Abstract
Kidney ischemia and reperfusion injury (IRI) is associated with a high mortality rate, which is attributed to tubular oxidative and nitrative stresses; however, an effective approach to limit IRI remains elusive. Spermidine, a naturally occurring polyamine, protects yeast cells against aging through the inhibition of oxidative stress and necrosis. In the present study, spermidine supplementation markedly attenuated histological damage and kidney dysfunction during IRI. In addition, exogenous spermidine potently inhibited poly(ADP-ribose) polymerase 1 (PARP1) activation and DNA nitrative/oxidative stress following IRI. Conversely, inhibition of ornithine decarboxylase (ODC) via siRNA transfection in vivo significantly enhanced DNA nitration, PARP1 activation, and functional damage during IRI. Finally, in ODC knockdown kidneys, PARP1 inhibition attenuated histological and functional damage induced by IRI, but not DNA nitrative stress. In conclusion, these data suggest that spermidine protects kidneys against IRI through blocking DNA nitration and PARP1 activation and this finding provides a novel target for prevention of acute kidney injury including IRI.
Kidney ischemia and reperfusion injury (IRI), generally regarded as the major cause of acute kidney injury (AKI), results from compromised perfusion of kidney tissues. Histologically, IRI is characterized by cell death in the outer medullary parenchyma, which is proportional to the severity of kidney ischemia [12]. In IRI-subjected kidneys, the majority of tubular damage is caused by necrotic cell death [34]. Oxidative/nitrative stress, poly(ADP-ribose) polymerase 1 (PARP1) upregulation, mitochondrial permeability transition and ferroptosis cause necrotic cellular demise [567], but the mechanism involved in regulating necrotic tubular cell death in the pathogenesis of IRI remains unclear.
Spermidine as a polyamine is present in various foods such as green leafy vegetables, milk, and meat products. Furthermore, spermidine is essential for nearly every cellular function including DNA replication, transcription, and translation [89]. Treatment with spermidine extends life span and health span through the induction of autophagy, and intriguingly, it protects yeast cells against aging through the inhibition of necrosis [10]. Additionally, a spermidine-related system exerts the strongest biological activity including an antioxidant effect in several organs after ischemic injury [111213]. However, little is known about the anti-necrotic mechanism of action of spermidine and the effect of exogenous spermidine on IRI.
Therefore, it has been sought to determine whether exogenous spermidine decreases tubular damage during IRI, and if so, whether genetic inhibition of ornithine decarboxylase (ODC), which synthesizes spermidine, exacerbates IRI. Finally, it has been assessed whether the protective effect of spermidine is implicated in the inhibition of DNA oxidation/nitration and PARP1 activation.
Male C57BL/6 mice aged 8 to 10 weeks were purchased from Orient Bio (Seongnam, Korea). All mouse experiments were performed in accordance with the animal protocols approved by the Institutional Animal Care and Use Committee of Jeju National University. Mice were subjected to 30 minutes of bilateral kidney ischemia followed by 6 hours or 24 hours of reperfusion or sham operation under anesthesia through an intraperitoneal injection of a cocktail containing ketamine (200 mg/kg body weight) and xylazine (16 mg/kg body weight), as previously described [14151617]. Sham-operated mice underwent the same surgical procedure except for the induction of ischemia. Spermidine (10 mg/kg body weight; Sigma, St. Louis, MO, USA) was dissolved in distilled water (vehicle) and administered orally at 24 hours and 1 hour before ischemia or sham operation. PARP1 inhibitor PJ34 (10 mg/kg body weight, R&D Systems, Minneapolis, MN, USA) or saline (vehicle) was administered intraperitoneally at 24 hours and 1 hour before ischemia or sham operation [1819]. To use a small interfering RNA (siRNA) targeting ODC in vivo, ODC siSTABLE siRNA (50 µg in 1 ml phosphate buffered saline [PBS]) modified from siGENOME (Dharmacon, Lafayette, CO, USA) or siSTABLE non-targeting siRNA (control siRNA; 50 µg in 1 ml PBS) was injected within 10 seconds into the tail vein at 48 hours and 24 hours before ischemia, as previously described [1620].
Histological damage due to tubular injury in the outer medulla of periodic acid-Schiff (PAS)-stained kidney sections was scored by counting the percentage of tubules that displayed tubular necrosis, cast formation, and tubular dilation as follows: 0, normal; 1, 1% to 10%; 2, 11% to 25%; 3, 26% to 50%; 4, 51% to 75%; and 5, 76% to 100%. Five randomly chosen high-power (×200 magnification) fields per kidney were used for the scoring [141516181921].
PARP1 activity, 8-nitroguanine, and 8-hydroxy-2′-deoxyguanosine assays were performed in whole kidneys using a universal PARP colorimetric assay kit (Trevigen, Gaithersburg, MD, USA), a 8-nitroguanine DNA/RNA damage ELISA kit (Cell Biolabs, San Diego, CA, USA) and a DNA/RNA oxidative damage ELISA kit (Cayman, Ann Arbor, MI, USA), respectively, according to the manufacturer's instructions [181922232425].
Analysis of variance was used to compare data among groups using Systat SigmaPlot (Systat Software Inc., San Jose, CA, USA). Differences between two groups were assessed by two-tailed unpaired Student's t tests. P-values <0.05 were considered statistically significant. Each experimental group consisted of nine animals (n=9).
Here, it has been confirmed that IRI time-dependently and markedly increased tubular damage scored in PAS-stained kidney sections and kidney dysfunction represented by high concentrations of plasma creatinine at 6 hours and 24 hours after reperfusion in vehicle-treated mice (Fig. 1A–C). Although it has been reported that loss of spermine/spermidine-N(1)-acetyltransferase, an enzyme in spermidine degradation protects against IRI [26], a direct effect of spermidine supplement on IRI has not yet been investigated. In the present study, exogenous spermidine significantly reduced tubular damage during IRI (Fig. 1A, B). Consistent with the histological data, the increases in plasma creatinine concentrations were also significantly lessened by exogenous spermidine (Fig. 1C). These data indicate that exogenous spermidine attenuates histological and functional damage induced by IRI.
As excessive activation of PARP1, which catalyzes poly (ADP-ribosyl)ation of various proteins as well as PARP1 itself, contributes to tubular damage and kidney dysfunction during AKI induced by IRI or cisplatin nephrotoxicity [1418192223], PARP1 activity during IRI was evaluated in the kidneys treated with spermidine or vehicle. Vehicle-treated mouse kidneys showed marked increases in PARP1 activity at 6 hours and 24 hours after IRI, whereas treatment with spermidine significantly diminished the increases in PARP1 activity at both time-points (Fig. 2A). Since PARP1 activation is considered a downstream effector of DNA oxidative and nitrative stress [2728], nitrative and oxidative levels of DNA in these kidneys were assessed using measurements of 8-nitroguanine and 8-hydroxy-2′-deoxyguanosine concentrations, respectively. DNA nitration was time-dependently increased in kidneys of mice treated with vehicle at 6 hours and 24 hours after IRI, but spermidine-treated mouse kidneys showed a significant reduction in DNA nitration at both time-points (Fig. 2B). Intriguingly, DNA oxidation was significantly attenuated by treatment with spermidine at 24 hours after IRI, but not at 6 hours after IRI (Fig. 2C). According to the results of nitration and oxidation of DNA, it is possible to suggest that inhibition of PARP1 activation is caused by the reduction in DNA nitration in IRI-subjected kidneys of spermidine-treated mice, and that the inhibition of PARP1 activation may lead to the reduction in DNA oxidation.
Spermidine is generated from ODC, which catalyzes the decarboxylation of ornithine to form putrescine, and the latter is metabolized to spermidine. Thus, to determine whether spermidine depletion exaggerates IRI in vivo, genetic inhibition of ODC was induced via siRNA transfection into mice. DNA nitration in ODC siRNA (siODC)-transfected mouse kidneys was significantly more enhanced at 6 hours and 24 hours after IRI, compared with that in control siRNA (siControl)-transfected mouse kidneys (Fig. 3A). PARP1 activity in siODC-transfected mouse kidneys was also consistently more increased at both time-points (Fig. 3B). Next, it has been investigated whether ODC inhibition exacerbates histological and functional damage induced by IRI. The data shown in Fig. 3C and D indicate that ODC inhibition significantly increased tubular damage at 6 hours after IRI, but not at 24 hours after IRI, because the score at the time-point in siControl-transfected kidneys almost reached the highest level (5). Levels of plasma creatinine in siODC-transfected mouse kidneys at 6 hours and 24 hours after IRI were significantly higher than those in siControl-transfected mouse kidneys (Fig. 3E). These data indicate that genetic inhibition of ODC exaggerates the consequences of IRI.
To determine whether there is a hierarchy in spermidine depletion, DNA nitration, and PARP1 activation involved in IRI, pharmacological inhibition of PARP1 and supplementation of spermidine were carried out in ODC-knockdown IRI kidneys, respectively. As shown in Fig. 4A and B, spermidine supplement prevented IRI-induced DNA nitration and PARP1 activation in siODC-transfected mouse kidneys. Meanwhile, pharmacological PARP1 inhibition attenuated PARP1 activation in siODC-transfected mouse kidneys, but not DNA nitration. These results suggest that DNA nitration is upstream of PARP1 activation, and spermidine prevents PARP1 activation through DNA nitration in mouse kidneys after IRI. Finally, PARP1 inhibition and spermidine supplement attenuated functional and histological damage induced by IRI in siODC-transfected mouse kidneys (Fig. 4C–E).
Polyamines, spermine and spermidine, suppress IRI in the heart and brain. A previous study of myocardial IRI has demonstrated that exogenous spermine reduces myocyte necrosis and apoptosis through nitric oxide reduction and promotes cardiac functional recovery after IRI [29]. A previous study of ischemic brain has also shown that polyamine supplement delays neuronal cell death in the hippocampus and striatum after IRI [30]. Consistent with the previous studies, the present study on kidney IRI has shown that spermidine supplement attenuates tubular injury and kidney dysfunction after IRI, and further, ODC downregulation induced by siRNA transfection in vivo makes the kidneys more susceptible to IRI. Previous studies have consistently reported that in ODC overexpressing rats and mice, stroke lesions induced by cerebral ischemia were reduced, but treatment with an ODC inhibitor mimicked the lesion response in wild-type ischemic brains [3132].
Why would spermidine regulate histological and functional damage in kidneys after IRI? Acute and severe dysfunction of oxygen delivery in kidneys leads to the generation of oxidative and nitrative stresses after reperfusion [33]. Oxidative and nitrative stresses interact with lipids, nucleic acids, proteins, and carbohydrates to contribute to the development of tubular injury, resulting in kidney dysfunction. As a result, treatment with free radical scavengers or antioxidants ameliorates IRI [3435]. In support of these reports, the present study has shown that the protective effect of spermidine supplement is implicated in oxidative and nitrative stresses in DNA. Furthermore, DNA nitration is attenuated by spermidine during the early and late stages of IRI, whereas DNA oxidation is decreased only in the late stage of IRI; suggesting that spermidine can more effectively inhibit DNA nitration than DNA oxidation following IRI.
PARP1, an important factor in various pathogeneses, is considered a downstream effector of oxidative and nitrative stresses [3637]. Although PARP1 acts as the key enzyme in DNA damage detection and repair, excessive activation of PARP1 leads to pathological necrotic cell death through ATP depletion [38]. Genetic or pharmacological inhibition of PARP1 protects against various kidney diseases, including IRI [39], cisplatin nephrotoxicity [18192223], obstructive nephropathy [40], and tubulointerstitial fibrosis [14]. Consistent with previous studies, the present study has shown that DNA nitration is upstream of PARP1 activation, and the protective effect of spermidine is implicated in inhibition of PARP1 activation through reduced DNA nitrative stress.
Conclusively, the present study shows that spermidine regulates nitrative stress and PARP1 activation during IRI, and leads to protection against histological and functional damage. Exogenous spermidine may constitute a viable strategy for the treatment of AKI.
Acknowledgements
The author thanks Youngsu Cho (JNU) for technical assistance with ELISA. This work was supported by a research grant from the Jeju National University Hospital Research Fund of Jeju National University in 2016.
References
1. Daemen MA, de Vries B, Buurman WA. Apoptosis and inflammation in renal reperfusion injury. Transplantation. 2002; 73:1693–1700. PMID: 12084988.
2. Bonventre JV, Weinberg JM. Recent advances in the pathophysiology of ischemic acute renal failure. J Am Soc Nephrol. 2003; 14:2199–2210. PMID: 12874476.
3. Linkermann A, Bräsen JH, Darding M, Jin MK, Sanz AB, Heller JO, De Zen F, Weinlich R, Ortiz A, Walczak H, Weinberg JM, Green DR, Kunzendorf U, Krautwald S. Two independent pathways of regulated necrosis mediate ischemia-reperfusion injury. Proc Natl Acad Sci U S A. 2013; 110:12024–12029. PMID: 23818611.
4. Eltzschig HK, Eckle T. Ischemia and reperfusion: from mechanism to translation. Nat Med. 2011; 17:1391–1401. PMID: 22064429.
5. Kroemer G, Galluzzi L, Brenner C. Mitochondrial membrane permeabilization in cell death. Physiol Rev. 2007; 87:99–163. PMID: 17237344.
6. Yu SW, Wang H, Poitras MF, Coombs C, Bowers WJ, Federoff HJ, Poirier GG, Dawson TM, Dawson VL. Mediation of poly (ADP-ribose) polymerase-1-dependent cell death by apoptosis-inducing factor. Science. 2002; 297:259–263. PMID: 12114629.
7. Dixon SJ, Lemberg KM, Lamprecht MR, Skouta R, Zaitsev EM, Gleason CE, Patel DN, Bauer AJ, Cantley AM, Yang WS, Morrison B 3rd, Stockwell BR. Ferroptosis: an iron-dependent form of nonapoptotic cell death. Cell. 2012; 149:1060–1072. PMID: 22632970.
8. Pegg AE. Functions of polyamines in mammals. J Biol Chem. 2016; 291:14904–14912. PMID: 27268251.
9. Eisenberg T, Abdellatif M, Schroeder S, Primessnig U, Stekovic S, Pendl T, Harger A, Schipke J, Zimmermann A, Schmidt A, Tong M, Ruckenstuhl C, Dammbrueck C, Gross AS, Herbst V, Magnes C, Trausinger G, Narath S, Meinitzer A, Hu Z, Kirsch A, Eller K, Carmona-Gutierrez D, Büttner S, Pietrocola F, Knittelfelder O, Schrepfer E, Rockenfeller P, Simonini C, Rahn A, Horsch M, Moreth K, Beckers J, Fuchs H, Gailus-Durner V, Neff F, Janik D, Rathkolb B, Rozman J, de Angelis MH, Moustafa T, Haemmerle G, Mayr M, Willeit P, von Frieling-Salewsky M, Pieske B, Scorrano L, Pieber T, Pechlaner R, Willeit J, Sigrist SJ, Linke WA, Mühlfeld C, Sadoshima J, Dengjel J, Kiechl S, Kroemer G, Sedej S, Madeo F. Cardioprotection and lifespan extension by the natural polyamine spermidine. Nat Med. 2016; 22:1428–1438. PMID: 27841876.
10. Eisenberg T, Knauer H, Schauer A, Büttner S, Ruckenstuhl C, Carmona-Gutierrez D, Ring J, Schroeder S, Magnes C, Antonacci L, Fussi H, Deszcz L, Hartl R, Schraml E, Criollo A, Megalou E, Weiskopf D, Laun P, Heeren G, Breitenbach M, Grubeck-Loebenstein B, Herker E, Fahrenkrog B, Fröhlich KU, Sinner F, Tavernarakis N, Minois N, Kroemer G, Madeo F. Induction of autophagy by spermidine promotes longevity. Nat Cell Biol. 2009; 11:1305–1314. PMID: 19801973.
11. Okumura S, Teratani T, Fujimoto Y, Zhao X, Tsuruyama T, Masano Y, Kasahara N, Iida T, Yagi S, Uemura T, Kaido T, Uemoto S. Oral administration of polyamines ameliorates liver ischemia/reperfusion injury and promotes liver regeneration in rats. Liver Transpl. 2016; 22:1231–1244. PMID: 27102080.
12. Adibhatla RM, Hatcher JF, Sailor K, Dempsey RJ. Polyamines and central nervous system injury: spermine and spermidine decrease following transient focal cerebral ischemia in spontaneously hypertensive rats. Brain Res. 2002; 938:81–86. PMID: 12031538.
13. Wang Z, Zahedi K, Barone S, Tehrani K, Rabb H, Matlin K, Casero RA, Soleimani M. Overexpression of SSAT in kidney cells recapitulates various phenotypic aspects of kidney ischemia-reperfusion injury. J Am Soc Nephrol. 2004; 15:1844–1852. PMID: 15213272.
14. Yoon SP, Kim J. Poly(ADP-ribose) polymerase 1 activation links ischemic acute kidney injury to interstitial fibrosis. J Physiol Sci. 2015; 65:105–111. PMID: 25388944.
15. Kim J, Padanilam BJ. Renal denervation prevents long-term sequelae of ischemic renal injury. Kidney Int. 2015; 87:350–358. PMID: 25207878.
16. Kim J, Devalaraja-Narashimha K, Padanilam BJ. TIGAR regulates glycolysis in ischemic kidney proximal tubules. Am J Physiol Renal Physiol. 2015; 308:F298–F308. PMID: 25503731.
17. Kim J, Seok YM, Jung KJ, Park KM. Reactive oxygen species/oxidative stress contributes to progression of kidney fibrosis following transient ischemic injury in mice. Am J Physiol Renal Physiol. 2009; 297:F461–F470. PMID: 19458120.
18. Kim J. Poly(ADP-ribose) polymerase activation induces high mobility group box 1 release from proximal tubular cells during cisplatin nephrotoxicity. Physiol Res. 2016; 65:333–340. PMID: 26447520.
19. Kim J, Long KE, Tang K, Padanilam BJ. Poly(ADP-ribose) polymerase 1 activation is required for cisplatin nephrotoxicity. Kidney Int. 2012; 82:193–203. PMID: 22437413.
20. Yoon SP, Kim J. Exogenous CGRP upregulates profibrogenic growth factors through PKC/JNK signaling pathway in kidney proximal tubular cells. Cell Biol Toxicol. 2017 May 24 [Epub]. DOI: 10.1007/s10565-017-9399-4.
21. Kim J, Padanilam BJ. Renal nerves drive interstitial fibrogenesis in obstructive nephropathy. J Am Soc Nephrol. 2013; 24:229–242. PMID: 23264683.
22. Yoon SP, Kim J. Poly(ADP-ribose) polymerase 1 contributes to oxidative stress through downregulation of sirtuin 3 during cisplatin nephrotoxicity. Anat Cell Biol. 2016; 49:165–176. PMID: 27722009.
23. Park S, Yoon SP, Kim J. Cisplatin induces primary necrosis through poly(ADP-ribose) polymerase 1 activation in kidney proximal tubular cells. Anat Cell Biol. 2015; 48:66–74. PMID: 25806124.
24. Song H, Yoon SP, Kim J. Poly(ADP-ribose) polymerase regulates glycolytic activity in kidney proximal tubule epithelial cells. Anat Cell Biol. 2016; 49:79–87. PMID: 27382509.
25. Lee JS, Lim JY, Kim J. Mechanical stretch induces angiotensinogen expression through PARP1 activation in kidney proximal tubular cells. In Vitro Cell Dev Biol Anim. 2015; 51:72–78. PMID: 25148826.
26. Zahedi K, Lentsch AB, Okaya T, Barone S, Sakai N, Witte DP, Arend LJ, Alhonen L, Jell J, Jänne J, Porter CW, Soleimani M. Spermidine/spermine-N1-acetyltransferase ablation protects against liver and kidney ischemia-reperfusion injury in mice. Am J Physiol Gastrointest Liver Physiol. 2009; 296:G899–G909. PMID: 19164485.
27. Filipovic DM, Meng X, Reeves WB. Inhibition of PARP prevents oxidant-induced necrosis but not apoptosis in LLC-PK1 cells. Am J Physiol. 1999; 277:F428–F436. PMID: 10484526.
28. Krishnakumar R, Kraus WL. The PARP side of the nucleus: molecular actions, physiological outcomes, and clinical targets. Mol Cell. 2010; 39:8–24. PMID: 20603072.
29. Zhao YJ, Xu CQ, Zhang WH, Zhang L, Bian SL, Huang Q, Sun HL, Li QF, Zhang YQ, Tian Y, Wang R, Yang BF, Li WM. Role of polyamines in myocardial ischemia/reperfusion injury and their interactions with nitric oxide. Eur J Pharmacol. 2007; 562:236–246. PMID: 17382924.
30. Gilad GM, Gilad VH. Polyamines can protect against ischemia-induced nerve cell death in gerbil forebrain. Exp Neurol. 1991; 111:349–355. PMID: 1999235.
31. Lukkarinen JA, Kauppinen RA, Gröhn OH, Oja JM, Sinervirta R, Järvinen A, Alhonen LI, Jänne J. Neuroprotective role of ornithine decarboxylase activation in transient focal cerebral ischaemia: a study using ornithine decarboxylase-overexpressing transgenic rats. Eur J Neurosci. 1998; 10:2046–2055. PMID: 9753092.
32. Lukkarainen J, Kauppinen RA, Koistinaho J, Halmekytö , Alhonen LM, Janne J. Cerebral energy metabolism and immediate early gene induction following severe incomplete ischaemia in transgenic mice overexpressing the human ornithine decarboxylase gene: evidence that putrescine is not neurotoxic in vivo. Eur J Neurosci. 1995; 7:1840–1849. PMID: 8528457.
33. Padanilam BJ. Cell death induced by acute renal injury: a perspective on the contributions of apoptosis and necrosis. Am J Physiol Renal Physiol. 2003; 284:F608–F627. PMID: 12620919.
34. Kim J, Kim KY, Jang HS, Yoshida T, Tsuchiya K, Nitta K, Park JW, Bonventre JV, Park KM. Role of cytosolic NADP+-dependent isocitrate dehydrogenase in ischemia-reperfusion injury in mouse kidney. Am J Physiol Renal Physiol. 2009; 296:F622–F633. PMID: 19106211.
35. Kim J, Kil IS, Seok YM, Yang ES, Kim DK, Lim DG, Park JW, Bonventre JV, Park KM. Orchiectomy attenuates post-ischemic oxidative stress and ischemia/reperfusion injury in mice: a role for manganese superoxide dismutase. J Biol Chem. 2006; 281:20349–20356. PMID: 16682413.
36. Obrosova IG, Drel VR, Pacher P, Ilnytska O, Wang ZQ, Stevens MJ, Yorek MA. Oxidative-nitrosative stress and poly(ADP-ribose) polymerase (PARP) activation in experimental diabetic neuropathy: the relation is revisited. Diabetes. 2005; 54:3435–3441. PMID: 16306359.
37. Ungvári Z, Gupte SA, Recchia FA, Bátkai S, Pacher P. Role of oxidative-nitrosative stress and downstream pathways in various forms of cardiomyopathy and heart failure. Curr Vasc Pharmacol. 2005; 3:221–229. PMID: 16026319.
38. Ha HC, Snyder SH. Poly(ADP-ribose) polymerase is a mediator of necrotic cell death by ATP depletion. Proc Natl Acad Sci U S A. 1999; 96:13978–13982. PMID: 10570184.
39. Devalaraja-Narashimha K, Singaravelu K, Padanilam BJ. Poly (ADP-ribose) polymerase-mediated cell injury in acute renal failure. Pharmacol Res. 2005; 52:44–59. PMID: 15911333.
40. Kim J, Padanilam BJ. Loss of poly(ADP-ribose) polymerase 1 attenuates renal fibrosis and inflammation during unilateral ureteral obstruction. Am J Physiol Renal Physiol. 2011; 301:F450–F459. PMID: 21613422.
Fig. 1
Exogenous spermidine attenuates tubular damage and kidney dysfunction during ischemia and reperfusion injury (IRI). Mice were subjected to 30 minutes of bilateral kidney ischemia or sham operation followed by 6 hours or 24 hours of reperfusion. Spermidine (10 mg/kg body weight) or vehicle was administered at 24 hours and 1 hour before ischemia. n=9 mice in each group. (A) Tubular damage in the outer medulla represented by periodic acid-Schiff (PAS) stain in kidney sections of mice. Scale bars=50 µm. (B) Tubular injury score measured in PAS-stained kidney sections. (C) Plasma creatinine concentration. ***P<0.001 vs. vehicle.
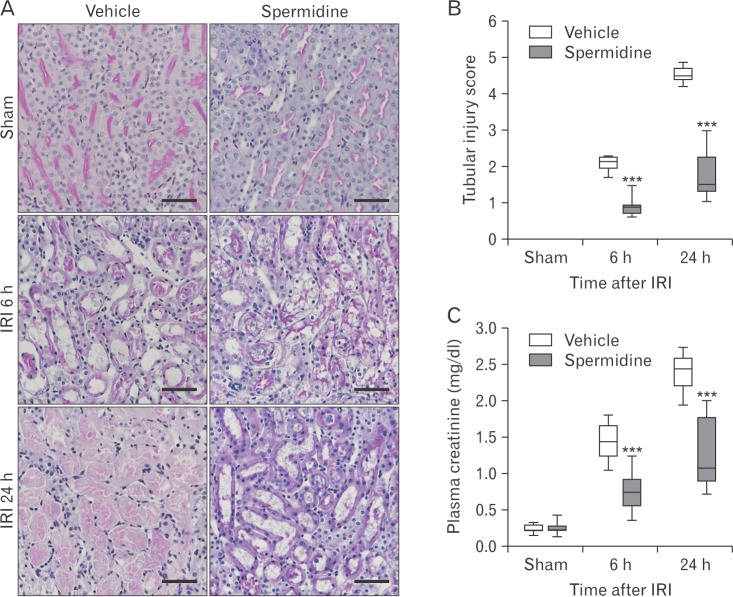
Fig. 2
Exogenous spermidine reduces poly(ADP-ribose) polymerase 1 (PARP1) activation and DNA nitration during ischemia and reperfusion injury (IRI). Mice were subjected to 30 minutes of bilateral kidney ischemia or sham operation followed by 6 or 24 hours of reperfusion. Spermidine (10 mg/kg body weight) or vehicle was administered at 24 hours and 1 hour before ischemia. n=9 mice in each group. (A) PARP1 activity in kidneys. (B) DNA nitration represented by 8-nitroguanine in kidneys. (C) DNA oxidation represented by 8-hydroxy-2′-deoxyguanosine concentration in kidneys. *P<0.05, **P<0.01 and ***P<0.001 vs. vehicle.
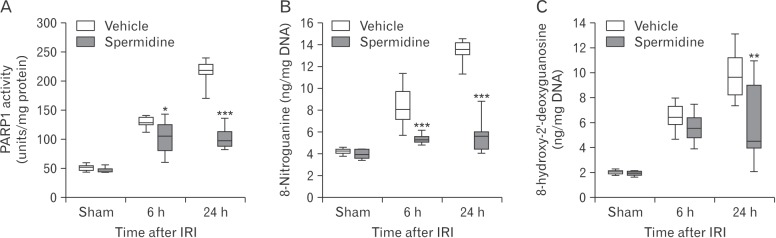
Fig. 3
Ornithine decarboxylase (ODC) siRNA exacervates the consequences of ischemia and reperfusion injury (IRI). Mice were subjected to 30 minutes of bilateral kidney ischemia or sham operation followed by 6 hours or 24 hours of reperfusion. ODC siRNA or control siRNA was injected at 48 hours and 24 hours before ischemia. n=9 mice in each group. (A) DNA nitration represented by 8-nitroguanine in kidneys. (B) Poly(ADP-ribose) polymerase 1 (PARP1) activity in kidneys. (C) Tubular damage in the outer medulla represented by periodic acid-Schiff (PAS) stain in kidney sections of mice. Scale bars=50 µm. (D) Tubular injury score measured in PAS-stained kidney sections. (E) Plasma creatinine concentration. ***P<0.001 vs. siControl.
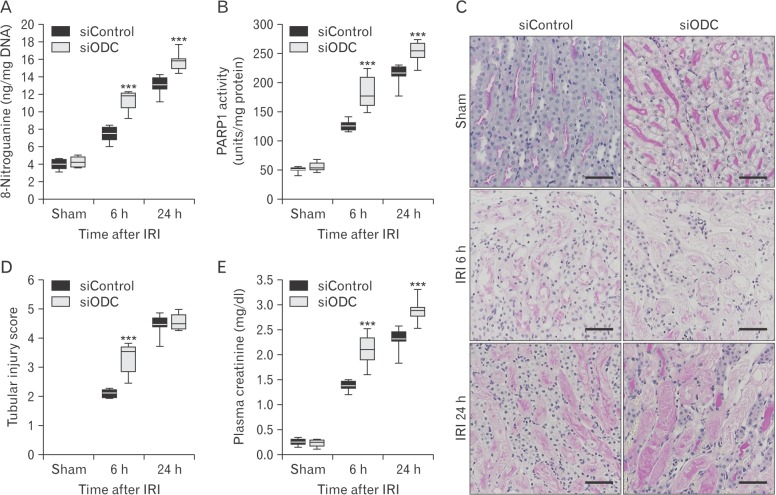
Fig. 4
Exogenous spermidine attenuates tubular damage and kidney dysfunction after ischemia and reperfusion injury (IRI) through inhibiting DNA nitration and poly(ADP-ribose) polymerase 1 (PARP1) activation. Mice were subjected to 30 minutes of bilateral kidney ischemia or sham operation followed by 24 hours of reperfusion. Ornithine decarboxylase (ODC) siRNA was injected at 48 and 24 hours before ischemia. Spermidine (10 mg/kg body weight), PJ34 (10 mg/kg body weight), or vehicle was administered at 24 hours and 1 hour before ischemia. n=9 mice in each group. (A) DNA nitration represented by 8-nitroguanine in kidneys. (B) PARP1 activity in kidneys. (C) Plasma creatinine concentration. (D) Tubular damage in the outer medulla represented by periodic acid-Schiff (PAS) stain in kidney sections of mice. Scale bars=50 µm. (E) Tubular injury score measured in PAS-stained kidney sections. ***P<0.001 vs. vehicle, ##P<0.01, ###P<0.001 vs. PJ34.
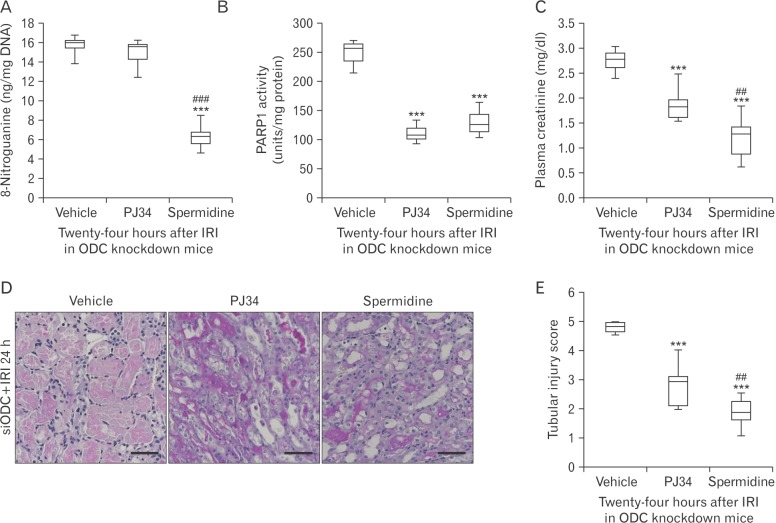