Abstract
Post-menopausal osteoporosis (PMO) is a major global human health concern. Owing to the need for therapeutic drugs without side effects, natural extracts containing various polyphenolic compounds that may exert estrogenic effects have been studied in depth. Rhus verniciflua Stokes (RVS), which has been used as a traditional herbal medicine for centuries in Korea, was recently revealed to exert estrogenic effects attributable to its bioactive ingredients sulfuretin and butein, which have strong estrogen receptor–binding affinities. In this study, the protective potential of RVS in PMO was evaluated by using an experimental animal model of PMO, which was established by ovariectomy (OVX) of female Sprague Dawley rats. The oral administration of RVS at 20 mg/kg or 100 mg/kg for 8 weeks markedly protected against OVX-induced atrophy of the uterine tube and reversed the elevation in the ratio of serum receptor activator of nuclear factor-κB ligand to osteoprotegerin, which is a marker of disease severity. In addition, RVS inhibited OVX-induced tibia bone loss, activated osteogenic activity, and suppressed osteoclastic activity in the tibial epiphyseal plate, a region of bone remodeling. Collectively, these factors indicated that the oral intake of RVS might be beneficial for the prevention of PMO.
Osteoporosis, which could exacerbate after menopause, is a serious problem for elderly women worldwide [1]. It is widely accepted that 17-beta-estradiol (E2) deficiency plays a key role in the pathogenesis of post-menopausal osteoporosis (PMO) [2]. Indeed, estrogen can modulate bone production and bone resorption, exerting distinct effects on osteoblasts and osteoclasts directly via estrogen receptor (ER)–mediated mechanisms [34]. It was recently reported that estrogen inhibited the production of bone resorption-related cytokines such as tissue necrosis factor-α, granulocyte macrophage colony-stimulating factor (M-CSF), and prostaglandin-E2; stimulated osteoblast proliferation; and suppressed osteoclast activity [5]. Based on the anabolic actions of estrogen on bone remodeling, estrogen replacement therapy (ERT) has been used as a therapeutic modality in elderly women for the prevention or treatment of PMO [6]. However, the economic burden and risk of malignant changes in estrogen-sensitive organs, e.g., the breast, uterus, and ovaries, owing to long-term ERT, have strongly restricted its clinical applicability [78]. Furthermore, bone mineral density may decline even more sharply after the withdrawal of short-term ERT, increasing the risk of fracture. Thus, there is an urgent need for the development of alternative therapeutic options for PMO with high cost-effectiveness and no harmful effects.
Interest in the use of plant-derived estrogens, also known as phytoestrogens, has increased significantly. Phytoestrogens are natural polyphenolic compounds with structural similarity to E2 [9]. Although efficacies of phytoestrogens are not as high as those of endogenous estrogens, many patients with PMO self-prescribe these compounds and they have gained worldwide acceptance as beneficial and safe treatments. Based on their functional similarity with E2, most flavonoids, including isoflavones, isoflavanones, flavones, chalcones, and flavanones, are considered to be phytoestrogens [1011].
Many natural plants are known to contain a variety of flavonoids. Rhus verniciflua Stokes (RVS) is an Asian tree species of the genus Toxicodendron, native to China and the Indian subcontinent, and cultivated in regions of China, Korea, and Japan [12]. Commonly known as the lacquer tree, RVS has been utilized as a natural dye, food additive, and herbal medicine [13]. Although Dongui-Bogam (the Korean traditional medicinal encyclopedia) reported that RVS has beneficial health-promoting effects, including prevention of circulatory problems, improvement of blood homeostasis, antibacterial activity, and stimulation of the bone marrow function, the clinical therapeutic use of RVS has been restricted because of the presence of urushiol, an allergenic constituent, which might cause anaphylaxis [1415]. RVS has been reported to exert various biological activities, including anti-inflammatory, anti-allergic, antitumor, neuroprotective, and antithrombotic effects [16171819]. A recent study revealed that butein and sulfuretin, the flavonoid components of RVS, have strong affinities for the ER and consequently exhibit prominent estrogenic activity [20].
Based on the accumulated evidence of the various biological actions of RVS, including the estrogenic activity described above, we hypothesized that the long-term intake of RVS might prevent or delay the progression of PMO. To date, no reports have been published on the possible in vivo effects of RVS in relation to the prevention of PMO. To address this issue, this study evaluated the anti-osteoporotic activity of RVS in an experimental animal model of PMO, which was produced by ovariectomy of female Sprague Dawley (SD) rats. After the intragastric administration of RVS, we investigated the serological changes in markers that reflect the severity of PMO and the histologic changes in the uterine tubes and the epiphyseal plates in the tibia. Furthermore, using microcomputed tomography (µCT), various indices that were indicative of the status of the tibial microarchitecture were analyzed.
The hot water extract of RVS was kindly supplied by Lifetree Biotech Co., Ltd (Suwon, Korea) and prepared as described previously [21]. In brief, the timber of RVS was harvested in Wonju, Gangwon-do, South Korea, cut into pieces of 11×1×0.2 cm, mixed with water (1:10, w/v), and then eluted with boiling water (90℃–110℃) for 4 hours. The extract was concentrated to yield 15% solid content. The concentrated extract was diluted with an equal volume of dextrin and dried by a spray-drying method. Urushiol, an allergenic constituent of RVS, was removed and the urushiol-free RVS was authenticated by Korea Advanced Food Research Institute (KAFRI, Seoul, Korea).
All experiments with rats were acknowledged and regulated by the Institutional Animal Care and Use Committee (IACUC; approved protocol No. P-16-05-A-03) of Konyang University (Daejeon, Korea). Eight-week-old female SD rats (n=48; body weight, 200–250 g) were purchased from Samtako (Osan, Korea). The rats were acclimatized in an environmentally controlled room (temperature, 23±2℃; relative humidity, 60±10%; 12-hour light/dark cycle) and given free access to water and food. All experiments were conducted in accordance with the “Guide for the Care and Use of Laboratory Animals” (National Institutes of Health publication, 8th edition, 2011).
The rats were randomly divided into the following groups (n=12 per group): sham-surgery (Sham); ovariectomized (OVX); ovariectomized and subsequently administered RVS at a low dose (20 mg/kg; RVS-L); and ovariectomized and subsequently administered RVS at a high dose (100 mg/kg; RVS-H). For the OVX, RVS-L, and RVS-H groups, a ketamine/Rompun cocktail (40 mg ketamine and 10 mg Rompun/kg body weight) was intraperitoneally administered for anesthesia and bilateral oophorectomies were conducted. For the RVS-L and RVS-H groups, RVS diluted in distilled water was intragastrically administered daily for 8 weeks from the first day after the operation. The Sham group was subjected to laparotomy, but without ovariectomy. After the RVS administration period, all rats were weighed and sacrificed. The livers and uterine tubes were excised and weighed. Arterial blood was collected from the abdominal aorta for serological assay. After the separation of the surrounding soft tissues, both tibiae were excised from individual rats and immersed in 10% neutral buffered formalin (NBF) for 24 hours.
After the collection of arterial blood, sera were obtained by centrifugation. The sera were stored at −70℃ until assayed. The levels of serum glutamic-oxaloacetic transaminase (GOT), glutamic pyruvic transaminase (GPT), and total cholesterol were measured with a DRI-CHEM 3000 colorimetric analyzer (Fujifilm, Tokyo, Japan). The serum levels of osteoprotegerin (OPG) and receptor activator of nuclear factor-κB ligand (RANKL) were determined by enzyme-linked immunosorbent assay assay kits (R&D Systems, Minneapolis, MN, USA) using a microplate reader (BioTek Instruments, Winooski, VT, USA) in accordance with the manufacturer's instructions.
The µCT determination of the excised tibia was performed with a Sky Scan 1076 CT scanner (Aartselaar, Belgium), as previously described [22]. Tibiae fixed with 10% NBF were scanned in batches of three at a nominal resolution (pixels) of 18 µm. The reconstruction was generated by using a modified Feldkamp algorithm using the Skyscan NRecon software. The X-ray source settings were as follows: 70 kV, 100 mA, and a pixel size of 18 µm. A hundred projections were acquired over an angular range of 180°. The image slices were reconstructed using cone-beam reconstruction software (version 2.6) in accordance with the Feldkamp algorithm. The following parameters were analyzed: bone volume per tissue volume (BV/TV), trabecular thickness (Tb.Th), number (Tb.N), and separation (Tb.Sp), and structural indices including trabecular pattern factor (Tb.Pf) and structure model index (SMI).
The uterine tubes and tibiae were dehydrated with an ethanol gradient series, embedded in paraffin, and sliced into 5 µm sections using a microtome (RM2255, Leica, Nussloch, Germany). Prior to the tissue processing, the tibiae were decalcified by soaking in 5 ml 10% EDTA at room temperature for 3 weeks; the EDTA solution was changed every 3 days. Decalcification was confirmed by the absence of resistance when the bones were punctured with a pin. Hematoxylin and eosin (H&E) staining of the uterine tubes and tibiae was performed as described in previous studies [2324]. In particular, for preparation of the high-magnification image of tibiae epiphyseal plate, stain with only hematoxylin was used for better visibility. For immunohistochemistry (IHC), the tibiae sections were deparaffinized in xylene, hydrated by immersion in a decreasing ethanol gradient series, and washed in distilled water. Next, each section was incubated with primary antibodies that were diluted in blocking solution at a ratio of 1:200 for rabbit anti-tartrate-resistant acid phosphatase (TRAP; Abcam, Cambridge, MA, USA) for 24 hours at 4℃. After three washes in phosphate-buffered saline, the sections incubated with anti-TRAP were incubated with secondary antibodies containing biotinylated anti-rabbit IgG (Vector Laboratories, Inc., Burlingame, CA, USA) for 1 hour at room temperature. The slides were further incubated with an avidin-biotin complex solution (Vector Laboratories, Inc.) for 1 hour at room temperature. The immunopositive regions turned dark brown after the addition of the chromogen 3,3′-diaminobenzidine tetrahydrochloride (Sigma-Aldrich, St. Louis, MO, USA). Two microscopic fields of tissue sections were randomly selected from each rat (n=12 in each group). These fields were photographed at 200× magnification by a digital camera connected to a light microscope (DM4, Leica) and examined by three blind observers.
All data were presented as the mean±standard error of the mean (SEM). Comparisons of the data from the different groups were performed with one-way analysis of variance (ANOVA, PASW Statistics version 18, SPSS Inc., Chicago, IL, USA). The differences with values of P<0.05 were considered to be statistically significant. Each “n” value refers to the number of separate experiments conducted.
Before the evaluation of the preventive effect of RVS in the rat model of PMO, general conditions and liver functions were assessed in terms of body weight, liver weight, and serological parameters, such as serum GOT and GPT levels (Table 1). Consistent with previous studies [2526], the OVX group exhibited significant weight gain compared with the Sham group. Although there were clear trends of weight reduction in the RVS-L and RVS-H groups compared with that in the OVX group, the differences were not statistically significant. Liver weight, as well as serum GOT and GPT levels, were not significantly increased by ovariectomy or RVS treatment, which suggested that both OVX and RVS did not induce hepatotoxicities such as hepatomegaly. Conversely, total cholesterol, a reliable marker of hyperlipidemia that is commonly associated with postmenopausal syndrome, was significantly elevated in the OVX group; however, treatment with RVS at 20 mg/kg and 100 mg/kg significantly reversed this elevation. Together, these results revealed that the 8-week oral administration of RVS exerted a beneficial effect against hyperlipidemia without causing significant hepatotoxicity.
The in vivo estrogenic activity of RVS was evaluated by the measurement of the extent of uterine tube atrophies in ovariectomized rats with or without RVS treatment. Representative macroscopic and microscopic images of the uterine tubes of rats in each group are shown in Fig. 1A. The average wet weight and the wall thickness of the uterine tubes of each group are shown in Fig. 1B and C, respectively. As shown in Fig. 1A, compared with the Sham group, the OVX group exhibited macroscopic narrowing of the uterine tubes, which were markedly normalized in the RVS-L and RVS-H groups. As demonstrated in Fig. 1B and C, the RVS-L and RVS-H groups showed a significant reversal of the uterine tube atrophies observed in the OVX group (0.36±0.14 g and 0.57±0.02 g vs. 0.09±0.01 g, respectively, for wet weight; 0.59±0.13 mm and 0.77±0.16 mm vs. 0.31±0.02 mm, respectively, for wall thickness). In particular, the extent of normalization in wet weight and wall thickness of uterine tubes was more prominent in the RVS-H groups than in the RVS-L group, which suggested that the observed changes were dose-dependent.
The protective effects of RVS on bone microstructure in the rat model of PMO were evaluated by µCT analysis of the excised tibia proximal ends. Representative µCT images of tibial metaphysis confirmed the expected trabecular bone loss in the OVX group compared with the Sham group, whereas marked preservation of the trabecular bone was observed in the RVS-L and RVS-H groups (Fig. 2A). The quantitative analysis of the trabecular responses (Fig. 2B–G) showed that, compared with that in the Sham group, BV/TV, Tb.Th, and Tb.N decreased and Tb.Sp, Tb.Pf, and SMI increased in the OVX group, as expected. RVS treatment of ovariectomized rats dose-dependently increased BV/TV, Tb.Th, and Tb.N relative to that in the OVX group, although all parameters were still lower than in the Sham group. In contrast, Tb.Sp, Tb.Pf, and SMI decreased in a dose-dependent manner in the RVS group in comparison with that in the OVX group. In conjunction with the µCT results on proximal ends of the tibiae, we concluded that RVS exerts a bone-preserving effect against PMO-induced bone loss.
As the biology of osteoblasts and osteoclasts is largely regulated by signaling mediators i.e., RANKL and its inhibitor OPG [2728], the serum levels of OPG and RANKL, as well as their relative ratio, were compared at 8 weeks after PMO induction in rats. As shown in Fig. 3A and B, the serum OPG level was not significantly changed in any group, whereas serum RANKL level was elevated in the OVX and RVS-L groups compared with that of the Sham group (0.58±0.07 and 0.50±0.04 vs. 0.33±0.08 ng/ml, respectively). Conversely, serum RANKL level decreased in the RVS-H group. However, the ratio of serum RANKL to OPG decreased in both the RVS-L and RVS-H groups in comparison with that of the OVX group (37.56±4.87% and 29.67±10.41% vs. 51.38±9.76%, respectively) (Fig. 3C). The decrease in serum RANKL to OPG ratio was dose-dependent. Collectively, these results suggested that the protective effect of RVS on bone microstructures was mediated, at least in part, by the suppression of osteoclastic activity.
To gain a deeper insight into the underlying bone-preservation effect of RVS, we assessed the possible effects of RVS on osteogenic and osteoclastic activity in the tibial epiphyseal plate, which is actively regenerated via bone remodeling. The H&E-stained sagittal sections (×50) (Fig. 4A) of the proximal tibia showed that the bony trabecular meshwork was apparently reduced and frequently interrupted (as indicated with yellow arrowheads) in the diaphysis in the OVX, RVS-L, and RVS-H groups in comparison with that in the Sham group. As shown in Fig. 4B (×200, representative images depicted with magnified regions of corresponding rectangular boxes in Fig. 4A) and Fig. 4C, the quantitative graph, the animals in the OVX group showed a widened resting zone (RZ) and reciprocally shortened proliferative zone (PZ) in the tibial epiphyseal plate. In contrast, a significant reversal of the effects on the above parameters was observed in the RVS-L and RVS-H groups in comparison with the OVX group (68±8.36 µm and 54±11.40 µm vs. 98±19.23 µm, respectively, for RZ, 40±7.07 µm and 48±8.36 µm vs. 34±8.94 µm, respectively, for PZ). Additionally, the possible effect of RVS on osteoclastic activity was evaluated by IHC using antibody against TRAP, a reliable histologic marker of multi-nucleated osteoclasts. As shown in Fig. 5A (the representative images) and Fig. 5B (the quantitative graph), the TRAP IHC staining (brown stain) showed a robust increase in osteoclast activities (as indicated with white arrowheads), especially in the junction between the diaphysis and the epiphyseal plate in proximal tibia of animals in the OVX group in comparison with that in the Sham group. However, osteoclast activity in the RVS-H group, but not in the RVS-L group, was markedly diminished compared with that of the OVX group (1.81±0.60 vs. 3.16±0.07). Collectively, these findings demonstrated that RVS consumption could reverse or delay the progression of PMO, at least partially, by the simultaneous promotion of osteogenesis and the suppression of osteoclast-mediated bone resorption in the epiphyseal plate.
This is the first study to investigate the possible preventive or delaying effects of RVS on PMO progression using various in vivo experiments in a rat model of PMO established by ovariectomy. This study revealed that orally administered RVS (20 or 100 mg/kg for 8 weeks) markedly attenuated the atrophy of the uterine tube, elevation of serum markers of osteoclastic activity (RANKL to OPG ratio), bone microstructural deterioration of the tibia, and imbalance between osteogenic and osteoclastic activity in the tibial epiphyseal plate of the PMO model. Collectively, these results suggested the potential role of RVS as a natural alternative for PMO management.
In addition to the discovery of anti-osteoporotic effects, this study also revealed a serum cholesterol-lowering effect of RVS. In agreement with previous studies, ovariectomy significantly increased serum total cholesterol [293031]. Although the exact mechanisms of ovariectomy-induced hypercholesterolemia have not been clearly elucidated, the most plausible explanation for this phenomenon is the disturbance of cholesterol clearance [32]. Indeed, the ovaries in rodent models are known to be second to the liver with regard to capacity for cholesterol clearance [33]. Post-menopause in elderly women, the incidence of atherosclerosis in coronary arteries surges dramatically owing to E2 deficiency [34]. Increased serum cholesterol level has been implicated as a major predisposition factor for atherosclerosis [35]. Other pharmacologic medications, e.g., statins, which are used to lower cholesterol are often associated with contraindications or side effects, which prevents their use in individuals who are taking other forms of medication. Thus, if possible, alternatives to pharmacologic medication for the reduction of cholesterol level, as well as the risk of atherosclerosis, are highly desirable. The serum cholesterol-lowering effect of RVS demonstrated in this study suggests that RVS may be a safe and efficient alternative to synthetic drugs for the prevention of postmenopausal atherosclerosis.
OPG is an endogenous protein of the tumor necrosis factor receptor superfamily; released by osteoblasts, it inhibits osteoclast activation by acting as a decoy receptor for RANKL [36]. Osteoclasts are multinucleated giant cells derived from hematopoietic progenitors of the monocyte/macrophage lineage through a differentiation process mainly governed by two key cytokines: M-CSF and RANKL [37]. M-CSF induces the proliferation and stimulation of the expression of RANK, the receptor of RANKL, in the precursor cells [38]. The binding of RANK to RANKL leads to the subsequent activation of downstream signaling pathways such as nuclear factor κB (NF-κB) [39]. NF-κB then induces the expression of the transcription factor nuclear factor of activated T cells 1 (NFATc1), a master regulator of osteoclast differentiation [40]. NFATc1 regulates the transcription of several target genes, including TRAP, which participate in the osteoclast phenotype and bone matrix degradation [41]. Thus, the osteoclastic activity might depend predominantly on the expression or activity of RANKL and its inhibitor, OPG, in the bone microenvironment [4243]. The present study showed that RVS decreased serum RANKL, but not the OPG level. Therefore, we can suggest that RVS acts primarily on osteoclasts, but does not directly stimulate osteoblasts to produce OPG, although a detailed study of the mechanism of RVS-mediated RANKL is required.
Phytoestrogens, including RVS, have been known to have multiple modes of action, which indicates that there may be other mechanisms responsible for its anti-osteoporotic effects in addition to the E2-mimetic property demonstrated in the present study; previous studies have demonstrated that various polyphenolic constituents of RVS are potent scavengers of reactive oxygen species (ROS) [4445]. Indeed, although estrogen deficiency has been considered the central and primary cause of osteoporosis in post-menopausal women, a variety of recent studies have indicated that aging and the associated increase in ROS are the main culprits [46]. In addition to original research, one review paper (written in 2010) elegantly provided the paradigm shift from the “estrogen-centric” account of involutional osteoporosis pathogenesis to one in which ROS-related mechanisms intrinsic to bone are the key events [47]. Therefore, although the present study revealed the E2-mimetic property of RVS was at least partly responsible for the protective effects of PMO, as demonstrated by the attenuation of uterine tube atrophy, further investigations will be required to elucidate the exact mechanism from the multiple modes of actions, including the role as a ROS scavenger.
Figures and Tables
Fig. 1
Protective effect of Rhus verniciflua Stokes (RVS) on ovariectomy-induced atrophy of the uterine tubes. Rats (n=12 in each group) were intragastrically treated with or without RVS (20 mg/kg for RVS at a low dose [RVS-L] and 100 mg/kg for RVS at a high dose [RVS-H] group) for 8 weeks after ovariectomy. The sham-surgery (Sham) group was subjected to laparotomy, but not ovariectomy. The ovariectomy (OVX) group was administered distilled water as a vehicle. The photomicrographs are representative gross morphologies (A, upper panel) and H&E-stained transverse sections (A, lower panel) of the excised uterine tubes of each group. The average wet weight (B) and thickness (C) of the uterine tubes of each group were quantitatively assessed. The data in all graphs are expressed as the mean±SEM (*P<0.05 and ***P<0.001 vs. Sham, #P<0.05, ##P<0.01, and ###P<0.001 vs. OVX).
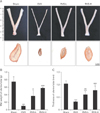
Fig. 2
Protective effects of Rhus verniciflua Stokes (RVS) on ovariectomy-induced deterioration of rat tibial microstructure. Rats (n=12 in each group) were intragastrically treated with or without RVS (20 mg/kg for RVS at a low dose [RVS-L] and 100 mg/kg for RVS at a high dose [RVS-H]) for 8 weeks after ovariectomy. The sham-surgery (Sham) group was subjected to laparotomy, but not ovariectomy. The ovariectomy (OVX) group was administered distilled water as a vehicle. After treatment of RVS for 8 weeks, both tibiae of each rat were excised. After the decalcification step, the bone microstructure of proximal tibia was analyzed by microcomputed tomography (µCT). Representative µCT images are shown in panel (A) and the parameters such as bone volume per tissue volume (BV/TV) (B), trabecular thickness (Tb.Th) (C), trabecular number (Tb.N) (D), trabecular separation (Tb.Sp) (E), trabecular pattern factor (Tb.Pf) (F), and structure model index (SMI) (G) were analyzed. The data in all graphs are expressed as the mean±SEM (*P<0.05, **P<0.01, and ***P<0.001 vs. Sham; #P<0.05, ##P<0.01, and ###P<0.001 vs. OVX).
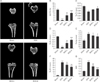
Fig. 3
Suppressive effect of Rhus verniciflua Stokes (RVS) on ovariectomy-induced elevation of the serum receptor activator of nuclear factor-κB ligand (RANKL)/osteoprotegerin (OPG) ratio. Rats (n=12 in each group) were intragastrically treated with or without RVS (20 mg/kg for RVS at a low dose [RVS-L] and 100 mg/kg for RVS at a high dose [RVS-H] group) for 8 weeks after ovariectomy. The sham-surgery (Sham) group was subjected to laparotomy, but not ovariectomy. The ovariectomy (OVX) group was administered distilled water as a vehicle. After RVS treatment for 8 weeks, the serum levels of OPG (A) and RANKL (B) were determined by using enzyme-linked immunosorbent assay kits and the ratio of RANKL to OPG (C) was calculated. The data in all graphs are presented as the mean±SEM (*P<0.05 and **P<0.01 vs. Sham, #P<0.05 vs. OVX).
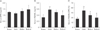
Fig. 4
Preserved or enhanced activity of bone remodeling in tibial epiphyseal plate by Rhus verniciflua Stokes (RVS) treatment in ovariectomy-induced osteoporotic rats. Rats (n=12 in each group) were intragastrically treated with or without RVS (20 mg/kg for RVS at a low dose [RVS-L] and 100 mg/kg for RVS at a high dose [RVS-H] group) for 8 weeks after ovariectomy. The sham-surgery (Sham) group was subjected to laparotomy, but not ovariectomy. The ovariectomy (OVX) group was administered distilled water as a vehicle. After RVS treatment for 8 weeks, both tibiae of each rat were excised. After a decalcification step, the histological morphology in the epiphyseal plate of tibia was observed after being stained with H&E (A, ×100, a representative image) or hematoxylin (B, ×400, the magnified regions of corresponding yellow rectangular boxes in A). Yellow arrowheads indicate the discontinued trabecular meshwork. The average length of resting zone (RZ) and proliferation zone (PZ) in the tibial epiphyseal plate of each group is quantitatively expressed in panel (C). The data in all graphs are expressed as the mean±SEM (*P<0.05 and **P<0.01 vs. Sham, #P<0.05 and ##P<0.01 vs. OVX).
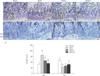
Fig. 5
Inhibition of osteoclastic activity in the tibial epiphyseal plate by Rhus verniciflua Stokes (RVS) treatment in ovariectomy-induced osteoporotic rats. Rats (n=12 in each group) were intragastrically treated with or without RVS (20 mg/kg for RVS at a low dose [RVS-L] and 100 mg/kg for RVS at a high dose [RVS-H] group) for 8 weeks after ovariectomy. The sham-surgery (Sham) group was subjected to laparotomy, but not ovariectomy. The ovariectomy (OVX) group was administered distilled water as a vehicle. After treatment of RVS for 8 weeks, both tibiae of each rat were excised. As shown in the representative images (A, ×100) and the quantitative graph (B), after the decalcification step, the activity of osteoclasts in tibia epiphyseal plate of each group was quantitatively analyzed by the analysis of the optical density of the brown-colored immunoreaction (indicated by white arrowheads) after immunohistochemical staining with tartrate-resistant acid phosphatase (TRAP). The data are expressed as the mean±SEM (*P<0.05 and ***P<0.001 vs. Sham, ##P<0.01 vs. OVX).
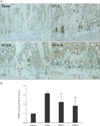
Table 1
Effect of RVS on ovariectomy-induced alterations in parameters related to obesity, hepatotoxicity, and hyperlipidemia

Values are presented as mean±SD. RVS, Rhus verniciflua Stokes; Sham, sham-surgery; OVX, ovariectomy; RVS-L, RVS at a low dose; RVS-H, RVS at a high dose; BW, body weight; GOT, glutamic-oxaloacetic transaminase; GPT, glutamic-pyruvic transaminase. a)P<0.05 compared with the Sham group. b)P<0.05 compared with the OVX group.
Acknowledgements
The work was supported by funds from Korea Research Foundation (grant no. NRF-2016R1C1B2012351, -2017R1C1B2006365, -2017R1D1A04034160 and 2017R1D1A3B04034812).
References
1. Bernabei R, Martone AM, Ortolani E, Landi F, Marzetti E. Screening, diagnosis and treatment of osteoporosis: a brief review. Clin Cases Miner Bone Metab. 2014; 11:201–207.
2. Greenwald MW, Gluck OS, Lang E, Rakov V. Oral hormone therapy with 17beta-estradiol and 17beta-estradiol in combination with norethindrone acetate in the prevention of bone loss in early postmenopausal women: dose-dependent effects. Menopause. 2005; 12:741–748.
3. Imai Y, Kondoh S, Kouzmenko A, Kato S. Minireview: osteoprotective action of estrogens is mediated by osteoclastic estrogen receptor-alpha. Mol Endocrinol. 2010; 24:877–885.
4. Galea GL, Meakin LB, Sugiyama T, Zebda N, Sunters A, Taipaleenmaki H, Stein GS, van Wijnen AJ, Lanyon LE, Price JS. Estrogen receptor alpha mediates proliferation of osteoblastic cells stimulated by estrogen and mechanical strain, but their acute down-regulation of the Wnt antagonist Sost is mediated by estrogen receptor beta. J Biol Chem. 2013; 288:9035–9048.
5. Khosla S, Oursler MJ, Monroe DG. Estrogen and the skeleton. Trends Endocrinol Metab. 2012; 23:576–581.
6. Na KS, Jee DH, Han K, Park YG, Kim MS, Kim EC. The ocular benefits of estrogen replacement therapy: a population-based study in postmenopausal Korean women. PLoS One. 2014; 9:e106473.
7. Fernandez E, Gallus S, Bosetti C, Franceschi S, Negri E, La Vecchia C. Hormone replacement therapy and cancer risk: a systematic analysis from a network of case-control studies. Int J Cancer. 2003; 105:408–412.
8. Humphries KH, Gill S. Risks and benefits of hormone replacement therapy: the evidence speaks. CMAJ. 2003; 168:1001–1010.
9. Gencel VB, Benjamin MM, Bahou SN, Khalil RA. Vascular effects of phytoestrogens and alternative menopausal hormone therapy in cardiovascular disease. Mini Rev Med Chem. 2012; 12:149–174.
10. Miksicek RJ. Commonly occurring plant flavonoids have estrogenic activity. Mol Pharmacol. 1993; 44:37–43.
11. Sulaiman CT, Arun A, Anandan EM, Sandhya CR, Balachandran I. Isolation and identification of phytoestrogens and flavonoids in an Ayurvedic proprietary medicine using chromatographic and mass spectroscopic analysis. Asian Pac J Reprod. 2015; 4:153–156.
12. Kitts DD, Lim KT. Antitumorigenic and cytotoxic properties of an ethanol extract derived from Rhus verniciflua Stokes (RVS). J Toxicol Environ Health A. 2001; 64:357–371.
13. Hong DH, Han SB, Lee CW, Park SH, Jeon YJ, Kim MJ, Kwak SS, Kim HM. Cytotoxicity of urushiols isolated from sap of Korean lacquer tree (Rhus vernicifera Stokes). Arch Pharm Res. 1999; 22:638–641.
14. Park JM, Lee JH, Na CS, Lee D, Lee JY, Satoh M, Lee MY. Heartwood extract of Rhus verniciflua Stokes and its active constituent fisetin attenuate vasoconstriction through calcium-dependent mechanism in rat aorta. Biosci Biotechnol Biochem. 2016; 80:493–500.
15. Park SD, Lee SW, Chun JH, Cha SH. Clinical features of 31 patients with systemic contact dermatitis due to the ingestion of Rhus (lacquer). Br J Dermatol. 2000; 142:937–942.
16. Shin JS, Park YM, Choi JH, Park HJ, Shin MC, Lee YS, Lee KT. Sulfuretin isolated from heartwood of Rhus verniciflua inhibits LPS-induced inducible nitric oxide synthase, cyclooxygenase-2, and pro-inflammatory cytokines expression via the down-regulation of NF-kappaB in RAW 264.7 murine macrophage cells. Int Immunopharmacol. 2010; 10:943–950.
17. Lee JD, Huh JE, Jeon G, Yang HR, Woo HS, Choi DY, Park DS. Flavonol-rich RVHxR from Rhus verniciflua Stokes and its major compound fisetin inhibits inflammation-related cytokines and angiogenic factor in rheumatoid arthritic fibroblast-like synovial cells and in vivo models. Int Immunopharmacol. 2009; 9:268–276.
18. Kim JH, Jung CH, Jang BH, Go HY, Park JH, Choi YK, Hong SI, Shin YC, Ko SG. Selective cytotoxic effects on human cancer cell lines of phenolic-rich ethyl-acetate fraction from Rhus verniciflua Stokes. Am J Chin Med. 2009; 37:609–620.
19. Lim KT, Hu C, Kitts DD. Antioxidant activity of a Rhus verniciflua Stokes ethanol extract. Food Chem Toxicol. 2001; 39:229–237.
20. Sun S, Choi YH, Na CS, Lee D, Yoo HH, Hong CY, Ahn BY, Dong MS. Estrogenic activity of a Rhus verniciflua extract and its major components. J Funct Foods. 2014; 11:250–260.
21. Gil MN, Choi DR, Yu KS, Jeong JH, Bak DH, Kim DK, Lee NS, Lee JH, Jeong YG, Na CS, Na DS, Ryu KH, Han SY. Rhus verniciflua Stokes attenuates cholestatic liver cirrhosis-induced interstitial fibrosis via Smad3 down-regulation and Smad7 up-regulation. Anat Cell Biol. 2016; 49:189–198.
22. Genant HK, Engelke K, Prevrhal S. Advanced CT bone imaging in osteoporosis. Rheumatology (Oxford). 2008; 47:Suppl 4. iv9–iv16.
23. Du J, Feng W, Sun J, Kang C, Amizuka N, Li M. Ovariectomy upregulated the expression of peroxiredoxin 1 &5 in osteoblasts of mice. Sci Rep. 2016; 6:35995.
24. Kim W, Bae S, Kim H, Kim Y, Choi J, Lim SY, Lee HJ, Lee J, Jang M, Lee KE, Chung SG, Hwang YI, Kang JS, Lee WJ. Ascorbic acid insufficiency induces the severe defect on bone formation via the down-regulation of osteocalcin production. Anat Cell Biol. 2013; 46:254–261.
25. Xie F, Wu CF, Lai WP, Yang XJ, Cheung PY, Yao XS, Leung PC, Wong MS. The osteoprotective effect of Herba epimedii (HEP) extract in vivo and in vitro. Evid Based Complement Alternat Med. 2005; 2:353–361.
26. Gjoksi B, Ghayor C, Siegenthaler B, Ruangsawasdi N, Zenobi-Wong M, Weber FE. The epigenetically active small chemical N-methyl pyrrolidone (NMP) prevents estrogen depletion induced osteoporosis. Bone. 2015; 78:114–121.
27. Walsh MC, Choi Y. Biology of the RANKL-RANK-OPG system in immunity, bone, and beyond. Front Immunol. 2014; 5:511.
28. Babaji P, Devanna R, Jagtap K, Chaurasia VR, Jerry JJ, Choudhury BK, Duhan D. The cell biology and role of resorptive cells in diseases: a review. Ann Afr Med. 2017; 16:39–45.
29. van Lenten BJ, Melchior GW, Roheim PS. Lipoprotein metabolism in the ovariectomized rat. J Lipid Res. 1983; 24:1475–1484.
30. Kikuchi-Hayakawa H, Onodera N, Matsubara S, Yasuda E, Chonan O, Takahashi R, Ishikawa F. Effects of soy milk and bifidobacterium fermented soy milk on lipid metabolism in aged ovariectomized rats. Biosci Biotechnol Biochem. 1998; 62:1688–1692.
31. Liu D, Bachmann KA. An investigation of the relationship between estrogen, estrogen metabolites and blood cholesterol levels in ovariectomized rats. J Pharmacol Exp Ther. 1998; 286:561–568.
32. Lucas EA, Juma S, Stoecker BJ, Arjmandi BH. Prune suppresses ovariectomy-induced hypercholesterolemia in rats. J Nutr Biochem. 2000; 11:255–259.
33. Spady DK, Bilheimer DW, Dietschy JM. Rates of receptor-dependent and -independent low density lipoprotein uptake in the hamster. Proc Natl Acad Sci U S A. 1983; 80:3499–3503.
34. Sznajderman M, Oliver MF. Spontaneous premature menopause, ischemic heart-disease, and serum-lipids. Lancet. 1963; 281:962–965.
35. Rosenberg L, Hennekens CH, Rosner B, Belanger C, Rothman KJ, Speizer FE. Early menopause and the risk of myocardial infarction. Am J Obstet Gynecol. 1981; 139:47–51.
36. Yasuda H, Shima N, Nakagawa N, Yamaguchi K, Kinosaki M, Mochizuki S, Tomoyasu A, Yano K, Goto M, Murakami A, Tsuda E, Morinaga T, Higashio K, Udagawa N, Takahashi N, Suda T. Osteoclast differentiation factor is a ligand for osteoprotegerin/osteoclastogenesis-inhibitory factor and is identical to TRANCE/RANKL. Proc Natl Acad Sci U S A. 1998; 95:3597–3602.
37. Boyle WJ, Simonet WS, Lacey DL. Osteoclast differentiation and activation. Nature. 2003; 423:337–342.
38. Arai F, Miyamoto T, Ohneda O, Inada T, Sudo T, Brasel K, Miyata T, Anderson DM, Suda T. Commitment and differentiation of osteoclast precursor cells by the sequential expression of c-Fms and receptor activator of nuclear factor kappaB (RANK) receptors. J Exp Med. 1999; 190:1741–1754.
39. Lee SE, Woo KM, Kim SY, Kim HM, Kwack K, Lee ZH, Kim HH. The phosphatidylinositol 3-kinase, p38, and extracellular signal-regulated kinase pathways are involved in osteoclast differentiation. Bone. 2002; 30:71–77.
40. Takatsuna H, Asagiri M, Kubota T, Oka K, Osada T, Sugiyama C, Saito H, Aoki K, Ohya K, Takayanagi H, Umezawa K. Inhibition of RANKL-induced osteoclastogenesis by (-)-DHMEQ, a novel NF-kappaB inhibitor, through downregulation of NFATc1. J Bone Miner Res. 2005; 20:653–662.
41. Takayanagi H. The role of NFAT in osteoclast formation. Ann N Y Acad Sci. 2007; 1116:227–237.
42. Hofbauer LC, Dunstan CR, Spelsberg TC, Riggs BL, Khosla S. Osteoprotegerin production by human osteoblast lineage cells is stimulated by vitamin D, bone morphogenetic protein-2, and cytokines. Biochem Biophys Res Commun. 1998; 250:776–781.
43. Hofbauer LC, Lacey DL, Dunstan CR, Spelsberg TC, Riggs BL, Khosla S. Interleukin-1beta and tumor necrosis factor-alpha, but not interleukin-6, stimulate osteoprotegerin ligand gene expression in human osteoblastic cells. Bone. 1999; 25:255–259.
44. Jung CH, Jun CY, Lee S, Park CH, Cho K, Ko SG. Rhus verniciflua Stokes extract: radical scavenging activities and protective effects on H2O2-induced cytotoxicity in macrophage RAW 264.7 cell lines. Biol Pharm Bull. 2006; 29:1603–1607.
45. Park BC, Lee YS, Park HJ, Kwak MK, Yoo BK, Kim JY, Kim JA. Protective effects of fustin, a flavonoid from Rhus verniciflua Stokes, on 6-hydroxydopamine-induced neuronal cell death. Exp Mol Med. 2007; 39:316–326.
46. Liochev SI. Reactive oxygen species and the free radical theory of aging. Free Radic Biol Med. 2013; 60:1–4.
47. Manolagas SC. From estrogen-centric to aging and oxidative stress: a revised perspective of the pathogenesis of osteoporosis. Endocr Rev. 2010; 31:266–300.