Abstract
The genus Populus (poplar) belonging to the Salicaceae family has been used in traditional medicine, and its several species show various pharmacological properties including antioxidant and anti-inflammatory effects. No study regarding protective effects of Populus species against cerebral ischemia has been reported. Therefore, in the present study, we examined neuroprotective effects of ethanol extract from Populus tomentiglandulosa (Korea poplar) in the hippocampal cornu ammonis (CA1) area of gerbils subjected to 5 minutes of transient global cerebral ischemia. Pretreatment with 200 mg/kg of P. tomentiglandulosa extract effectively protected CA1 pyramidal neurons from transient global cerebral ischemia. In addition, glial fibrillary acidic protein immunoreactive astrocytes and ionized calcium binding adapter molecule 1 immunoreactive microglia were significantly diminished in the ischemic CA1 area by pretreatment with 200 mg/kg of P. tomentiglandulosa extract. Briefly, our results indicate that pretreatment with P. tomentiglandulosa extract protects neurons from transient cerebral ischemic injury and diminish cerebral ischemia-induced reactive gliosis in ischemic CA1 area. Based on these results, we suggest that P. tomentiglandulosa can be used as a potential candidate for prevention of ischemic injury.
Transient global cerebral ischemia (tGCI), which is caused by lack of blood supply to the brain, results in irreversible neuronal death in venerable regions of the brain, such as the hippocampus and cerebral cortex [12]. The hippocampus is extremely sensitive to cerebral ischemic injury [3]. In particular, pyramidal neurons in the cornu ammonis 1 (CA1) are killed from several days after tGCI [45].
Medicinal plants, which have been used to heal various ailments in humans, have received considerable interest in research fields for treatments of various diseases including neurological disorders, because many medicinal plants have a variety of biological properties [678]. The genus Populus (poplar) belonging to the Salicaceae family includes various species distributes in template zone and subtropical areas, and several species of genus Populus have been used in traditional medicine [910]. For example, Populus nigra and Populus davidiana possess pharmacological actions including antioxidant, anti-inflammatory and hepatoprotective properties [1112]. Furthermore, Populus balsamifera has pharmacological potential for treatment of obesity and diabetes [13]. However, among Populus species, few studies regarding scientific validation of pharmacological actions of P. tomentiglandulosa (Korea poplar), which is a species of the genus Populus, have been reported. Especially, to the best of our knowledge, protective effects of P. tomentiglandulosa against cerebral ischemic insults have never been investigated. Therefore, in the present study, we examined neuroprotective effects of P. tomentiglandulosa extract in the hippocampal CA1 using gerbils, which are a good model of tGCI [1415].
P. tomentiglandulosa was collected in September 2014 by Professor Jong Dai Kim in Kangwon province (South Korea). For the preparation of ethanol P. tomentiglandulosa extract (PTE), P. tomentiglandulosa was washed with distilled water, air-dried at 60℃ and ground into fine powder by a grinder (IKA M20, IKA, Staufen, Germany). The P. tomentiglandulosa powder was refluxed with 10 vol (v/w) of 70% ethanol at 70℃ for 24 hours, and the extraction procedure was repeated three times. The extract was filtered via Whatman No. 1 filter paper (Whatman Ltd., Maidstone, Kent, UK), concentrated with a vacuum evaporator, and it was completely dried with a freeze-drier. The extraction yield was 14.5%.
We used male Mongolian gerbils (6 months of age and 70–80 g of body weight). Experimental protocol was approved (approval No. KW-160802-1) by the Institutional Animal Care and Use Committee at Kangwon National University. Animals were divided into 6 groups (n=7 in each group): (1) vehicle-sham group, which was treated with vehicle (sterile normal saline; 0.9% w/v NaCl) and given sham operation, (2) vehicle-tGCI group, which was treated with vehicle and given tGCI, (3) and (4) PTE-sham group, which was treated with 100 and 200 mg/kg PTE, respectively, and given sham operation, and (5) and (6) PTE-tGCI group, which was treated with 100 and 200 mg/kg PTE, respectively, and given tGCI. PTE was dissolved in saline, and PTE or saline was orally administered once a day for 7 days before ischemic surgery. We adapted the oral administration of PTE for 1 week, because extracts from plants have been taken orally in traditional medicine and no data regarding the absorption and metabolism of PTE haven reported.
In brief, according to our published method [4], the gerbils were anesthetized with a mixture of 2.5% isoflurane in 33% oxygen and 67% nitrous oxide, and both common carotid arteries were occluded for 5 minutes. Restoration of blood flow (reperfusion) was directly observed under an ophthalmoscope. Body (rectal) temperature was maintained under normothermic (37±0.5℃) condition before, during and after tGCI. Sham-operated animals were subjected to the same surgical procedures except that the common carotid arteries were not occluded.
As we described previously [15], briefly, the gerbils were anesthetized with sodium pentobarbital (30 mg/kg, intraperitoneally) and perfused transcardially with 4% paraformaldehyde 5 days after tGCI. Their brains were removed and postfxed in the same fxative for 6 hours, cryoprotected by infiltration with 30% sucrose, and the frozen bran tissues were serially sectioned into 30-µm coronal sections in a cryostat (Leica, Wetzler, Germany).
To examine distribution pattern of cells in the ischemic hippocampus, the hippocampal sections were stained with cresyl violet (CV) according to our protocol [15]. Briefly, cresyl violet acetate (Sigma-Aldrich, St. Louis, MO, USA) was dissolved at 1.0% (w/v) in distilled water, and glacial acetic acid was added to this solution. The sections were stained, subsequently dehydrated by immersing in serial ethanol baths and mounted with Canada balsam (Tokyo, Japan).
In order to examine neuronal damage and change in astrocytes and microglia, immunohistochemistry was carried out according to our published method [16]. Briefly, we used mouse anti-neuronal nuclear antigen (NeuN, 1:1,000, Chemicon, Temecula, CA, USA) for neurons, mouse anti-glial fibrillary acidic protein (GFAP, 1:800, Chemicon) for astrocytes, and rabbit anti-ionized calcium-binding adapter molecule 1 (Iba-1, 1:800, Chemicon) for microglia as primary antibodies. The sections were incubated with each primary antibody, exposed to biotinylated goat anti-mouse or rabbit IgG and streptavidin peroxidase complex (Vector Laboratories, Burlingame, CA, USA), and visualized with 3,3′-diaminobenzidine solution.
Data analysis were done as previously described [16]. In brief, for quantitative analysis of NeuN-immunoreactive (+) neurons, we selected 10 section with 150-µm interval in each animal, and captured digital images of the CA1 with an AxioM1 light microscope (Carl Zeiss, Jena, Germany) equipped with a digital camera (Axiocam, Carl Zeiss) connected to a PC monitor. NeuN+ neurons were counted in a 250×250 µm square applied at the center of the CA1 using an image analyzing system (software: Optimas 6.5, CyberMetrics, Scottsdale, AZ, USA). Cell counts were obtained by averaging total cell numbers.
For quantitative analyses of GFAP+ and Iba-1+ structures, their images were calibrated into an array of 512×512 pixels corresponding to a tissue area of 140×140 µm (40× primary magnification). Each immunoreactivity was measured by 0–255 gray scale system, and a ratio of relative optical density (ROD) for GFAP+ and Iba-1+ structures was calibrated as % using Adobe Photoshop version 8.0 and then analyzed using NIH Image 1.59 software. A ratio of the ROD was calibrated as %, with the vehicle-sham group designated as 100%.
The data shown here represent the means±SEM. Differences of the means among the groups were statistically analyzed by one-way analysis of variance (ANOVA) with a post hoc Bonferroni's multiple comparison test in order to elucidate ischemia-related differences among experimental groups. Statistical significance was considered at P<0.05.
In the vehicle-sham group, cells were well stained with CV in all layers of the hippocampus (Fig. 1A, B). In the 100 mg/kg and 200 mg/kg PTE-sham groups, the distribution pattern of CV+ cells was similar to that in the vehicle-sham group (Fig. 1E, F, I, J).
In the vehicle-tGCI group, pyramidal cells in the stratum pyramidale were not stained with CV only in the CA1 5 days after tGCI (Fig. 1C, D). In the 100 mg/kg PTE-tGCI group, the CA1 pyramidal cells were not stained with CV 5 days after tGCI, which means that CA1 pyramidal cells were not protected from tGCI (Fig. 1G, H). On the other hand, in the 200 mg/kg PTE-tGCI group, the CA1 pyramidal neurons were well stained with CV 5 days after tGCI, which means that CA1 pyramidal cells were well protected from tGCI (Fig. 1K, L).
The CA1 pyramidal cells showed strong NeuN immunoreactivity in all sham operated groups (Fig. 2A–C), and no significant difference in the number of NeuN+ cells between those groups was observed (Fig. 2G).
In the vehicle-tGCI group, a few NeuN+ cells (7±2.3 neurons) were found in the CA1 stratum pyramidale at 5 days post-tGCI (Fig. 2D, G). In the 100 mg/kg PTE-tGCI group, the distribution and number of NeuN+ cells (11±3.5 neurons) were not significantly different from those in the vehicle-tGCI group (Fig. 2E, G). However, in the 200 mg/kg PTE-tGCI group, many NeuN+ cells (70±2.7 neurons) were detected in the CA1 stratum pyramidale, and the mean number of NeuN+ cells was similar to that in the vehicle-sham group (Fig. 2F, G).
In the vehicle-sham group, normal GFAP+ astrocytes were found in the strata oriens and radiatum of the CA1, and they showed small cell bodies and thread-like processes (Fig. 3A). In the 100 and 200 mg/kg PTE-sham groups, the morphology, distribution and ROD of GFAP+ astrocytes were similar to those in the vehicle-sham group (Fig. 3B, C, G).
In the vehicle-tGCI group, the cytoplasm of GFAP+ astrocytes was hypertrophied with thickened processes, and the ROD of GFAP+ structures was significantly increased 5 days after tGCI compared with that in the vehicle-sham group (about 264% of the vehicle-sham group) (Fig. 3D, F). In the 100 mg/kg PTE-tGCI group, the morphology and ROD of GFAP+ astrocytes were not significantly different from those in the vehicle-tGCI group (Fig. 3E, G). Whereas, in the 200 mg/kg PTE-tGCI group, GFAP+ astrocytes were significantly less hypertrophied (Fig. 3F), and their ROD was significantly deceased compared with those in the vehicle-tGCI group (about 122% of the vehicle-sham group) (Fig. 3G).
In the vehicle-sham group, Iba-1+ microglia showed small cytoplasm and long processes, and they were distributed throughout the CA1 (Fig. 4A). In the 100 and 200 mg/kg PTE-sham groups, the morphology and distribution of Iba-1+ microglia and their ROD were not changed compared with those in the vehicle-sham group (Fig. 4B, C, G).
In the vehicle-tGCI group, Iba-1+ microglia showed large cytoplasm and thick processes, and many of them were aggregated in the stratum pyramidale (Fig. 4D). In addition, in this group, the ROD of Iba-1+ microglia was significantly increased compared with that in the vehicle-sham group (about 220% of the vehicle-sham group) (Fig. 4G). In the 100 mg/kg PTE-tGCI group, the morphology, distribution and ROD of Iba-1+ microglia were not significantly different from the vehicle-tGCI group (Fig. 4E, G). However, in the 200 mg/kg PTE-tGCI group, their morphology and distribution were not significantly changed from those in the vehicle-sham group (Fig. 4F), and their ROD was significantly low compared with that in the vehicle-tGCI group (about 122% of the vehicle-sham group) (Fig. 4G).
Until now, numerous researchers have tried to find medicinal plants which have pharmacological potentials for therapeutic applications in cerebral ischemia, and many studies in vivo and in vitro have reported that extracts from various medicinal plants and their components show therapeutic effects against cerebral ischemia [81718]. However, therapeutic effects of PTE against tGCI has not yet been investigated. Therefore, in the present study, we examined neuroprotective effects of PTE in the gerbil hippocampal CA1 subjected to 5 minutes of tGCI and found that many of pyramidal neurons survived in the ischemic CA1 of gerbils pre-treated with 200 mg/kg PTE when we examined the CA1 pyramidal cells using CV staining and NeuN immunohistochemistry, which are universally used staining techniques for examining neuronal death in the brain [119]. This finding is the first in proving neuroprotective effects of the P. tomentiglandulosa against tGCI.
It has been well demonstrated that cerebral ischemia causes reactive gliosis of astrocytes and microglia and that reactive astrocytes and microglia are involved in cerebral ischemia-induced neuronal death through production and release of neurotoxic molecules and pro-inflammatory cytokines [2021]. Therefore, it has been thought that inhibiting reactive astrocytes and microglia is considered to protect neurons from cerebral ischemic insults [2223]. In this study, we examined reactive gliosis of astrocytes and microglia in the ischemic CA1 after pretreatment with PTE via GFAP and Iba-1 immunohistochemistry, respectively. Immunohistochemical results showed that tGCI-induced reactive gliosis of astrocytes and microglia was significantly diminished by pretreatment with 200 mg/kg of PTE. Although there were no studies regarding effects of Populus species on the reactive gliosis induced by cerebral ischemic insults, it was reported that extracts of some species such as P. davidiana and Populus maximowiczii strongly inhibited production of nitric oxide, which is considered as a pro-inflammatory mediator, in lipopolysaccharides (LPS)-stimulated macrophages [24]. In addition, a recent study demonstrated that salicortin isolated from Populus euramericana extract significantly suppressed expressions of pro-inflammatory cytokines, such as tumor necrosis factor-α, interleukin (IL)-1β and IL-6 in macrophages stimulated by LPS [25]. Therefore, it is likely that the inhibition of tGCI-induced reactive gliosis in the ischemic CA1 by PTE pretreatment might be closely associated with neuroprotection of PTE against tGCI.
In brief, the results of this study obviously show that pretreatment with PTE can protect CA1 pyramidal neurons from ischemic injury following tGCI and that its neuroprotective effect is closely related to attenuation of reactive gliosis. Therefore, our recent study suggests that P. tomentiglandulosa can be a promising candidate for prevention of cerebral ischemia-induced neuronal damage/death, although further researches are positively necessary to explain neuroprotective mechanisms of P. tomentiglandulosa against tGCI.
Figures and Tables
Fig. 1
CV staining in the hippocampus of the vehicle-sham (A, B), PTE-sham (E, F, I, J), vehicle-tGCI (C, D), and PTE-tGCI (G, H, K, L) groups 5 days after tGCI. Pyramidal cells in the SP (black asterisks) of the CA1 are hardly stained with CV in the vehicle-tGCI group. In the 200 mg/kg PTE-tGCI group, CA1 pyramidal cells (white asterisks) are well stained with CV. CV, cresyl violet; PTE, Populus tomentiglandulosa extract; tCGI, transient global cerebral ischemia; SP, stratum pyramidale; CA, cornu ammonis; DG, dentate gyrus; SO, stratum oriens; SR, stratum radiatum. Scale bars=400 µm (A, C, E, G, I, K), 40 µm (B, D, F, H, J, L).
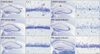
Fig. 2
NeuN immunohistochemistry in the CA1 area of the vehicle-sham (A), PTE-sham (B, C), vehicle-tGCI (D), and PTE-tGCI (E, F) groups 5 days after tGCI. In all sham groups, CA1 pyramidal cells show strong NeuN immunoreactivity; however, a few NeuN+ cells (arrows) are detected in the vehicle-tGCI group. In the 200 mg/kg PTE-tGCI group, many NeuN+ cells are observed in the SP (asterisk). NeuN, neuronal nuclear antigen; CA, cornu ammonis; PTE, Populus tomentiglandulosa extract; tCGI, transient global cerebral ischemia; SP, stratum pyramidale; SO, stratum oriens; SR, stratum radiatum. Scale bar=40 µm. (G) The mean number of NeuN+ cells in the CA1 5 days after tGCI (n=7 in each group, *P<0.05 vs. vehicle-sham group, #P<0.05 vs. vehicle-tGCI group). The bars indicate the means±SEM.
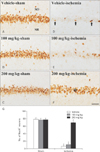
Fig. 3
Immunohistochemistry for GFAP in the CA1 area of the vehicle-sham (A), PTE-sham (B, C), vehicle-tGCI (D), and PTE-tGCI (E, F) groups 5 days after tGCI. No differences in the distribution and morphology of GFAP+ astrocytes are shown between all sham groups. In the vehicle-tGCI group, GFAP+ astrocytes (arrows) show hypertrophic morphology. However, in the 200 mg/kg PTE-tGCI group, GFAP+ astrocytes are less hypertrophied. GFAP, glial fibrillary acidic protein; CA, cornu ammonis; PTE, Populus tomentiglandulosa extract; tCGI, transient global cerebral ischemia; ROD, relative optical density; SO, stratum oriens; SP, stratum pyramidale; SR, stratum radiatum. Scale bar=40 µm. (G) ROD as % of GFAP+ structures in the CA 5 days after tGCI (n=7 in each group, *P<0.05 vs. vehicle-sham group, #P<0.05 vs. vehicle-ischemia group). The bars indicate the means±SEM.
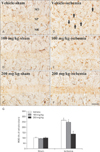
Fig. 4
Immunohistochemistry for Iba-1 in the CA1 area of the vehicle-sham (A), PTE-sham (B, C), vehicle-tGCI (D), and PTE-tGCI (E, F) groups 5 days after tGCI. In the vehicle-tGCI group, Iba-1+ microglia show large cytoplasm with thick processes, and many microglia are concentrated in the SP (asterisk). In the 200 mg/kg PTE-tGCI group, the distribution and morphology of Iba-1+ microglia are similar to those in the sham group. Iba-1, ionized calcium-binding adapter molecule 1; CA, cornu ammonis; PTE, Populus tomentiglandulosa extract; tCGI, transient global cerebral ischemia; SP, stratum pyramidale; ROD, relative optical density; SO, stratum oriens; SR, stratum radiatum. Scale bar=40 µm. (G) ROD as % of Iba-1+ structures in the CA1 at 5 days post-tGCI (n=7 in each group, *P<0.05 vs. vehicle-sham group, #P<0.05 vs. vehicle-tGCI group). The bars indicate the means±SEM.
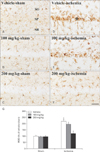
Acknowledgements
This work was supported by the Bio-Synergy Research Project (NRF-2015M3A9C4076322) of the Ministry of Science, ICT and Future Planning through the National Research Foundation, and by Basic Science Research Program through the National Research Foundation of Korea (NRF) funded by the Ministry of Education (NRF-2017R1D1A1B03033271).
References
1. Park JH, Kim YH, Ahn JH, Choi SY, Hong S, Kim SK, Kang IJ, Kim YM, Lee TK, Won MH, Lee CH. Atomoxetine protects against NMDA receptor-mediated hippocampal neuronal death following transient global cerebral ischemia. Curr Neurovasc Res. 2017; 14:158–168.
2. Spray S, Edvinsson L. Improved assessment of outcomes following transient global cerebral ischemia in mice. Exp Brain Res. 2016; 234:1925–1934.
3. Globus MY, Busto R, Martinez E, Valdés I, Dietrich WD, Ginsberg MD. Comparative effect of transient global ischemia on extracellular levels of glutamate, glycine, and gamma-aminobutyric acid in vulnerable and nonvulnerable brain regions in the rat. J Neurochem. 1991; 57:470–478.
4. Lee JC, Park JH, Kim IH, Cho GS, Ahn JH, Tae HJ, Choi SY, Cho JH, Kim DW, Kwon YG, Kang IJ, Won MH, Kim YM. Neuroprotection of ischemic preconditioning is mediated by thioredoxin 2 in the hippocampal CA1 region following a subsequent transient cerebral ischemia. Brain Pathol. 2017; 27:276–291.
5. Ye M, Yang W, Ainscough JF, Hu XP, Li X, Sedo A, Zhang XH, Zhang X, Chen Z, Li XM, Beech DJ, Sivaprasadarao A, Luo JH, Jiang LH. TRPM2 channel deficiency prevents delayed cytosolic Zn2+ accumulation and CA1 pyramidal neuronal death after transient global ischemia. Cell Death Dis. 2014; 5:e1541.
6. Chun HS, Kim JM, Choi EH, Chang N. Neuroprotective effects of several korean medicinal plants traditionally used for stroke remedy. J Med Food. 2008; 11:246–251.
7. Park JH, Cho JH, Kim IH, Ahn JH, Lee JC, Chen BH, Shin BN, Tae HJ, Yoo KY, Hong S, Kang IJ, Won MH, Kim JD. Oenanthe javanica extract protects against experimentally induced ischemic neuronal damage via its antioxidant effects. Chin Med J (Engl). 2015; 128:2932–2937.
8. Shi X, Yu W, Yang T, Liu W, Zhao Y, Sun Y, Chai L, Gao Y, Dong B, Zhu L. Panax notoginseng saponins provide neuroprotection by regulating NgR1/RhoA/ROCK2 pathway expression, in vitro and in vivo. J Ethnopharmacol. 2016; 190:301–312.
9. Bruch G, Wirth EH. Studies on poplar bud. J Pharm Sci. 1936; 25:672–682.
10. Bae KH. The medicinal plants of Korea. Seoul: Kyo-Hak Publishing Co.;1999. p. 98.
11. Debbache-Benaida N, Atmani-Kilani D, Schini-Keirth VB, Djebbli N, Atmani D. Pharmacological potential of Populus nigra extract as antioxidant, anti-inflammatory, cardiovascular and hepatoprotective agent. Asian Pac J Trop Biomed. 2013; 3:697–704.
12. Zhang X, Thuong PT, Min BS, Ngoc TM, Hung TM, Lee IS, Na M, Seong YH, Song KS, Bae K. Phenolic glycosides with anti-oxidant activity from the stem bark of Populus davidiana. J Nat Prod. 2006; 69:1370–1373.
13. Harbilas D, Brault A, Vallerand D, Martineau LC, Saleem A, Arnason JT, Musallam L, Haddad PS. Populus balsamifera L. (Salicaceae) mitigates the development of obesity and improves insulin sensitivity in a diet-induced obese mouse model. J Ethnopharmacol. 2012; 141:1012–1020.
14. Karpiak SE, Tagliavia A, Wakade CG. Animal models for the study of drugs in ischemic stroke. Annu Rev Pharmacol Toxicol. 1989; 29:403–414.
15. Lee JC, Park JH, Ahn JH, Kim IH, Cho JH, Choi JH, Yoo KY, Lee CH, Hwang IK, Cho JH, Kwon YG, Kim YM, Kang IJ, Won MH. New GABAergic neurogenesis in the hippocampal CA1 region of a gerbil mdel of long-term survival after transient cerebral ischemic injury. Brain Pathol. 2016; 26:581–592.
16. Park JH, Shin BN, Chen BH, Kim IH, Ahn JH, Cho JH, Tae HJ, Lee JC, Lee CH, Kim YM, Lee YL, Kim SK, Won MH. Neuroprotection and reduced gliosis by atomoxetine pretreatment in a gerbil model of transient cerebral ischemia. J Neurol Sci. 2015; 359:373–380.
17. Gong J, Sun F, Li Y, Zhou X, Duan Z, Duan F, Zhao L, Chen H, Qi S, Shen J. Momordica charantia polysaccharides could protect against cerebral ischemia/reperfusion injury through inhibiting oxidative stress mediated c-Jun N-terminal kinase 3 signaling pathway. Neuropharmacology. 2015; 91:123–134.
18. Zhang K, Li YJ, Yang Q, Gerile O, Yang L, Li XB, Guo YY, Zhang N, Feng B, Liu SB, Zhao MG. Neuroprotective effects of oxymatrine against excitotoxicity partially through down-regulation of NR2B-containing NMDA receptors. Phytomedicine. 2013; 20:343–350.
19. Golub VM, Brewer J, Wu X, Kuruba R, Short J, Manchi M, Swonke M, Younus I, Reddy DS. Neurostereology protocol for unbiased quantification of neuronal injury and neurodegeneration. Front Aging Neurosci. 2015; 7:196.
20. Giulian D, Vaca K. Inflammatory glia mediate delayed neuronal damage after ischemia in the central nervous system. Stroke. 1993; 24:12 Suppl. I84–I90.
21. Wang Y, Ge P, Yang L, Wu C, Zha H, Luo T, Zhu Y. Protection of ischemic post conditioning against transient focal ischemia-induced brain damage is associated with inhibition of neuroinflammation via modulation of TLR2 and TLR4 pathways. J Neuroinflammation. 2014; 11:15.
22. Pérez-Álvarez MJ, Maza Mdel C, Anton M, Ordoñez L, Wandosell F. Post-ischemic estradiol treatment reduced glial response and triggers distinct cortical and hippocampal signaling in a rat model of cerebral ischemia. J Neuroinflammation. 2012; 9:157.
23. Zhang H, Park JH, Maharjan S, Park JA, Choi KS, Park H, Jeong Y, Ahn JH, Kim IH, Lee JC, Cho JH, Lee IK, Lee CH, Hwang IK, Kim YM, Suh YG, Won MH, Kwon YG. Sac-1004, a vascular leakage blocker, reduces cerebral ischemia-reperfusion injury by suppressing blood-brain barrier disruption and inflammation. J Neuroinflammation. 2017; 14:122.
24. Ryu JH, Ahn H, Kim JY, Kim YK. Inhibitory activity of plant extracts on nitric oxide synthesis in LPS-activated macrophages. Phytother Res. 2003; 17:485–489.
25. Kwon DJ, Bae YS, Ju SM, Youn GS, Choi SY, Park J. Salicortin suppresses lipopolysaccharide-stimulated inflammatory responses via blockade of NF-kappaB and JNK activation in RAW 264.7 macrophages. BMB Rep. 2014; 47:318–323.