Abstract
Transportation between the cytoplasm and the nucleoplasm is critical for many physiological and pathophysiological processes including gene expression, signal transduction, and oncogenesis. So, the molecular mechanism for the transportation needs to be studied not only to understand cell physiological processes but also to develop new diagnostic and therapeutic targets. Recent progress in the research of the nuclear transportation (import and export) via nuclear pore complex and four important factors affecting nuclear transport (nucleoporins, Ran, karyopherins, and nuclear localization signals/nuclear export signals) will be discussed. Moreover, the clinical significance of nuclear transport and its application will be reviewed. This review will provide some critical insight for the molecular design of therapeutics which need to be targeted inside the nucleus.
The nuclear envelope is a physical barrier which regulates the traffic between nucleoplasm and cytoplasm. It is a phospholipid bilayer membrane which consists of two layers; inner and outer membrane [12]. Inner and outer membranes are separated by the perinuclear space. The cytoplasm is connected to the nucleoplasm via nuclear pores. Although small size of molecules (less than 30 kDa) freely move through the nuclear pore, bigger molecules need the help of special carrier proteins. In the nuclear pore, the nuclear pore complex (NPC) limits the transportation of macromolecules including protein or RNAs.
Recently, new roles of the nuclear pore in gene expression, chromatin organization and DNA repair have been reported in addition to its roles as a physical barrier. Moreover, its abnormal changes like mutation, copy number alteration and mislocalization of molecules, have been associated with various pathologic conditions such as cancers, genetic disorders, and neurodegeneration [23456]. So, investigating its mechanism and disease-associated changes should be helpful for developing novel therapeutic strategies. These abnormal changes could be potential drug targets. Moreover, the necessity to deliver therapeutic DNA or proteins into the nucleus has arisen to treat diseases such as cancer and genetic diseases. Recent progresses in the research of the molecular mechanism for the nuclear transport via NPC, factors affecting the nuclear transport and the application for therapeutics will be summarized in this review.
Transportation of macromolecules including protein or RNAs between nucleoplasm and cytoplasm occurs through NPC in the nuclear envelope. NPC is highly selective and bidirectional transporter for various cargo molecules. There are four important factors for the nuclear transport: (1) nucleoporins (NUPs) that are constituent proteins of NPCs, (2) RanGTPase that allows for active transport and directionality, (3) karyopherins (importin/exportin/transportin) that can recognize cargo molecules, (4) nuclear localization signals (NLSs) or nuclear export signals (NESs) in cargo molecules. NLSs or NESs are recognized by karyopherins. After briefly reviewing the overall process of nuclear transport cycle, four factors will be discussed.
First step of the nuclear transport cycle is the formation of importin-cargo complex. Importins can bind cargo molecules after recognition of their NLSs. Depending on types of NLSs, different importins are involved. For example, classical NLSs can be recognized by importin-α in the cytoplasm where RanGTP is very rare. Then, the N-terminus (importin-β binding domain) of importin-α binds to importin-β (Fig. 1A) [27]. However, importin-β can directly recognize proline-tyrosine (PY) NLS without importin-α, and this pathway is fast and efficient (Fig. 1B) [789101112].
After the formation of importin(s)-cargo complex, importin-β is specifically recruited to NPC in the nuclear pore and then the complex can pass through the nuclear pore. In the nucleoplasm, binding of RanGTP to importin-cargo complex facilitates dissociation of cargo, and then RanGTP-bound importin is exported to the cytoplasm. Recycling of importin-α is mediated by a nuclear export receptor, cellular apoptosis susceptibility protein (CAS), but CAS is not necessary to export of importin-β (Fig. 1).
Cargos containing NES are recognized by exportins. The NES-bound exportin forms a complex with RanGTP. RanGTP is 100-fold more abundant in the nucleoplasm than in the cytoplasm. Binding of RanGTP to exportins induces the high affinity of exportin for its cargo molecule [213]. The exportin-cargo-RanGTP complex specifically binds the docking site of NPCs and then passes through nuclear pore. RanGAP in the cytoplasm hydrolyzes RanGTP in the complex, which leads to the dissociation of the complex (Fig. 1) [21314]. RanGDP is reimported into the nucleoplasm with help of nuclear transport factor 2 [1315].
NPC is a complex basket-like structure with huge molecular mass of 120 MDa in humans. It made up of NUPs with 100–150 nm in diameter and 50–70 nm in thickness according to the species [161718]. Each NPC has a central hole (~30 nm in diameter and ~50 nm in long) that connects between the nucleoplasm and the cytoplasm [17]. Main structures of NPC include the inner pore ring, the nuclear and cytoplasmic rings, the nuclear basket, and the cytoplasmic basket. NPC is composed of multiple copies of 30–50 different NUPs and 500–1,000 NUPs are integrated into the NPC structure. They are functionally conserved throughout eukaryotic cells [2171920].
NUPs serve as an architectural scaffold and a permeability regulator. Some NUPs have intrinsically disordered domains rich in repeating amino acid sequences such as FXFG (Phe-X-Phe-Gly), Phe-Gly (FG), or GLFG (Gly-Leu-Phe-Gly), which act as docking sites for karyopherin-βs (importin or exportin) [161819202122232425262728293031]. These repeating sequences line the central hole and regulate the passage of cargo molecules. NUP62 complex which consists of NUP62, NUP58, and NUP54 has been shown to include FG-repeats [32].
RanGTP gives the nuclear transport directionality. The association of RanGTP with exportins moves the complex out of the nucleoplasm. However, its association with importins leads to the dissociation with cargo molecules. RanGTP is about 100-fold more abundant in the nucleoplasm than in the cytoplasm, which is possible due to the fact that Ran's guanine-exchange factor RCC1 is located in the nucleoplasm but Ran GTPase activating protein, Ran GAP in the cytoplasm [213].
More than 20 karyoperins in human have been reported. Among them, 11 karyopherins (importin-β, importin-β2, importin-4, importin-5, importin-7, importin-8, importin-9, importin-11, transportin-SR, importin-13, exportin-4) are involved in import or bidirectional transport of cargo molecules. Eight exportins (exportin-1–exportin-7, exportin-t) are involved in the export of cargo molecules. Each karyopherin has its own specific cargo molecules. For example, CAS (exportin-2) contributes to the export of importin-α and exportin-t to the export of t-RNA [14].
Importin-β have high molecular flexibility because it can have structural changes using their tandem Huntingtin, elongation factor 3 (EF3), protein phosphatase 2A (PP2A), and the yeast kinase TOR1 (HEAT) repeats which can be thought as a helical spring [17333435]. A single HEAT motif consists of a pair of α-helices and both helices are amphiphilic. Because of an unusual hydrophobic core that supports intramolecular helix-helix interactions, the molecular structure of importin-β is highly flexible [153637]. CAS has similar structure to importin-β and it is based on 19 HEAT repeats [1537].
Importin-α has been shown to contain a tandem series of Armadillo (ARM) repeats that are composed of an array of Trp, Asn, and acidic residues on the inner surface [2383940]. Although ARM motif has three helical structures instead of two in HEAT motif, the superhelical 3D structure of ARM repeats is similar with that of HEAT repeats.
Karyopherins can also export nucleotide motif including tRNA, miRNA, rRNA, viral RNA, and uridine-rich small nuclear RNAs with or without ribonucleoprotein as an adaptor [71441424344]. However, structure of mRNAs is highly diverse unlike other RNAs, so that they can be transported by processing and assembly into mRNs [14].
The best well characterized NLS is classical NLSs for the nuclear protein import. Classical NLSs contain monopartite or bipartite signals that can be recognized by importin-α. A first characterized classical monopartite NLS is simian virus 40 (SV40) large T antigen (PKKKRKV) (Fig. 2). Bipartite NLSs have two clusters of basic amino acids sequence. The prototypical bipartite NLS is nucleoplasmin found in Xenopus laevis (KRPAATKKAGQAKKKK) (Fig. 2) [431344546474849]. The classical NLS is thought as the typical NLS because it was the first NLS to be defined.
In contrast to classical NLSs, PY-NLS has diverse sequence and large structure, nonetheless, importin-βs can recognize them by multiple attractions of weak affinities between NLS and importin [72125]. PY-NLS sequences are composed of a loose N-terminal hydrophobic motifs and a C-terminal RX2-5PY motif [735]. hnRNP A1, Hrp1 are the representative PY-NLSs (Fig. 2) [3550].
Karyopherin121 (Kap121) is one of the most essential for nuclear transport in Saccharomyces cerevisiae and it can mediate transportation for diverse cargos [5152]. Recent studies demonstrated that the small lysine-rich NLSs (consensus sequences: K-V/I-X-K-X1-2-K/H/R) interact with Kap121 [5152].
Transportin3 (Trn3) binds the cargo containing RS (Arg-Ser) repeats domain especially phosphorylated RS repeats. In proteomic analysis, about 32% of Trn3 cargos have RS repeats [5153]. Other cargos of Trn3 contain RE (Arg-Glu) or RD (Arg-Asp) motifs, which may imitate phosphoRS motifs [51]. According to identification of new cargos for importins, additional consensus sequences for new classification will be required.
There are many other NLSs recognized by importin-α. Representative sequences from Borna Disease Virus p10 protein and S. cerevisiae phospholipid scramblase 1 contain hydrophobic residues (Fig. 2) [1754]. Importin-β also binds various other NLSs included in CREB, ribosomal proteins, the human immunodeficiency virus Rev and Tat, the human T-cell leukemia virus type 1 protein Rex, PTHrP, cyclin B1, Smad3, SREBP-2, and TRF (Fig. 2) [3789101112133536375055565758].
The consensus sequence for NESs is Φ1-X(2-3)-Φ2-X(2-3)-Φ3-X-Φ4 motif (Φ: represents hydrophobic residues L, I, F, M, or V and X: any amino acid). Different exportins have their specific cargo molecules. For example, CAS (exportin-2) transports importin-α. CRM1 (exportin-1) is a ubiquitous nuclear export receptor containing hydrophobic residues. Binding site of CRM1 consists of five pockets [113161823242730]. CRM1 can recognize relatively diverse molecules. Overexpression of CRM1 was noted in many types of cancer [21920].
Several factors such as NLSs, NESs, and 3D structures need to be considered to improve the efficiency of nuclear transport. NLSs are well-studied part in the nuclear transport, and commercial NLS peptides including HIV-Tat, penetratin, and (Arg)9, are available for the gene delivery. However, as the importance of conformation is being emphasized, many researches have focused on the 3D structures.
NLSs can be recognized as linear and/or conformational signals by importins. The 3D structure of NLSs would be changed in NLSs-bound complex, which causes changes of binding affinity with importin. Many groups has made many efforts to bridge the gap between 3D structure and binding capacity of complex (Fig. 3) [41424344]. Karyopherins have different binding sites according to their subtypes and binding residues of certain karyopherin can be different according to different kinds of cargo. One study demonstrated that the C-terminal and N-terminal structures of NLS significantly affect the efficiency of the nuclear transport as well as their binding affinity to importin [5960]. Kim et al. [61], compared with structurally modified SV40 NLS peptides. They made modified peptides from SV40 NLS by addition of cysteine, deletion of cysteine, homodimerization, or circularization. These modifications showed different transfection efficiency according to structures even though the NLS has same sequences.
Furthermore, although many researchers have tried different amount of NLS peptides which were fused with cargos and different locations for the nuclear delivery, the effects of them still require further study [596061]. Overall, alteration of 3D structure can change the binding affinity to importin and the conformation is a more important factor to be considered when we use NLS peptides for the nuclear delivery.
In multiple cancers, changes in the expression of karyopherins including importin-α2, CAS, importin-β1, exportin-1, have been reported [24662]. Overexpression of exportin-1 has been associated with various cancers including acute and chronic leukemia, multiple myeloma, ovarian cancer, pancreatic cancer, gastric cancer, sarcoma, melanoma, glioma, and cervical cancer [62]. A small molecule inhibitor for exportin-1 showed some beneficial effects in the clinical trial [62]. In cancer cells, exportin-1 played critical roles in exporting various tumor suppressor proteins including retinoblastoma protein, adenomatous polyposis coli, p53, p21, p27, FOXO, IκB, topoisomerase II, and PAR-4 [26].
Furthermore, abnormal localization of proteins due to abnormalities in the nuclear transport, can cause various disorders that involve protein aggregation, biosynthesis, or cell metabolism [4663646566676869]. For example, sex-determining region Y (SRY) is transported by a specific importin to the nucleus to activate testis-related genes. There are two NLS motifs in the DNA-binding domain of SRY that are called high-mobility group box. Mutations of NLS of SRY have been observed to be associated with Swyer syndrome. Swyer syndrome is a sex reversal disease of male caused by XY gonadal dysgenesis [464548]. A similar alteration was reported in NLS of trichorhinophalangeal syndrome type 1 gene in patients with trichorhinophalangeal syndrome type I [669]. Additionally, alterations in several NLSs of disease-related proteins have been identified; short stature homeobox in patients with Léri-Weill dyschondrosteosis, aristaless related homeobox in patients with X-linked lissencephaly, forkhead box P2 in patients with speech-language disorder [6656667].
The necessity to deliver therapeutic DNAs or proteins into the nucleus has been increased to correct genetic or nuclear abnormalities. Although its efficiency is high, viral transduction showed several critical problems such as immunogenic response and safety risk. Non-viral methods are safe but their delivery efficiency are low [70].
To increase the efficiency of the nuclear delivery, NLSs have been used. Instead of long sequences of NLSs, researchers inserted short NLSs like SV40, (Arg)x or HIV-tat. Some studies developed a plasmid DNA containing the NLS of SV40, and thereby the transfection efficiency of the target DNA was increased [6870]. The insertion of NLS showed great results for the delivery of CRISPR/CAS system. For example, Ramakrishna et al. [71], developed (Arg)9-mediated RNA-guided endonucleases (RGEN) delivery process [71727374]. Furthermore, TAT-based gene delivery systems was also developed to enhance the efficiency of nuclear import [75]. Simple insertion of NLSs in the target molecules cannot be the solution for delivery to the nucleus, because NLSs can be hindered in inserted status. So, 3D structure of drugs should be considered for the nuclear targeting.
Many researchers have tried to develop ideal drug delivery systems by using modification of NLSs [72737677]. Based on previous results, several bioinformatic tools, such as NucPred, NLS Mapper, NESbase and NLSdb, have been developed about subcellular localization of proteins [787980818283]. Using these tools, we can search the subcellular locations of targeting molecules. More importantly, users can predict subcellular location of their own drugs containing NLSs by using NucPred website (http://www.sbc.su.se/~maccallr/nucpred/) [78]. Fig. 2 shows the predictive subcellular location of popular NLS sequences based on NucPred.
Based on its clinical significance, further studies about roles and molecular mechanisms about the nuclear transport need to be done in the future study. How to improve the efficiency of NLSs for the nuclear delivery has been discussed. To enhance the delivery efficiency by using NLSs, several factors such as its types and 3D structures should be considered. If we can predict nuclear transport efficiency based on 3D structures of cargos-karyopherin complex, it might be very helpful to develop treatment methods.
Figures and Tables
Fig. 1
The nuclear transport cycle of proteins. (A) Import cycle for importin-αβ. (B) Import cycle for importin-β. (C) Export cycle. See main text for details. NLS, nuclear localization signal; NPC, nuclear pore complex; NES, nuclear export signal.
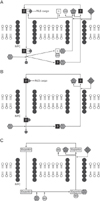
Fig. 2
Examples of NLSs and predictive location of NLSs. PY, proline-tyrosine; NLS, nuclear localization signal.
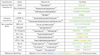
Fig. 3
3D structures of representative karyopherin-cargo complex. (A) Structure of importin-α with HIV-1 Tat NLS. (B) Structure of importin-β with RpL4 PY-NLS. (C) Structure of CRM1-Ran-RanBP1 with CPEB4 NES. Indicated number of amino acid residues are the binding sites of each karyopherins. These figures downloaded from RCSB PDB (http://www.rcsb.org/pdb/home/home.do). NLS, nuclear localization signal; PY, proline-tyrosine; NES, nuclear export signal.
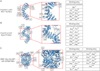
References
1. Cautain B, Hill R, de Pedro N, Link W. Components and regulation of nuclear transport processes. FEBS J. 2015; 282:445–462.
2. Kau TR, Way JC, Silver PA. Nuclear transport and cancer: from mechanism to intervention. Nat Rev Cancer. 2004; 4:106–117.
3. Bakhoum SF, Compton DA. Chromosomal instability and cancer: a complex relationship with therapeutic potential. J Clin Invest. 2012; 122:1138–1143.
4. McLane LM, Corbett AH. Nuclear localization signals and human disease. IUBMB Life. 2009; 61:697–706.
5. Patel VP, Chu CT. Nuclear transport, oxidative stress, and neurodegeneration. Int J Clin Exp Pathol. 2011; 4:215–229.
6. Hung MC, Link W. Protein localization in disease and therapy. J Cell Sci. 2011; 124(Pt 20):3381–3392.
7. Chook YM, Süel KE. Nuclear import by karyopherin-betas: recognition and inhibition. Biochim Biophys Acta. 2011; 1813:1593–1606.
8. Chook YM, Blobel G. Karyopherins and nuclear import. Curr Opin Struct Biol. 2001; 11:703–715.
9. Conti E, Izaurralde E. Nucleocytoplasmic transport enters the atomic age. Curr Opin Cell Biol. 2001; 13:310–319.
10. Görlich D, Kutay U. Transport between the cell nucleus and the cytoplasm. Annu Rev Cell Dev Biol. 1999; 15:607–660.
11. Tran EJ, Bolger TA, Wente SR. SnapShot: nuclear transport. Cell. 2007; 131:420.
12. Weis K. Regulating access to the genome: nucleocytoplasmic transport throughout the cell cycle. Cell. 2003; 112:441–451.
13. Wente SR, Rout MP. The nuclear pore complex and nuclear transport. Cold Spring Harb Perspect Biol. 2010; 2:a000562.
14. Köhler A, Hurt E. Exporting RNA from the nucleus to the cytoplasm. Nat Rev Mol Cell Biol. 2007; 8:761–773.
15. Stewart M. Molecular mechanism of the nuclear protein import cycle. Nat Rev Mol Cell Biol. 2007; 8:195–208.
16. Turner JG, Dawson J, Cubitt CL, Baz R, Sullivan DM. Inhibition of CRM1-dependent nuclear export sensitizes malignant cells to cytotoxic and targeted agents. Semin Cancer Biol. 2014; 27:62–73.
17. Wolff T, Unterstab G, Heins G, Richt JA, Kann M. Characterization of an unusual importin alpha binding motif in the borna disease virus p10 protein that directs nuclear import. J Biol Chem. 2002; 277:12151–12157.
18. Zolotukhin AS, Felber BK. Nucleoporins nup98 and nup214 participate in nuclear export of human immunodeficiency virus type 1 Rev. J Virol. 1999; 73:120–127.
19. Kudo N, Matsumori N, Taoka H, Fujiwara D, Schreiner EP, Wolff B, Yoshida M, Horinouchi S. Leptomycin B inactivates CRM1/exportin 1 by covalent modification at a cysteine residue in the central conserved region. Proc Natl Acad Sci U S A. 1999; 96:9112–9117.
20. Kudo N, Wolff B, Sekimoto T, Schreiner EP, Yoneda Y, Yanagida M, Horinouchi S, Yoshida M. Leptomycin B inhibition of signal-mediated nuclear export by direct binding to CRM1. Exp Cell Res. 1998; 242:540–547.
21. Cansizoglu AE, Lee BJ, Zhang ZC, Fontoura BM, Chook YM. Structure-based design of a pathway-specific nuclear import inhibitor. Nat Struct Mol Biol. 2007; 14:452–454.
22. Carmody SR, Wente SR. mRNA nuclear export at a glance. J Cell Sci. 2009; 122(Pt 12):1933–1937.
23. Fornerod M, Ohno M, Yoshida M, Mattaj IW. CRM1 is an export receptor for leucine-rich nuclear export signals. Cell. 1997; 90:1051–1060.
24. Fornerod M, van Deursen J, van Baal S, Reynolds A, Davis D, Murti KG, Fransen J, Grosveld G. The human homologue of yeast CRM1 is in a dynamic subcomplex with CAN/Nup214 and a novel nuclear pore component Nup88. EMBO J. 1997; 16:807–816.
25. Imasaki T, Shimizu T, Hashimoto H, Hidaka Y, Kose S, Imamoto N, Yamada M, Sato M. Structural basis for substrate recognition and dissociation by human transportin 1. Mol Cell. 2007; 28:57–67.
26. Ohno M, Segref A, Bachi A, Wilm M, Mattaj IW. PHAX, a mediator of U snRNA nuclear export whose activity is regulated by phosphorylation. Cell. 2000; 101:187–198.
27. Ossareh-Nazari B, Bachelerie F, Dargemont C. Evidence for a role of CRM1 in signal-mediated nuclear protein export. Science. 1997; 278:141–144.
28. Rodriguez MS, Dargemont C, Stutz F. Nuclear export of RNA. Biol Cell. 2004; 96:639–655.
29. Simos G, Grosshans H, Hurt E. Nuclear export of tRNA. Results Probl Cell Differ. 2002; 35:115–131.
30. Stade K, Ford CS, Guthrie C, Weis K. Exportin 1 (Crm1p) is an essential nuclear export factor. Cell. 1997; 90:1041–1050.
31. Südbeck P, Scherer G. Two independent nuclear localization signals are present in the DNA-binding high-mobility group domains of SRY and SOX9. J Biol Chem. 1997; 272:27848–27852.
32. Tran EJ, King MC, Corbett AH. Macromolecular transport between the nucleus and the cytoplasm: advances in mechanism and emerging links to disease. Biochim Biophys Acta. 2014; 1843:2784–2795.
33. Conti E, Uy M, Leighton L, Blobel G, Kuriyan J. Crystallographic analysis of the recognition of a nuclear localization signal by the nuclear import factor karyopherin alpha. Cell. 1998; 94:193–204.
34. Dingwall C, Laskey RA. Nuclear targeting sequences: a consensus? Trends Biochem Sci. 1991; 16:478–481.
35. Lee BJ, Cansizoglu AE, Süel KE, Louis TH, Zhang Z, Chook YM. Rules for nuclear localization sequence recognition by karyopherin beta 2. Cell. 2006; 126:543–558.
36. Conti E, Müller CW, Stewart M. Karyopherin flexibility in nucleocytoplasmic transport. Curr Opin Struct Biol. 2006; 16:237–244.
37. Cook A, Fernandez E, Lindner D, Ebert J, Schlenstedt G, Conti E. The structure of the nuclear export receptor Cse1 in its cytosolic state reveals a closed conformation incompatible with cargo binding. Mol Cell. 2005; 18:355–367.
38. Conti E, Kuriyan J. Crystallographic analysis of the specific yet versatile recognition of distinct nuclear localization signals by karyopherin alpha. Structure. 2000; 8:329–338.
39. Isoyama T, Murayama A, Nomoto A, Kuge S. Nuclear import of the yeast AP-1-like transcription factor Yap1p is mediated by transport receptor Pse1p, and this import step is not affected by oxidative stress. J Biol Chem. 2001; 276:21863–21869.
40. Kaffman A, Rank NM, O'Shea EK. Phosphorylation regulates association of the transcription factor Pho4 with its import receptor Pse1/Kap121. Genes Dev. 1998; 12:2673–2683.
41. Hing ZA, Fung HY, Ranganathan P, Mitchell S, El-Gamal D, Woyach JA, Williams K, Goettl VM, Smith J, Yu X, Meng X, Sun Q, Cagatay T, Lehman AM, Lucas DM, Baloglu E, Shacham S, Kauffman MG, Byrd JC, Chook YM, Garzon R, Lapalombella R. Next-generation XPO1 inhibitor shows improved efficacy and in vivo tolerability in hematological malignancies. Leukemia. 2016; 30:2364–2372.
42. Huber FM, Hoelz A. Molecular basis for protection of ribosomal protein L4 from cellular degradation. Nat Commun. 2017; 8:14354.
43. Rose AS, Bradley AR, Valasatava Y, Duarte JM, Prlić A, Rose PW. Web-based molecular graphics for large complexes. In : Proceedings of the 21st International Conference on Web3D Technolog; 2016 Jul 22-24; Anaheim, California. New York: ACM;2016. p. 185–186.
44. Rose AS, Hildebrand PW. NGL Viewer: a web application for molecular visualization. Nucleic Acids Res. 2015; 43:W576–W579.
45. Forwood JK, Harley V, Jans DA. The C-terminal nuclear localization signal of the sex-determining region Y (SRY) high mobility group domain mediates nuclear import through importin beta 1. J Biol Chem. 2001; 276:46575–46582.
46. Kalderon D, Richardson WD, Markham AF, Smith AE. Sequence requirements for nuclear location of simian virus 40 large-T antigen. Nature. 1984; 311:33–38.
47. Kalderon D, Roberts BL, Richardson WD, Smith AE. A short amino acid sequence able to specify nuclear location. Cell. 1984; 39(3 Pt 2):499–509.
48. Poulat F, Girard F, Chevron MP, Gozé C, Rebillard X, Calas B, Lamb N, Berta P. Nuclear localization of the testis determining gene product SRY. J Cell Biol. 1995; 128:737–748.
49. Robbins J, Dilworth SM, Laskey RA, Dingwall C. Two interdependent basic domains in nucleoplasmin nuclear targeting sequence: identification of a class of bipartite nuclear targeting sequence. Cell. 1991; 64:615–623.
50. Lange A, Mills RE, Devine SE, Corbett AH. A PY-NLS nuclear targeting signal is required for nuclear localization and function of the Saccharomyces cerevisiae mRNA-binding protein Hrp1. J Biol Chem. 2008; 283:12926–12934.
51. Soniat M, Chook YM. Nuclear localization signals for four distinct karyopherin-beta nuclear import systems. Biochem J. 2015; 468:353–362.
52. Kobayashi J, Matsuura Y. Structural basis for cell-cycle-dependent nuclear import mediated by the karyopherin Kap121p. J Mol Biol. 2013; 425:1852–1868.
53. Maertens GN, Cook NJ, Wang W, Hare S, Gupta SS, Öztop I, Lee K, Pye VE, Cosnefroy O, Snijders AP, KewalRamani VN, Fassati A, Engelman A, Cherepanov P. Structural basis for nuclear import of splicing factors by human Transportin 3. Proc Natl Acad Sci U S A. 2014; 111:2728–2733.
54. Chen MH, Ben-Efraim I, Mitrousis G, Walker-Kopp N, Sims PJ, Cingolani G. Phospholipid scramblase 1 contains a nonclassical nuclear localization signal with unique binding site in importin alpha. J Biol Chem. 2005; 280:10599–10606.
55. Leslie DM, Grill B, Rout MP, Wozniak RW, Aitchison JD. Kap121p-mediated nuclear import is required for mating and cellular differentiation in yeast. Mol Cell Biol. 2002; 22:2544–2555.
56. Moye-Rowley WS, Harshman KD, Parker CS. Yeast YAP1 encodes a novel form of the jun family of transcriptional activator proteins. Genes Dev. 1989; 3:283–292.
57. Roberts RL, Fink GR. Elements of a single MAP kinase cascade in Saccharomyces cerevisiae mediate two developmental programs in the same cell type: mating and invasive growth. Genes Dev. 1994; 8:2974–2985.
58. Truant R, Cullen BR. The arginine-rich domains present in human immunodeficiency virus type 1 Tat and Rev function as direct importin beta-dependent nuclear localization signals. Mol Cell Biol. 1999; 19:1210–1217.
59. Eguchi A, Furusawa H, Yamamoto A, Akuta T, Hasegawa M, Okahata Y, Nakanishi M. Optimization of nuclear localization signal for nuclear transport of DNA-encapsulating particles. J Control Release. 2005; 104:507–519.
60. Sun Y, Xian L, Xing H, Yu J, Yang Z, Yang T, Yang L, Ding P. Factors influencing the nuclear targeting ability of nuclear localization signals. J Drug Target. 2016; 24:927–933.
61. Kim BK, Kang H, Doh KO, Lee SH, Park JW, Lee SJ, Lee TJ. Homodimeric SV40 NLS peptide formed by disulfide bond as enhancer for gene delivery. Bioorg Med Chem Lett. 2012; 22:5415–5418.
62. Mahipal A, Malafa M. Importins and exportins as therapeutic targets in cancer. Pharmacol Ther. 2016; 164:135–143.
63. Hoover BR, Reed MN, Su J, Penrod RD, Kotilinek LA, Grant MK, Pitstick R, Carlson GA, Lanier LM, Yuan LL, Ashe KH, Liao D. Tau mislocalization to dendritic spines mediates synaptic dysfunction independently of neurodegeneration. Neuron. 2010; 68:1067–1081.
64. Mendes HF, van der Spuy J, Chapple JP, Cheetham ME. Mechanisms of cell death in rhodopsin retinitis pigmentosa: implications for therapy. Trends Mol Med. 2005; 11:177–185.
65. Mizutani A, Matsuzaki A, Momoi MY, Fujita E, Tanabe Y, Momoi T. Intracellular distribution of a speech/language disorder associated FOXP2 mutant. Biochem Biophys Res Commun. 2007; 353:869–874.
66. Sabherwal N, Schneider KU, Blaschke RJ, Marchini A, Rappold G. Impairment of SHOX nuclear localization as a cause for Leri-Weill syndrome. J Cell Sci. 2004; 117(Pt 14):3041–3048.
67. Shoubridge C, Tan MH, Fullston T, Cloosterman D, Coman D, McGillivray G, Mancini GM, Kleefstra T, Gécz J. Mutations in the nuclear localization sequence of the Aristaless related homeobox: sequestration of mutant ARX with IPO13 disrupts normal subcellular distribution of the transcription factor and retards cell division. Pathogenetics. 2010; 3:1.
68. Wilson GL, Dean BS, Wang G, Dean DA. Nuclear import of plasmid DNA in digitonin-permeabilized cells requires both cytoplasmic factors and specific DNA sequences. J Biol Chem. 1999; 274:22025–22032.
69. Kaiser FJ, Brega P, Raff ML, Byers PH, Gallati S, Kay TT, de Almeida S, Horsthemke B, Lüdecke HJ. Novel missense mutations in the TRPS1 transcription factor define the nuclear localization signal. Eur J Hum Genet. 2004; 12:121–126.
70. Escriou V, Carrière M, Scherman D, Wils P. NLS bioconjugates for targeting therapeutic genes to the nucleus. Adv Drug Deliv Rev. 2003; 55:295–306.
71. Ramakrishna S, Kwaku Dad AB, Beloor J, Gopalappa R, Lee SK, Kim H. Gene disruption by cell-penetrating peptide-mediated delivery of Cas9 protein and guide RNA. Genome Res. 2014; 24:1020–1027.
72. Cong L, Ran FA, Cox D, Lin S, Barretto R, Habib N, Hsu PD, Wu X, Jiang W, Marraffini LA, Zhang F. Multiplex genome engineering using CRISPR/Cas systems. Science. 2013; 339:819–823.
73. Ma S, Chang J, Wang X, Liu Y, Zhang J, Lu W, Gao J, Shi R, Zhao P, Xia Q. CRISPR/Cas9 mediated multiplex genome editing and heritable mutagenesis of BmKu70 in Bombyx mori. Sci Rep. 2014; 4:4489.
74. Wang HY, Chen JX, Sun YX, Deng JZ, Li C, Zhang XZ, Zhuo RX. Construction of cell penetrating peptide vectors with N-terminal stearylated nuclear localization signal for targeted delivery of DNA into the cell nuclei. J Control Release. 2011; 155:26–33.
75. Yi WJ, Yang J, Li C, Wang HY, Liu CW, Tao L, Cheng SX, Zhuo RX, Zhang XZ. Enhanced nuclear import and transfection efficiency of TAT peptide-based gene delivery systems modified by additional nuclear localization signals. Bioconjug Chem. 2012; 23:125–134.
76. Weisbart RH, Chan G, Jordaan G, Noble PW, Liu Y, Glazer PM, Nishimura RN, Hansen JE. DNA-dependent targeting of cell nuclei by a lupus autoantibody. Sci Rep. 2015; 5:12022.
77. Weisbart RH, Gera JF, Chan G, Hansen JE, Li E, Cloninger C, Levine AJ, Nishimura RN. A cell-penetrating bispecific antibody for therapeutic regulation of intracellular targets. Mol Cancer Ther. 2012; 11:2169–2173.
78. Brameier M, Krings A, MacCallum RM. NucPred: predicting nuclear localization of proteins. Bioinformatics. 2007; 23:1159–1160.
79. Emanuelsson O, Nielsen H, Brunak S, von Heijne G. Predicting subcellular localization of proteins based on their N-terminal amino acid sequence. J Mol Biol. 2000; 300:1005–1016.
80. Kosugi S, Hasebe M, Tomita M, Yanagawa H. Systematic identification of cell cycle-dependent yeast nucleocytoplasmic shuttling proteins by prediction of composite motifs. Proc Natl Acad Sci U S A. 2009; 106:10171–10176.
81. la Cour T, Gupta R, Rapacki K, Skriver K, Poulsen FM, Brunak S. NESbase version 1.0: a database of nuclear export signals. Nucleic Acids Res. 2003; 31:393–396.
82. Nair R, Carter P, Rost B. NLSdb: database of nuclear localization signals. Nucleic Acids Res. 2003; 31:397–399.
83. Nair R, Rost B. Mimicking cellular sorting improves prediction of subcellular localization. J Mol Biol. 2005; 348:85–100.