Abstract
Apoptosis after global or focal cerebral ischemia plays a crucial role in mediating cell death. In this study, we observed the time point expression of physiologic events involving apoptosis regulatory proteins after photochemically-induced focal cerebral ischemia in Sprague-Dawley rats. Protein expression was evaluated at days 1, 3, and 7 by Western blot. Bcl-2, Bax, caspase-3, and phosphorylated Akt (pAkt) activity markedly increased in the ischemic hemisphere in a time-dependent manner, not affected. The expression of Bcl-2, Bax, and caspase-3 was dramatically changed around day 3, whereas changes in pAkt expression occurred at day 1. Differential elevation of these apoptosis regulatory proteins at various time points indicates that different modes of cell death occur in photochemically-induced focal cerebral ischemia in a rat brain.
Stroke is the major cause of chronic disability and the third leading cause of death in humans after cancer and coronary heart disease in developed countries. Because stroke causes severe neurological complications, new strategies for patients suffering from stroke are needed. Neuroprotection in ischemic stroke has been a topic of increasing concern and research. The capacity for neuroprotection depends on multiple factors including the use of an appropriate agent at the appropriate time and during the appropriate interval.1
A variety of animal models have been developed for modeling ischemic stroke. Among them, the most common model of focal ischemia in rats is the occlusion of the middle cerebral artery (MCAo) with an intraluminal suture, which mechanically occludes the major cerebral arteries.2 In contrast with other models of cerebral ischemia that involve mechanical occlusion of blood vessels, the rat photochemical cortical lesion models show endothelial damage, resulting in platelet aggregation, thrombosis, and permanent cerebral vascular occlusion.3 Considering that vascular thrombosis is responsible for more than 70% of human stroke cases and that only one Food and Drug Administration (FDA)-approved therapy exists for the treatment of acute ischemic stroke (the thrombolytic tissue plasminogen activator, or tPA), this model may be useful for understanding the pathobiology of ischemic stroke.4
Cerebral ischemia/reperfusion (I/R) injury triggers multiple and distinct but overlapping cell signaling pathways that may lead to cell survival or cell damage.5 Compared with global ischemia, focal ischemia is a reduction in blood flow to a very specific brain region as in embolic occlusion of the specific vessels.6 There are also significant differences in the modes of cell death between global and focal cerebral ischemia. In focal cerebral ischemia, most of the cells in the ischemic core undergo necrosis, but the rim of brain tissue that is hypoperfused surrounding the core is called the ischemic penumbra, which has the capacity to recover if perfusion is improved.7
In the penumbra, multiple mechanisms lead to apoptosis: excitotoxicity and ionic imbalance, oxidative stress, and apoptotic-like cell death may all occur and in both caspase-dependent and caspase-independent manners that may contribute to delayed ischemic cell death.8 A quite simplistic view implies poor prospects regarding cell survival in the core of the cerebral infarction and therapeutic expectations to control cell death and cell survival in the penumbra. In fact, focal cerebral ischemia triggers a cascade of molecular events that produce neuronal death ranging from immediate death to that occurring many days later, and this phenomenon raises the hypothesis that a "therapeutic window" exists during which interventions may improve neurological outcome.9 The aim of neuroprotection is to prevent delayed neuron apoptosis in the zone of the ischemic penumbra. On the basis of our current knowledge, many experimental trials have been reported to restore this process.10-12
In the photochemical stroke model, a ring lesion, which creates a cortical region of viable tissue surrounded by a circular rim of damaged tissue, has been described for the study of histological, biochemical, and molecular changes associated with the ischemic penumbra.13 TUNEL stain is used to demonstrate apoptotic and nonapoptotic features.14
Although the expression of pro- and anti-apoptotic factors and apoptotic cell death sequences have been extensively investigated, the time point changes in the mechanisms of cell death in photochemically induced focal cerebral ischemia remain inadequately defined. Previous investigations have reported on changes in the bcl-2 family in this model.14-16 Therefore, we examined changes in the expression of Bcl-2, Bax, caspase-3, phosphorylated Akt (pAkt), and survivin by Western blot 1, 3, and 7 days after photochemically induced focal cerebral ischemia in the rat.
All surgical procedures and postoperative care were performed in accordance with the guidelines of the Chonnam National University Animal Care and Usage Committee. Twenty male Sprague-Dawley rats weighing between 200 and 250 g were used in this study. These animals were maintained on a 12-h light/dark cycle and were allowed free access to food and water. Each rat was anesthetized with 5% isoflurane and was maintained with 2% isoflurane in an oxygen/air mixture with the use of a gas anesthesia mask in a stereotaxic frame (Stoelting, Wood Dale, IL, USA). Focal cortical ischemia was induced by photothrombosis of the cortical microvessels using Rose Bengal (RB; Sigma Chemical Co., St. Louis, MO, USA) with cold light (Zeiss KL1500 LCD, Jena, Germany) as previously described with minor modifications.3,17 The expression of each apoptosis associated protein was measured 1, 3, and 7 days after the onset of ischemia. Body temperature was maintained during surgery at 37±0.5℃ with a heating pad controlled with a rectal probe. For illumination, a 4.5-mm fiber optic bundle from a cold light source was positioned on the exposed skull 0.5 mm anterior to the bregma and 3.7 mm lateral to the midline over the left sensorimotor cortex. The brain was illuminated for 10 min after infusion of 50 mg/kg of RB in normal saline into the right femoral vein via a microinjection pump within 1 min. The scalp was sutured and the rats were allowed to awake before being returned to their cages. The sham-operated control group received the same vein injection of RB and the same surgery, but without exposure to light; thus, no photochemical reaction was induced.3
After decapitation, rat brains from different reperfusion time points (sham and days 1, 3, and 7) were quickly removed (each group, n=5). The ipsilateral and contralateral cerebral cortex was dissected. Samples were flash-frozen with liquid nitrogen and were stored at -80℃ until analyzed. Brain tissues were homogenized and proteins were purified by using 50 mM Tris-Cl (pH 7.4), 150 mM NaCl, 1 mM EDTA, 60 mM n-Octyl-β-D-Glucopyranoside (OGP), and 1 mM phenylmethylsulfonyl fluoride (PMSF) supplemented with a protease inhibitor cocktail (Sigma). To prepare lysates, tissues were sonicated for 4 s for a total of five times and the homogenate was spun at 20,000×g for 20 min at 4℃ to remove insoluble material. Protein concentrations were determined with the BCA Protein Assay Reagent Kit (Pierce, USA) using bovine serum albumin (BSA) as the standard. Equal amounts of nuclear protein (20 µg) for Bcl-2, Bax, caspase-3, pAkt (serine-473), and survivin were separated on 6% to 12% SDS-polyacrylamide gels (USB Fueling Innovation, Cleveland, OH, USA). A PageRuler Plus Prestained Protein Ladder™ (Fermentas Life Sciences, Hanover, MD, USA) was used as a size reference. Proteins were transferred to pure nitrocellulose membranes (Bio-Rad, Richmond, CA, USA). The membranes were incubated in blocking buffer, 5% nonfat dry milk in TBST (200 mM Tris-HCl, pH 7.4, 500 mM NaCl, 0.2% Tween 20), at room temperature for 1 h with agitation. The membranes were then incubated with rabbit Bcl-2 polyclonal antibody (Santa Cruz, 1:2,000 in TBS with 0.2% Tween 20), rabbit Bax polyclonal antibody (Millipore, 1:3,000 in TBS with 0.2% Tween 20), mouse caspase-3 monoclonal antibody (Millipore, 1:1,000 in TBS with 0.2% Tween 20), polyclonal rabbit anti-phopho-Akt antibody (serine-472, Santa Cruz, 1:5,000 in TBS with 0.2% Tween 20), and mouse survivin monoclonal antibody (Santa Cruz, 1:1,000 in TBS with 0.2% Tween 20) overnight at 4℃. Following washes, the membranes were incubated with peroxidase-conjugated goat anti-mouse or anti-rabbit immunoglobulin (Santa Cruz Biotechnology, 1:8,000) in TBS with 0.2% Tween 20 for 1 h. After rinses in washing buffer, signal of bound antibodies was developed by an enhanced Immoblion Western chemiluminescent HRP substrate (Millipore, Bedford, MA, USA). Quantification of each band was performed by densitometry analysis (Scion, NIH software, Bethesda, MD, USA) of the protein signal using LAS 3000. Mouse anti-GAPDH monoclonal antibody conjugated with HRP (Sigma, 1:25,000) was detected on immunoblots as a loading control for protein quantitation.
To analyze the amount of the Bcl-2, Bax, caspase-3, pAkt, survivin, and GAPDH, we used a relative protein ratio to control, and quantitative data were expressed as means±SD. Differences between means were determined by one-way ANOVA followed by Dunnett's post hoc test for multiple comparisons. p<0.05 was considered statistically significant.
Ischemia caused changes in the expression of various apoptosis-associated proteins depending on the time of study (Fig. 1). Notably, the expression of Bax, caspase-3, and pAkt proteins was markedly increased in the ischemic hemisphere 1 day after the operation compared with that in the sham controls. The expression of Bcl-2 and survivin was slightly reduced at day 1 and then gradually increased by day 3. Bax expression, the bands at 21 kDa, reached peak levels at day 3 and gradually decreased by day 7. Caspase-3 expression, identified at 32 kDa, was abruptly increased from day 1 to day 3 compared with the sham control and decreased at day 7. pAkt was increased at day 1 but was slightly reduced from day 3. The Bcl-2 expression pattern was different at different time points. It was slightly reduced at day 1, but gradually increased from day 3, and was rising on day 7. However, the variation in expression of survivin, which is an inhibitor apoptosis protein (IAP), was insignificant compared with the others.
Changes in the density of each apoptosis-associated protein at each time point are shown in Fig. 2. Bcl-2 protein was increased 1.9-fold at day 3 (p<0.05 versus control) and increased 2.8-fold at day 7 (p<0.05 versus control) in the ischemic hemisphere. Bax and pAkt were increased 1.6-fold at day 3 and 1.8-fold at day 1, respectively (p<0.05 versus control). Caspase-3 was abruptly increased 8.4- to 9.9-fold from day 1 to day 3 (p<0.01 versus control), respectively, after the operation. No significant differences in survivin levels were found between sham controls and the experimental groups.
Focal and global cerebral ischemia in rats triggers apoptotic cell death within the ischemic lesion. In many previous reports,1,18 it was shown that new protein synthesis appears to be required for apoptosis. Comparison of human small cortical infarcts reveals identical histological patterns to photochemically induced rat brain lesions as well as naturally occurring human infarcts.19 Comparatively little is known about the apoptotic process in the photochemically induced model, so we evaluated the time point expression patterns of the major apoptosis regulatory proteins.
The Bcl-2 family of proto-oncogenes encode specific proteins such as Bcl-2, Bcl-XL, and Bax that regulate apoptosis. The anti-apoptotic effect of Bcl-2 occurs by prevention of cytochrome c release into the cytoplasm.5 Many studies have shown that over-expression of Bcl-2 can reduce ischemic brain injury in animal models of stroke.12,20 Activated Bax promotes cell death, unless it is bound by either Bcl-2 or Bcl-XL.21 However, the two molecules can act independently to exert their effects on cell death.5 In this study, we found strong induction of Bax protein 1 day after infarction in the ischemic hemisphere and it reached a peak on day 3. Compared with Bax expression, Bcl-2 protein expression was slightly reduced at day 1 and then abruptly increased at day 3 and was sustained at day 7. In a previous report using the same stroke model,15 Bax protein was in the cytoplasm of degenerating neurons in the center of the infarction between 4 h and 3 days after photothrombosis, and at the same time points, the levels of Bcl-2 and Bcl-XL proteins were markedly reduced in this region. These findings suggest that Bcl-2 and Bax protein expression switches around day 3 in this model. Interestingly, in the MCAo mouse model with preconditioning, Bcl-2 and Bcl-Xl were increased after sublethal forebrain ischemia but Bax remained unchanged.22 These differential expressions of the Bcl-2 family were associated with animal modeling, such as preparing of preconditioning, and reproducibility of lethal legion induction. The slight decrease in Bcl-2 expression within 1 day might also be attributed to postischemic protease activation.23
Caspases are cysteine proteases that are constitutively expressed as zymogens or pro-caspases and that are specifically activated during apoptotic stimuli.24 Several previous studies reported that the role of caspase-3 is as a key mediator of apoptosis in ischemic stroke.25 Briefly, upregulation of caspase-3 mRNA in rat brain occurs 1 h after the onset of focal ischemia, whereas caspase-3 and its cleavage products in mouse brain are activated during early reperfusion 2 h after MCAo. Ischemic damage could be reduced by caspase inhibitor treatment even 9 h after MCAo. We observed a significant increase in the cleaved active form of caspase-3 from day 1 of operation until day 3, when the maximal intensity was observed (Fig. 2C). Also, this increased caspase-3 expression was sustained at day 7. We did not observe any significant change in the contralateral hemisphere. These findings are in agreement with an extended treatment window for caspase inhibition after stroke.18
Akt is a subfamily of serine/threonine protein kinases and is activated via phosphatidylinositol-3 kinase (PI3K). After phosphorylation, Akt promotes cell survival and prevents apoptosis by inactivating several targets. In the MCAo model,26 phosphorylation of Akt (pAkt) was temporally accelerated at serine-473 in the ischemic cortex after transient focal cerebral ischemia and was then decreased by 24 h. However, in the ischemic core, pAkt was decreased only after ischemia. pAkt-positive cells were colocalized with NeuN-positive cells in the cortex, but pAkt-positive cells did not co-localize with TUNEL-positive cells. In this study, pAkt expression was increased about 2-fold at day 1 and was decreased by day 3 compared with the sham control. This change in pAkt expression could be explained by the propagation of the ischemic lesion. The infarct develops in the superficial cortex, whereas the higher-order branches of the larger patent vessels in the deeper cortical layers are occluded by tissue compression caused by the vasogenic edema associated with this procedure.27 Further, complete wedge-shaped coagulative necrosis was formed 24 h after ischemia according to histopathological examination.28 Thus, as in previously reported studies,26 pAkt expression in the photochemical-induced stroke model might also depend on the severity of the ischemia.
Survivin is a member of the IAP family that serves multiple functions related to both cell survival and cell division within many cell types and only recently has come under study in relation to ischemia in heart or brain.29 Recently, Zhang et al.30 reported that this protein is a target for the PI3-kinase/Akt pathway and can antagonize the effects of apoptosis-inducing factor. On the basis of current knowledge, we hypothesized that survivin would be significantly changed in our stroke model, but as noted, the changes in survivin expression evaluated by Western blot were insignificant. In fact, infarct volumes in survivin+/-. mice at 24 h and 3 d were not significantly different from those of survivin+/+ mice, which suggests that the role of survivin in neuroprotection is minimal.29
In this study, we evaluated the expression of apoptosis regulatory proteins in photochemically induced focal cerebral ischemic rat brain. To interpret these changes, we have to consider the changes in cerebral blood flow (CBF) after photothrombosis. In rats subjected to a photothrombotic stroke lesion, cortical CBF at the center of the region at risk was gradually reduced until 48 h after irradiation with flow values of 33%. However, a significant partial restoration of blood flow compared with cCBF values at 24 h was observed at 72 and 96 h after irradiation. Hence, cortical CBF was 56% of preischemic levels at 72 h and 87% at 96 h after ischemic induction. These CBF changes could explain the meaningful changes in Bcl-2, Bax, and caspase-3 around day 3.
In conclusion, targeting and preventing apoptosis in the penumbra seems to be a rational therapeutic goal for reducing cerebral infarct volume after clinical stroke, and the present study may provide the basis for the design of therapeutic interventions using the photochemical thrombosis stroke model. However, the expression of each apoptosis regulatory protein as well as the regional distribution and association with DNA fragmentation should be assessed further.
Figures and Tables
FIG. 1
Representative Western blots showing immunoreactivity of Bcl-2, Bax, caspase-3, pAkt, and survivin proteins in controls and in the ischemic hemisphere 1, 3, and 7 days after photochemically induced focal cerebral ischemia. All proteins were detected at the predicted molecular size and showed different time point expression patterns. The results of GAPDH analysis are shown as an internal control.
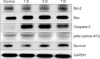
ACKNOWLEDGEMENTS
This survivin study was supported by a grant from the Chonnam National University (Lee MC, 2007-3328). The comprehensive study was supported by the Basic Science Research Program through the National Research Foundation of Korea (NRF) funded by the Ministry of Education, Science and Technology (Kim HS, 2009-0074 754).
References
1. Ferrer I. Apoptosis: future targets for neuroprotective strategies. Cerebrovasc Dis. 2006. 21:Suppl 2. 9–20.


2. Belayev L, Alonso OF, Busto R, Zhao W, Ginsberg MD. Middle cerebral artery occlusion in the rat by intraluminal suture. Neurological and pathological evaluation of an improved model. Stroke. 1996. 27:1616–1622.


3. Watson BD, Dietrich WD, Busto R, Wachtel MS, Ginsberg MD. Induction of reproducible brain infarction by photochemically initiated thrombosis. Ann Neurol. 1985. 17:497–504.


4. Feng R, Li S, Li F. Toll-like receptor 4 is involved in ischemic tolerance of postconditioning in hippocampus of tree shrews to thrombotic cerebral ischemia. Brain Res. 2011. 1384:118–127.


5. Nakka VP, Gusain A, Mehta SL, Raghubir R. Molecular mechanisms of apoptosis in cerebral ischemia: multiple neuroprotective opportunities. Mol Neurobiol. 2008. 37:7–38.


7. Ginsberg MD. Adventures in the pathophysiology of brain ischemia: penumbra, gene expression, neuroprotection: the 2002 Thomas Willis Lecture. Stroke. 2003. 34:214–223.


8. Ferrer I, Friguls B, Dalfó E, Justicia C, Planas AM. Caspase-dependent and caspase-independent signalling of apoptosis in the penumbra following middle cerebral artery occlusion in the adult rat. Neuropathol Appl Neurobiol. 2003. 29:472–481.


10. Wei Y, Yemisci M, Kim HH, Yung LM, Shin HK, Hwang SK, et al. Fingolimod provides long-term protection in rodent models of cerebral ischemia. Ann Neurol. 2011. 69:119–129.


11. Wang Q, Li X, Chen Y, Wang F, Yang Q, Chen S, et al. Activation of epsilon protein kinase C-mediated anti-apoptosis is involved in rapid tolerance induced by electroacupuncture pretreatment through cannabinoid receptor type 1. Stroke. 2011. 42:389–396.


12. Okazaki T, Magaki T, Takeda M, Kajiwara Y, Hanaya R, Sugiyama K, et al. Intravenous administration of bone marrow stromal cells increases survivin and Bcl-2 protein expression and improves sensorimotor function following ischemia in rats. Neurosci Lett. 2008. 430:109–114.


13. Hu X, Wester P, Brännström T, Watson BD, Gu W. Progressive and reproducible focal cortical ischemia with or without late spontaneous reperfusion generated by a ring-shaped, laser-driven photothrombotic lesion in rats. Brain Res Brain Res Protoc. 2001. 7:76–85.


14. Eichenbaum JW, Pevsner PH, Pivawer G, Kleinman GM, Chiriboga L, Stern A, et al. A murine photochemical stroke model with histologic correlates of apoptotic and nonapoptotic mechanisms. J Pharmacol Toxicol Methods. 2002. 47:67–71.


15. Isenmann S, Stoll G, Schroeter M, Krajewski S, Reed JC, Bähr M. Differential regulation of Bax, Bcl-2, and Bcl-X proteins in focal cortical ischemia in the rat. Brain Pathol. 1998. 8:49–62.


16. Nishioka T, Nakase H, Nakamura M, Konishi N, Sakaki T. Sequential and spatial profiles of apoptosis in ischemic penumbra after two-vein occlusion in rats. J Neurosurg. 2006. 104:938–944.


17. Lee JK, Kwak HJ, Piao MS, Jang JW, Kim SH, Kim HS. Quercetin reduces the elevated matrix metalloproteinases-9 level and improves functional outcome after cerebral focal ischemia in rats. Acta Neurochir (Wien). 2011. 153:1321–1329.


18. Broughton BR, Reutens DC, Sobey CG. Apoptotic mechanisms after cerebral ischemia. Stroke. 2009. 40:e331–e339.


19. Pevsner PH, Eichenbaum JW, Miller DC, Pivawer G, Eichenbaum KD, Stern A, et al. A photothrombotic model of small early ischemic infarcts in the rat brain with histologic and MRI correlation. J Pharmacol Toxicol Methods. 2001. 45:227–233.


20. Xing B, Chen H, Zhang M, Zhao D, Jiang R, Liu X, et al. Ischemic postconditioning inhibits apoptosis after focal cerebral ischemia/reperfusion injury in the rat. Stroke. 2008. 39:2362–2369.


21. Okuno S, Saito A, Hayashi T, Chan PH. The c-Jun N-terminal protein kinase signaling pathway mediates Bax activation and subsequent neuronal apoptosis through interaction with Bim after transient focal cerebral ischemia. J Neurosci. 2004. 24:7879–7887.


22. Wu C, Fujihara H, Yao J, Qi S, Li H, Shimoji K, et al. Different expression patterns of Bcl-2, Bcl-xl, and Bax proteins after sublethal forebrain ischemia in C57Black/Crj6 mouse striatum. Stroke. 2003. 34:1803–1808.


23. Panayiotidis MI, Bortner CD, Cidlowski JA. On the mechanism of ionic regulation of apoptosis: would the Na+/K+-ATPase please stand up? Acta Physiol (Oxf). 2006. 187:205–215.


24. Chaitanya GV, Babu PP. Activation of calpain, cathepsin-b and caspase-3 during transient focal cerebral ischemia in rat model. Neurochem Res. 2008. 33:2178–2186.


25. Le DA, Wu Y, Huang Z, Matsushita K, Plesnila N, Augustinack JC, et al. Caspase activation and neuroprotection in caspase-3-deficient mice after in vivo cerebral ischemia and in vitro oxygen glucose deprivation. Proc Natl Acad Sci U S A. 2002. 99:15188–15193.


26. Noshita N, Lewén A, Sugawara T, Chan PH. Akt phosphorylation and neuronal survival after traumatic brain injury in mice. Neurobiol Dis. 2002. 9:294–304.


27. Dietrich WD, Busto R, Watson BD, Scheinberg P, Ginsberg MD. Photochemically induced cerebral infarction. II. Edema and blood-brain barrier disruption. Acta Neuropathol. 1987. 72:326–334.
28. Lee MC, Jin CY, Kim HS, Kim JH, Kim MK, Kim HI, et al. Stem cell dynamics in an experimental model of stroke. Chonnam Med J. 2011. 47:90–98.


29. Conway EM, Zwerts F, Van Eygen V, DeVriese A, Nagai N, Luo W, et al. urvivin-dependent angiogenesis in ischemic brain: molecular mechanisms of hypoxia-induced up-regulation. Am J Pathol. 2003. 163:935–946.