Abstract
In an attempt to clarify the function of the suboccipital muscles, we performed morphological observation of the suboccipital muscles for variations in the muscle belly and compared the morphology of their muscle fibers in terms of cross-sectional area by immunostaining with anti-myosin heavy chain antibodies. The cadavers of 25 Japanese individuals were used: 22 for morphological examinations and three for histological examinations. Among samples of the rectus capitis posterior major muscle (RCPma) and rectus capitis posterior minor muscle (RCPmi), 86.4% had a typical muscle appearance with a single belly, and 13.6% had an anomalous morphology. None of the samples of the obliquus capitis superior (OCS) or obliquus capitis inferior (OCI) muscles had an anomalous appearance. Measurement of cross-sectional area revealed that fast-twitch muscle fibers in the RCPma and OCI had a significantly greater cross-sectional area than those of the RCPmi and OCS. The cross-sectional area of intermediate muscle fibers was also significantly greater in the OCS than in the RCPma, RCPmi, and OCI. The cross-sectional area of slow-twitch muscle fibers was significantly greater in the OCS than in the RCPma, RCPmi, and OCI, and the RCPmi showed a significantly greater cross-sectional area for slow-twitch muscle fibers than did the RCPma, and OCI. Our findings indicate that the RCPmi and OCS exert a greater force than the RCPma and OCI, and act as anti-gravity agonist muscles of the head. Prolonged head extension in individuals with anomalous suboccipital muscle groups could result in dysfunction due to undue stress.
The suboccipital muscles are located in the deepest layer of the neck, comprising the four paired muscles of the rectus capitis posterior major (RCPma), the rectus capitis posterior minor (RCPmi), the obliquus capitis superior (OCS), and the obliquus capitis inferior (OCI) muscles. Unilateral contraction of these muscles results in head rotation, whereas bilateral contraction results in head extension [1]. An association between the suboccipital muscles and headache has recently been pointed out, and McPartland et al. [2] reported that atrophy of the suboccipital muscles following whiplash is involved in marked, chronic neck pain and reduced standing balance. Malformation, defects, and anomalies of the suboccipital muscles, including the presence of accessory muscles, might also affect head and neck pain [3456]. In Japan, Mori [7] have reported variations of the suboccipital muscles, but few studies have examined these muscle groups morphologically, and therefore many morphological aspects of these muscles remain unclear.
Some studies have shed light on the muscle fiber characteristics of skeletal muscles using cell biology techniques [8910]. Myosin heavy chain (MHC) is a contractile protein with the largest mass of all such proteins, and is known to directly reflect the characteristics of muscles [11]. Depending on their rate of contraction, muscle fibers are classified as fast-twitch, intermediate, and slow-twitch types [12]. Sartorius et al. [13] reported that skeletal muscles in myosin heavy chain-IId-null mice show increased levels of myosin heavy chain-IIa (MHC-IIa). Yanagisawa et al. [14] have also reported that the presence of MHC-IIa after weaning in microphthalmic (mi/mi) mice with no tooth eruption was attributable to compensation for a lack of proper masticatory function and sucking-like movements, as MHC-IIa is necessary for these movements. In other words, muscle fibers change according to muscle function and structure, and therefore an understanding of the composition of MHC is important when considering the function of the suboccipital muscles.
The present study investigated: (1) morphological variations in the suboccipital muscles, and (2) the muscle fiber characteristics of these muscle groups. On the basis of our findings, we also discussed the role of the suboccipital muscles.
This study was performed in accordance with the provisions of the Declaration of Helsinki 1995 (as revised in Edinburgh 2000) and was approved by the Human Research Ethics Committee at the dental school. We dissected 25 cadavers donated for the annual student dissection courses at Tokyo Dental College (14 males, 11 females; mean age 80 years at death; range, 53–97 years). The cause of death in each case had been ischemic heart failure. All cadavers had been donated to Tokyo Dental College for research and education on human anatomy, and their use for research did not require approval by the university ethics committee.
Forty-four muscle specimens (22 cadavers) had been fixed by intravenous injection of non-neutralized 10% (v/v) formalin solution and preserved in 50% ethanol solution for more than 3 months. Morphological observation of the suboccipital muscles for variations in the muscle belly was performed. The RCPma, RCPmi, OCS, and OCI were dissected under a magnifying glass after resecting the trapezius, splenius capitis and semispinalis capitis muscles. Variations in the muscle bellies of the suboccipital muscles were classified into different types by macroscopic observation.
The remaining six muscle specimens (3 cadavers) were those of unfixed fresh cadavers, all of the individuals having died within the previous 3 days. These specimens were chosen as being representative of normal morphology because they showed no deformation or damage to the cervical spinal joints and no positional anomalies in the surrounding muscles beyond the area of observation. A square tissue slice measuring approximately 10 mm on all sides was harvested from the vicinity of the insertion of the suboccipital muscles and mounted on a piece of cork to ensure that the direction of the muscle fiber bundles was vertical. The tissue samples were then rapidly frozen in isopentane solution (−160℃) cooled with liquid nitrogen, and thereafter cryopreserved at −80℃ until use in experiments. Samples were prepared using a CM 3000 cryostat (Leica Microsystems, Wetzlar, Germany) to create 10-µm-thick serial sections on a plane perpendicular to the muscle fibers. The obtained serial sections were then subjected to immunostaining using mouse monoclonal anti-myosin heavy chain fast (MHCf) antibody (Abcam plc., Cambridge, UK) and mouse monoclonal anti-myosin heavy chain slow (MHCs) antibody (Sigma-Aldrich, St. Louis, MO, USA) as the primary antibody, and fluorescein isothiocyanate-donkey anti-mouse IgG antibody (Jackson Immuno Research, West Grove, PA, USA) as the secondary antibody. Slides were observed using an Axiophot 2 polarizing light microscope (Carl Zeiss, Jena, Germany) and the approximate center of the tissue sections was observed and photographed at ×100 magnification. Immunohistochemical examinations were performed using the method described by Doi et al. [9] for objective evaluation. Muscle fibers stained with anti-MHCf antibodies were designated as fast-twitch muscle fibers, those stained with anti-MHCs antibodies as slow-twitch muscle fibers, and those showing positive staining with both antibodies as intermediate fibers. Mean cross-sectional area (CSA) of muscle fibers per square 500 mm was determined using Image-Pro Plus software (Media Cybernetics, Rockville, MD, USA) (Fig. 1).
Macroscopic morphological observation revealed the following points of origin and insertion of each of the suboccipital muscles. The RCPma showed a tendinous origin from the spinous process of the second cervical vertebra and a tendinous insertion into the inferior nuchal line of the occipital bone. The RCPmi had a tendinous origin from the posterior tubercle of the first cervical vertebra and a tendinous insertion into the inferior nuchal line close to the occipital bone midline. The OCS displayed a tendinous origin from the transverse process of the first cervical vertebra and a tendinous insertion into the distal end of the inferior nuchal line of the occipital bone. The OCI had a tendinous origin from the spinous process of the second cervical vertebra and a tendinous insertion into the transverse process of the first cervical vertebra. As anomalous anatomy was evident for both the RCPmi and the RCPma, these muscles were classified into five types.
(1) Type I: The muscle is formed of muscle fiber bundles exhibiting a typical form of attachment and all muscle bellies form a single belly. Muscle fiber bundles have a tendinous origin and insertion (Fig. 2A) The RCPma and RCPmi were of this type in 38/44 (86.4%) and 38/44 (86.4%) of specimens, respectively (Table 1).
(2) Type II: All the muscle at the origin is consistent with typical muscle (Type I), but the insertion consists of a tendinous attachment to two adjacent locations, and two muscle bellies are present (Fig. 2B). A tendency for a wider area of attachment than the insertion of type I muscle is evident. The RCPma and RCPmi were of this type in 2/44 (4.5%) and 1/44 (2.3%) of specimens, respectively (Table 1).
(3) Type III: The origin is at the spinous process of the second cervical vertebra in the RCPma and at the posterior tubercle of the first cervical vertebra in the RCPmi. The insertion is at the inferior nuchal line of the occipital bone in both the RCPma and the RCPmi. While all muscle at the origin is consistent with typical muscle, the insertion consists of tendinous attachments to three adjacent locations, and three muscle bellies are present (Fig. 2B). A tendency for a wider area of attachment than the insertions of types I and II muscle is also evident. Type III muscle was seen in 1/44 (2.3%) of RCPma samples, but not in any of the RCPmi samples (Table 1).
(4) Type IV: The muscle belly is completely missing and the muscle is filled with fat tissue in its place (Fig. 2C). Two (4.5%) of the 44 RCPmi samples were classified as this type. Bilateral insertions of the RCPma in type IV are attached closer to the midline than in other samples, and the width of the muscle belly is greater. No defects of the muscle belly were seen in the RCPma (Table 1).
(5) Type V: Fusion of the muscle bellies is seen between the RCPma and RCPmi. The origin of the fused muscle bellies is at the posterior tubercle of the first cervical vertebra, matching the origin of the RCPmi. The insertion is at the inferior nuchal line of the occipital bone, consistent with the insertion of the RCPma (Fig. 2D). The RCPma and RCPmi were of this muscle type in 3/44 (6.8%) and 3/44 (6.8%) of specimens, respectively (Table 1).
The case of RCPmi defect was bilateral (one cadaver; two samples). In one case, the anomalous muscle was located in the midline, where it originated from the spinous process of the second cervical vertebra and terminated on the inferior nuchal line of the occipital bone (Fig. 3). No anomalous muscle was seen in the OCS or OCI, which appeared as a typical muscle in all samples (Table 1). Features such as fat tissue, nerves, and blood vessels were seen between the muscle bellies of anomalous muscles.
A comparison of the CSA of fast- and slow-twitch muscle fibers revealed that the CSA of the latter was at least two-fold greater than that of the former in RCPmi OCS (Table 2, Fig. 4). We were demonstrated no right-left difference (Table 3). The RCPma displayed a significantly greater CSA of fast-twitch muscle fibers than the RCPmi, and the OCI had a significantly greater CSA of fast-twitch muscle fibers than the RCPma. Meanwhile, the OCS had a significantly different CSA for intermediate fibers than the RCPma, RCPmi, and OCI. A comparison of the CSAs of slow-twitch muscle fibers revealed a significantly greater CSA in the OCS than in the other three muscles, and the RCPmi had a significantly greater CSA of slow-twitch muscle fibers than the RCPma and OCI (Fig. 5).
The spine is generally surrounded by deep muscle and ligaments, but the atlanto-occipital and atlanto-axial joints contain no articular discs or interspinous ligaments [1516]. A likely reason for this is to enable highly flexible movement of the head instead of strong intervertebral immobilization. This suggests that the atlanto-occipital joint is controlled by the surrounding tissue to functionally maintain stability. The present study showed that slow-twitch muscle fibers have a CSA approximately greater than that of fast-twitch muscle fibers in all suboccipital muscles. Furthermore, these suboccipital muscles have a high density of muscle spindles, which, in addition to allowing flexible movement, act as specific sensory receptors [17]. The suboccipital muscles therefore act to functionally maintain the stability of the head while allowing delicate control of movement of the atlanto-occipital and atlanto-axial joints with a weak, sustained force.
Kamibayashi and Richmond [18] reported that the CSAs of the neck muscles were proportional to the height and weight of the individual. Furthermore, Ikai and Fukunaga [19] reported that muscle strength was proportional to the CSA of muscle fibers. Meanwhile, Rantanen et al. [20] demonstrated that no major age-related changes occur in the CSA of muscle fibers as a result of changes in the muscles of the erector spinae. The results for muscle fiber CSAs obtained in this study indicated that the RCPmi and OCS might provide more force than the RCPma and OCI, suggesting their potential roles as anti-gravity agonist muscles of the head.
This study also revealed variations in the number of muscle bellies of the suboccipital muscles, which typically have only one muscle belly. Moreover, anomalous muscle was observed in 13.6% of RCPma and RCPmi samples, but was not observed at all in the OCS or OCI. Comparisons of the incidence of anomalous muscles in this study with that found by Mori [7] are presented in Tables 4 and 5. The presence of three muscle bellies was not described at all by Mori, but this feature was seen in 2.3% of the RCPma samples in our study, suggesting a large variation in the type of muscle anomaly. The incidence of two muscle bellies in the RCPmi in our study was about 21% lower, but the rate of muscle belly defects was about 1% higher. The incidence of typical muscle was also about 26% higher, revealing many cases of typical and missing muscle in the RCPmi. Katori et al. [21] reported that the mode of travel of structures such as nerves and blood vessels influences variations in the muscle belly. Because we observed features such as fat tissue, nerves and blood vessels between muscle bellies, the influence of embryological factors also seems likely.
Many previous studies have suggested that the suboccipital muscles are associated with chronic head and neck pain [2223]. Andary et al. [24] pointed out that atrophy of the suboccipital muscles is associated with chronic neck pain. Evaluation of the RCPmi by magnetic resonance imaging has shown that subjects with atrophied suboccipital muscles experience twice as much cervical vertebral dysfunction as control subjects [25]. Furthermore, in their clinical studies of chronic neck pain, Elliott et al. [262728] stated that fat tissue infiltrates the RCPmi as a result of damage to suboccipital muscle tissue. The pattern of the RCPmi defect in this study resembled that reported by Elliott et al. [262728]. Moreover, the RCPma was notably large in comparison with the other muscles in cases of type IV muscle defect, suggesting that the RCPma acted to compensate for RCPmi defects.
Mild neck pain can worsen when affected individuals have to work with visual display terminals (VDTs). Caution is therefore required with VDT work [29]. Individuals with anomalous suboccipital muscles could develop dysfunction if they are subjected to undue stress resulting from prolonged head extension.
Acknowledgements
We are grateful to the individuals who donated their bodies after death to Tokyo Dental College for research and education on human anatomy without any economic benefit. We also thank their families for agreeing to the donation as well as their patience in waiting for the return of their remains after study.
References
1. Hiatt JL, Gartner LP. Textbook of head and neck anatomy. 2nd ed. Baltimore, MD: Williams & Wilkins;1987. p. 109–122.
2. McPartland JM, Brodeur RR, Hallgren RC. Chronic neck pain, standing balance, and suboccipital muscle atrophy: a pilot study. J Manipulative Physiol Ther. 1997; 20:24–29. PMID: 9004119.
3. Loukas M, Tubbs RS. An accessory muscle within the suboccipital triangle. Clin Anat. 2007; 20:962–963. PMID: 17415745.
4. Tagil SM, Ozçakar L, Bozkurt MC. Insight into understanding the anatomical and clinical aspects of supernumerary rectus capitis posterior muscles. Clin Anat. 2005; 18:373–375. PMID: 15971221.
5. Nayak SR, Swamy R, Krishnamurthy A, Dasgupta H. Bilateral anomaly of rectus capitis posterior muscles in the suboccipital triangle and its clinical implication. Clin Ter. 2011; 162:355–356. PMID: 21912824.
6. Bergmann RA, Thompson SA, Afifi AK, Saadeh FA. Compendium of human anatomic variation. Baltimore, MD: Urban & Schwarzennberg;1988. p. 33–34.
7. Mori M. Statistics on the Musculature of the Japanese. Okajimas Folia Anat Jpn. 1964; 40:195–300. PMID: 14213705.
8. Abe S, Maejima M, Watanabe H, Shibahara T, Agematsu H, Doi T, Sakiyama K, Usami A, Gojyo K, Hashimoto M, Yoshinari M, Ide Y. Muscle-fiber characteristics in adult mouse-tongue muscles. Anat Sci Int. 2002; 77:145–148. PMID: 12418096.
9. Doi T, Abe S, Ide Y. Masticatory function and properties of masseter muscle fibers in microphthalmic (mi/mi) mice during postnatal development. Ann Anat. 2003; 185:435–440. PMID: 14575270.
10. Feng X, Zhang T, Xu Z, Choi SJ, Qian J, Furdui CM, Register TC, Delbono O. Myosin heavy chain isoform expression in the Vastus Lateralis muscle of aging African green vervet monkeys. Exp Gerontol. 2012; 47:601–607. PMID: 22617406.
11. Pette D, Staron RS. Cellular and molecular diversities of mammalian skeletal muscle fibers. Rev Physiol Biochem Pharmacol. 1990; 116:1–76. PMID: 2149884.
12. Henneman E, Olson CB. Relations between structure and function in the design of skeletal muscles. J Neurophysiol. 1965; 28:581–598. PMID: 14328455.
13. Sartorius CA, Lu BD, Acakpo-Satchivi L, Jacobsen RP, Byrnes WC, Leinwand LA. Myosin heavy chains IIa and IId are functionally distinct in the mouse. J Cell Biol. 1998; 141:943–953. PMID: 9585413.
14. Yanagisawa N, Abe S, Agematsu H, Sakiyama K, Usami A, Tamatsu Y, Ide Y. Myosin heavy chain composition of tongue muscle in microphthalmic (mi/mi) mice before and after weaning. Ann Anat. 2006; 188:329–336. PMID: 16856597.
15. Chang H, Gilbertson LG, Goel VK, Winterbottom JM, Clark CR, Patwardhan A. Dynamic response of the occipito-atlanto-axial (C0-C1-C2) complex in right axial rotation. J Orthop Res. 1992; 10:446–453. PMID: 1569507.
16. Johnson GM, Zhang M, Jones DG. The fine connective tissue architecture of the human ligamentum nuchae. Spine (Phila Pa 1976). 2000; 25:5–9. PMID: 10647153.
17. Kulkarni V, Chandy MJ, Babu KS. Quantitative study of muscle spindles in suboccipital muscles of human foetuses. Neurol India. 2001; 49:355–359. PMID: 11799407.
18. Kamibayashi LK, Richmond FJ. Morphometry of human neck muscles. Spine (Phila Pa 1976). 1998; 23:1314–1323. PMID: 9654620.
19. Ikai M, Fukunaga T. Calculation of muscle strength per unit cross-sectional area of human muscle by means of ultrasonic measurement. Int Z Angew Physiol. 1968; 26:26–32. PMID: 5700894.
20. Rantanen J, Rissanen A, Kalimo H. Lumbar muscle fiber size and type distribution in normal subjects. Eur Spine J. 1994; 3:331–335. PMID: 7532536.
21. Katori Y, Yamamoto M, Asakawa S, Maki H, Rodríguez-Vázquez JF, Murakami G, Abe S. Fetal developmental change in topographical relationship between the human lateral pterygoid muscle and buccal nerve. J Anat. 2012; 220:384–395. PMID: 22352373.
22. Alix ME, Bates DK. A proposed etiology of cervicogenic headache: the neurophysiologic basis and anatomic relationship between the dura mater and the rectus posterior capitis minor muscle. J Manipulative Physiol Ther. 1999; 22:534–539. PMID: 10543584.
23. Fernández-de-Las-Peñas C, Cuadrado ML, Arendt-Nielsen L, Ge HY, Pareja JA. Association of cross-sectional area of the rectus capitis posterior minor muscle with active trigger points in chronic tension-type headache: a pilot study. Am J Phys Med Rehabil. 2008; 87:197–203. PMID: 18174844.
24. Andary MT, Hallgren RC, Greenman PE, Rechtien JJ. Neurogenic atrophy of suboccipital muscles after a cervical injury: a case study. Am J Phys Med Rehabil. 1998; 77:545–549. PMID: 9862543.
25. McPartland JM, Brodeur RR. Rectus capitis posterior minor: a small but important suboccipital muscle. J Bodyw Mov Ther. 1999; 3:30–35.
26. Elliott JM, Galloway GJ, Jull GA, Noteboom JT, Centeno CJ, Gibbon WW. Magnetic resonance imaging analysis of the upper cervical spine extensor musculature in an asymptomatic cohort: an index of fat within muscle. Clin Radiol. 2005; 60:355–363. PMID: 15710139.
27. Elliott J, Jull G, Noteboom JT, Darnell R, Galloway G, Gibbon WW. Fatty infiltration in the cervical extensor muscles in persistent whiplash-associated disorders: a magnetic resonance imaging analysis. Spine (Phila Pa 1976). 2006; 31:E847–E855. PMID: 17047533.
28. Elliott J, Sterling M, Noteboom JT, Darnell R, Galloway G, Jull G. Fatty infiltrate in the cervical extensor muscles is not a feature of chronic, insidious-onset neck pain. Clin Radiol. 2008; 63:681–687. PMID: 18455560.
29. Jang JH, Kim TH, Oh JS. Effects of visual display terminal works on cervical movement pattern in patients with neck pain. J Phys Ther Sci. 2014; 26:1031–1032. PMID: 25140089.
Fig. 1
Enlarged schematic diagram of a measurement square. Muscle fibers inside four 500-µm2 were analyzed. Fibers touching the upper horizontal line and left vertical line were excluded.
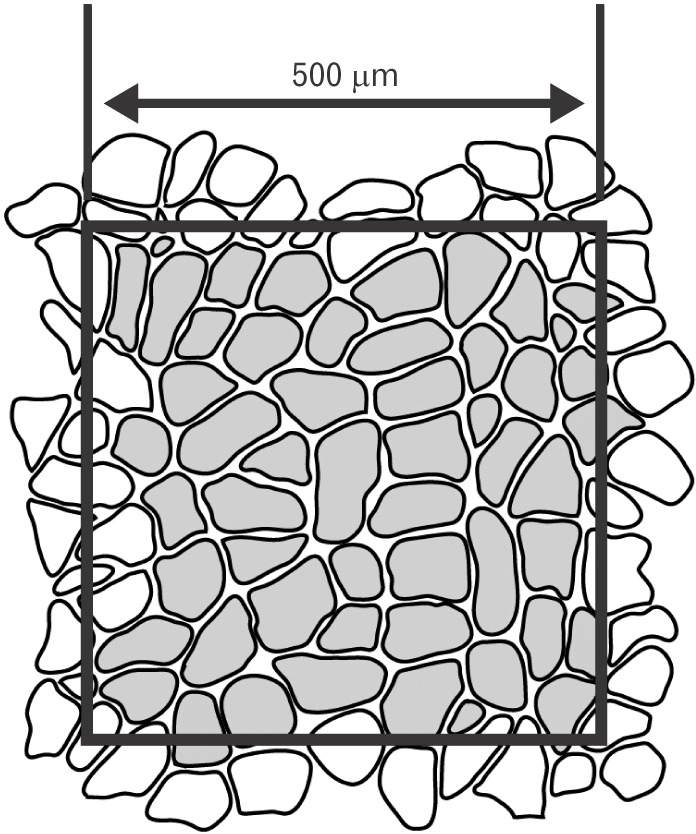
Fig. 2
Morphological variation in the suboccipital muscles. (A) Type I: Muscle fiber bundles exhibiting a typical form of attachment. (B) Type II: Attached muscle fiber bundles with two muscle bellies (arrow). Type III: Attached muscle fiber bundles with three muscle bellies (asterisk). (C) Type IV: The muscle belly is missing (asterisk). (D) Type V: Muscle fiber bundles where the muscle belly has fused with another muscle belly (asterisk). RCPma, rectus capitis posterior major muscle; RCPmi, rectus capitis posterior minor muscle; OCS, obliquus capitis superior; OCI, obliquus capitis inferior.
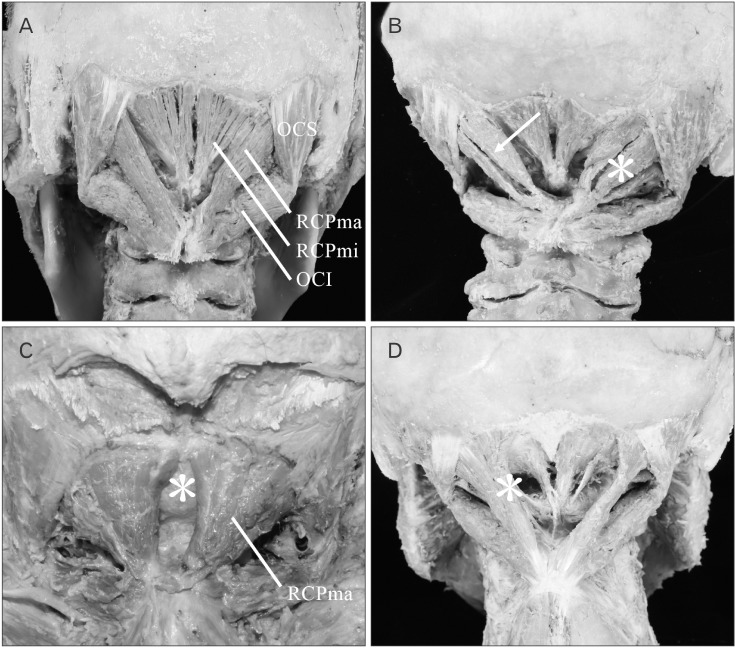
Fig. 3
An accessory muscle to the suboccipital muscles (asterisk). This anomalous muscle is located in the midline, where it originates from the spinous process of the second cervical vertebra and terminates at the inferior nuchal line of the occipital bone.
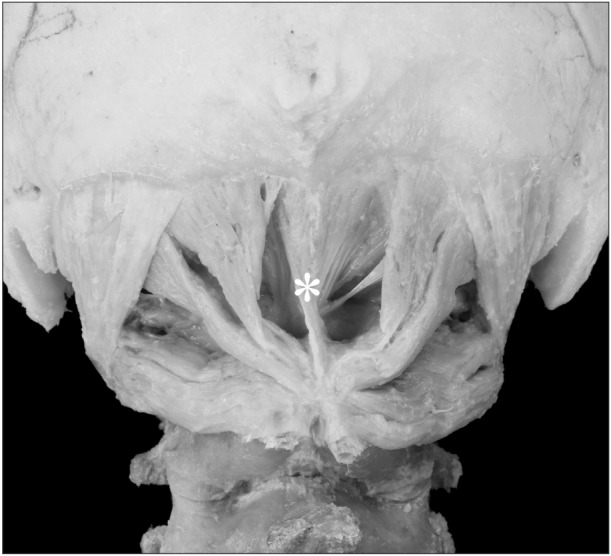
Fig. 4
Immunohistochemical staining using anti-myosin heavy chain fast (MHCf) antibodies and anti-myosin heavy chain slow (MHCs) antibodies. (A, C, E, G) Images of anti-MHCf antibody staining. (B, D, F, H) Images of anti-MHCs antibody staining. (A, B) Stained rectus capitis posterior major muscle. (C, D) Stained rectus capitis posterior minor muscle. (E, F) Stained obliquus capitis superior. (G, H) Stained obliquus capitis inferior. White arrow, black arrowhead, and black arrow indicate fast-twitch muscle fibers, intermediate muscle fibers, and slow-twitch muscle fibers. Scale bars=100 µm (A–H).
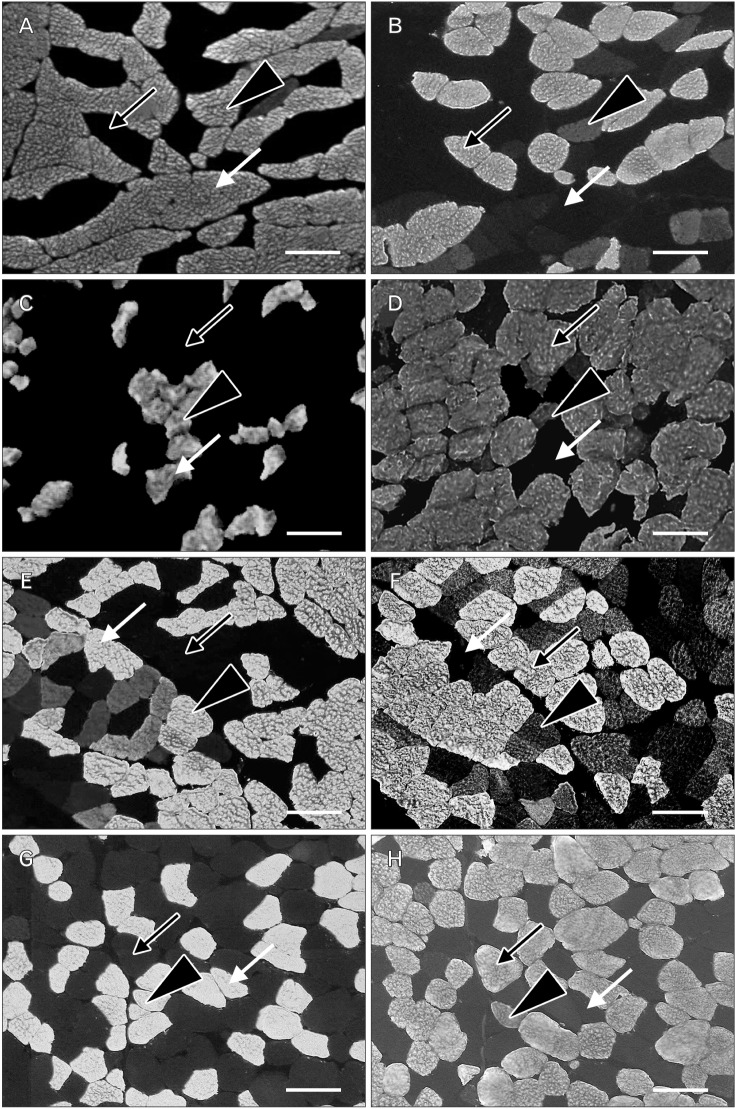
Fig. 5
Comparison of cross-sectional areas (CSAs) of muscle fibers. (A) Comparison of CSAs of fast-twitch muscle fibers. The rectus capitis posterior major muscle (RCPma) had a significantly greater CSA for fast-twitch muscle fibers than did the rectus capitis posterior minor muscle (RCPmi) and obliquus capitis superior (OCS). The obliquus capitis inferior (OCI) had a significantly greater CSA for fast-twitch muscle fibers than did the RCPmi and OCS. (B) Comparison of CSAs of intermediate muscle fibers. The OCS had a significantly greater CSA for intermediate fibers than did the RCPma, RCPmi, and OCI. (C) Comparison of CSAs of slow-twitch muscle fibers. The RCPmi showed a significantly greater CSA of slow-twitch muscle fibers compared to the RCPma and OCI. The OCS showed a significantly greater CSA of slow-twitch muscle fibers compared to the RCPma, RCPmi, and OCI. *P<0.05.
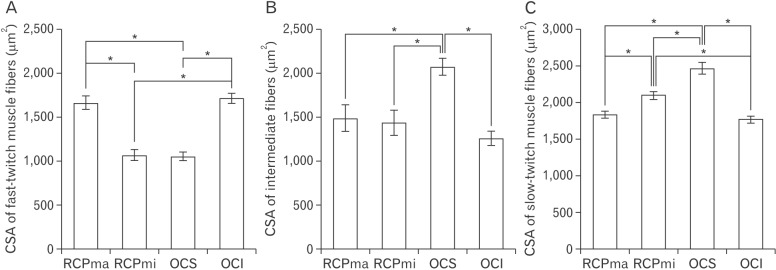
Table 1
Rate of variation in suboccipital muscle groups (n=44)

Type I | Type II | Type III | Type IV | Type V | |
---|---|---|---|---|---|
RCPma | 86.4 | 4.5 | 2.3 | 0 | 6.8 |
RCPmi | 86.4 | 2.3 | 0 | 4.5 | 6.8 |
OCS, OCI | 100 | 0 | 0 | 0 | 0 |
Values are presented as number (%). Type I, muscle fiber bundles exhibiting a typical form of attachment; type II, attached muscle fiber bundles with two muscle bellies; type III, attached muscle fiber bundles with three muscle bellies; type IV, muscle belly is missing; type V, muscle fiber bundles where the muscle belly has fused with another muscle belly; RCPma, rectus capitis posterior major muscle; RCPmi, rectus capitis posterior minor muscle; OCS, obliquus capitis superior; OCI, obliquus capitis inferior.
Table 2
CSA of each muscle fiber (µm2) (n=6)
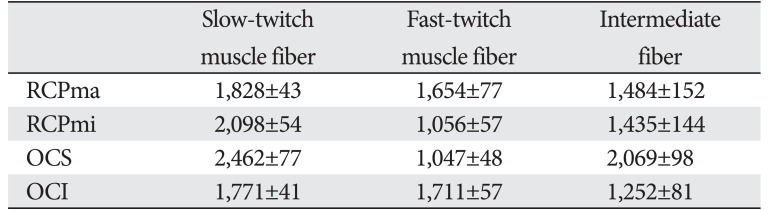
Slow-twitch muscle fiber | Fast-twitch muscle fiber | Intermediate fiber | |
---|---|---|---|
RCPma | 1,828±43 | 1,654±77 | 1,484±152 |
RCPmi | 2,098±54 | 1,056±57 | 1,435±144 |
OCS | 2,462±77 | 1,047±48 | 2,069±98 |
OCI | 1,771±41 | 1,711±57 | 1,252±81 |
Table 3
CSA of each sides muscle fiber (µm2) (n=3)
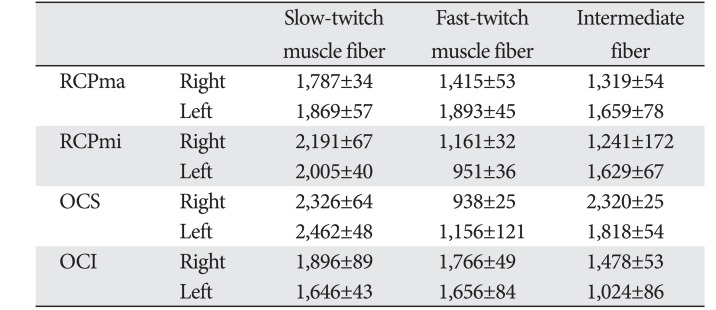