes may make prostate cancer cells more vulnerable to the apoptotic process.
Further studies are required to elucidate the precise roles of AQP3 in apoptosis in prostate cells. Jung et al.17 provided important clues to the possible role of AQPs by demonstrating the localization of AQP1 and AQP9 in rat ventral prostate. Their immunolabeling showed that AQP1 was mainly expressed in the capillaries and venules, AQP9 was expressed in the cytoplasm and cell membrane of the individual cells of the rat prostatic epithelium, and AQP9 was mainly associated with the plasma membrane of the individual cells of the intermediate epithelium with secretory function, which is consistent with the present finding of AQP3 expression in the human prostate. The expression was not changed in subepithelial cells expressing AQP1 after castration. However, the membrane expression of AQP9 was decreased after castration. This result is similar to our human prostate study (rat ventral prostate AQP1 vs. human prostate AQP1, and rat ventral prostate AQP9 vs. human prostate AQP3). The prior observations17 provided important clues about the possible role of AQPs by demonstrating the correlation of AQP9 and apoptosis in the rat ventral prostate; when membrane expression of AQP was preserved, maximal apoptosis developed. Jablonski et al.7,8 reported that water movement during AVD in thymocytes, granulosa cells, and hepatocytes is mediated primarily by AQPs, which directly affect the rate of apoptotic progression. In our prostate cancer patients, AQP expressed on the membrane was translocated to the cytoplasm. This may explain the ability of prostate cancer cells to escape apoptosis.
Water-channel protein may be involved in the preservation of cellular character in the human prostate, and prostate cancer may be associated with an alteration of water-transporting mechanisms. Changes in the localization of AQP3 in cancer cells may result from tumorigenesis or may facilitate tumorigenesis. Thus, the role of AQP in prostate cancer could be an apoptotic role rather than a participation in proliferation. Other functional roles of AQPs in the prostate could include water movement during AVD, although this remains conjecture pending further studies.
The present study showed that the normal epithelium in the human prostate expresses AQP1, 3, and 9 proteins. Characterizing or modifying the expression of the AQPs may lead to an understanding of their role in human prostatic disease.
A limitation of our study was the lack of comparison between normal prostate tissue and pathologic tissue. It was not possible to obtain normal prostate tissue in this study. Further evaluation with prostate tissue of young male adults is needed. Also, we did not analyze the expression of AQP9 in prostate cancer in this preliminary study.
In conclusion, our finding of the distinct localization of AQP1, 3, and 9 in the human prostate implies the selective involvement of the AQPs in prostate secretion. Among the AQPs, only AQP3 is expressed in the epithelial cell membrane and is changed in prostate cancer. The clinical implications of specific AQP localization in human prostatic tissue and the functional roles of these AQPs must be clarified in future studies.
Figures and Tables
FIG. 1
Western blot analysis of AQP expression in benign prostatic hyperplasia. Anti-AQP antibody recognized bands at 28 to 36 kD, which corresponded to AQP1, 3, and 9 protein (AQP1: 28 kDa, AQP3: 36 kDa, AQP9: 29 kDa).
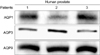
FIG. 2
Representative immunofluorescence confocal microscopic images of AQP1 (A), AQP3 (B), and AQP9 (C) in benign prostatic hyperplasia. (A) Images of AQP1 localization in the prostate. AQP1 was detected along the endothelium of the capillaries and venules of the prostate (arrow). (B) Images of AQP3 localization in the prostates. AQP3 was evident in the plasma membrane of the epithelium of the prostate (arrow). (C) Images of AQP9 localization in the prostate. AQP9 was observed in the cytoplasm of the epithelium of the prostate (arrow).
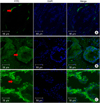
FIG. 3
Representative immunofluorescence confocal microscopic images of AQP1 (A) and AQP3 (B) in prostate cancer. (A) Immunofluorescence confocal microscopic images of AQP1 localization in prostate cancer. AQP1 was detected along the endothelium of the capillaries and venules of the prostate (arrow). (B) Immunofluorescence confocal microscopic images of AQP3 localization in the prostate cancer. AQP3 was observed in the cytoplasm of the epithelium of the prostate (arrow).
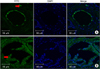
ACKNOWLEDGEMENTS
This study was supported by grant CRI 11-043-1 of Chonnam National University Hospital Research Institute of Clinical Medicine.
References
1. Verkman AS, Mitra AK. Structure and function of aquaporin water channels. Am J Physiol Renal Physiol. 2000. 278:F13–F28.


2. Zardoya R. Phylogeny and evolution of the major intrinsic protein family. Biol Cell. 2005. 97:397–414.


3. Huang HF, He RH, Sun CC, Zhang Y, Meng QX, Ma YY. Function of aquaporins in female and male reproductive systems. Hum Reprod Update. 2006. 12:785–795.


4. Agre P, Kozono D. Aquaporin water channels: molecular mechanisms for human diseases. FEBS Lett. 2003. 555:72–78.
5. Verkman AS. More than just water channels: unexpected cellular roles of aquaporins. J Cell Sci. 2005. 118:3225–3232.


6. Verkman AS, Hara-Chikuma M, Papadopoulos MC. Aquaporins-new players in cancer biology. J Mol Med (Berl). 2008. 86:523–529.


7. Jablonski EM, Webb AN, McConnell NA, Riley MC, Hughes FM Jr. Plasma membrane aquaporin activity can affect the rate of apoptosis but is inhibited after apoptotic volume decrease. Am J Physiol Cell Physiol. 2004. 286:C975–C985.


8. Jablonski EM, Mattocks MA, Sokolov E, Koniaris LG, Hughes FM Jr, Fausto N, et al. Decreased aquaporin expression leads to increased resistance to apoptosis in hepatocellular carcinoma. Cancer Lett. 2007. 250:36–46.


9. Cho YS, Svelto M, Calamita G. Possible functional implications of aquaporin water channels in reproductive physiology and medically assisted procreation. Cell Mol Biol (Noisy-le-grand). 2003. 49:515–519.
10. Wang J, Tanji N, Kikugawa T, Shudou M, Song X, Yokoyama M. Expression of aquaporin 3 in the human prostate. Int J Urol. 2007. 14:1088–1092.


11. Mobasheri A, Wray S, Marples D. Distribution of AQP2 and AQP3 water channels in human tissue microarrays. J Mol Histol. 2005. 36:1–14.


12. Nielsen S, Kwon TH, Christensen BM, Promeneur D, Frøkiaer J, Marples D. Physiology and pathophysiology of renal aquaporins. J Am Soc Nephrol. 1999. 10:647–663.


13. Ishibashi K, Sasaki S, Fushimi K, Uchida S, Kuwahara M, Saito H, et al. Molecular cloning and expression of a member of the aquaporin family with permeability to glycerol and urea in addition to water expressed at the basolateral membrane of kidney collecting duct cells. Proc Natl Acad Sci U S A. 1994. 91:6269–6273.


14. Fulmer BR, Turner TT. A blood-prostate barrier restricts cell and molecular movement across the rat ventral prostate epithelium. J Urol. 2000. 163:1591–1594.


15. Schrier RW, Cadnapaphornchai MA. Renal aquaporin water channels: from molecules to human disease. Prog Biophys Mol Biol. 2003. 81:117–131.

