Abstract
Cerebrospinal fluid (CSF) contains several molecules which are essential for neurogenesis. Human dental pulp stem cells (hDPSCs) are putatively neural crest cell-derived that can differentiate into neurons and glial cells under appropriate neurotrophic factors. The aim of this study was to induce differentiation of hDPSCs into neuroglial phenotypes using retinoic acid (RA) and CSF. The hDPSCs from an impacted third molar were isolated by mechanical and digestion and cultured. The cells have treated by 10−7 µM RA (RA group) for 8 days, 10% CSF (CSF group) for 8 days and RA with CSF for 8 days (RA/CSF group). Nestin, microtubule-associated protein 2 (MAP2), and glial fibrillary acidic protein immunostaining were used to examine the differentiated cells. Axonal outgrowth was detected using Bielschowsky's silver impregnation method and Nissl bodies were stained in differentiated cells by Cresyl violet. The morphology of differentiated cells in treated groups was significantly changed after 3–5 days. The results of immunocytochemistry showed the presence of neuroprogenitor marker nestin was seen in all groups. However, the high percentage of nestin positive cells and MAP2, as mature neural markers, were observed at the pre-induction and induction stage, respectively. Nissl bodies were detected as dark-blue particles in the cytoplasm of treated cells. Our findings showed the RA as pre-inducer and CSF as inducer for using in vitro differentiation of neuron-like cells and neuroglial cells from hDPSCs.
Stem cell replacement is a pivotal subject issue in neuroscience because of the inability of central neurons to regenerate correct axonal and dendritic connections [123]. In 2000, a new group of adult mesenchymal stem cells, namely as dental stem cells were found to be the source of embryonic and mesenchymal cells with different biological characteristics [456]. These cells can be derived from human dental pulp and periodontal ligament. The Human dental pulp stem cells (hDPSCs) are the migration output of the neural crest cell with ectodermal origin (cranial neural crest) to the mouth area [7]. These ecto-mesenchymal cells have important features including high proliferation, easy access, self renewal ability, desertion from the host immune system and vigorous cloning. In addition hDPSCs are able to differentiate into multiple lineages including neurogenic, osteogenic, and myogenic [5789].
As hDPSCs is embryonically close to the source of nerve cells and also has neuronal morphology with expression of primary neural specific markers, they are introduced as an ideal source for the treatment of neurological diseases and disorders [578910111213]. These stem cells express primary neural progenitor markers such as nestin and glial fibrillary acidic protein (GFAP) in both gene and protein levels without neural inducer in culture [910111213141516].
Retinoic acid (RA) is an important factor in the primary neural developmental environment and has biological effects in culture for example, transcription regulation and receptor expression of neurotrophic factor on the surface of differentiating cells [17]. However, the ratio of positive neuron like cells is low and not responsible for the clinical application [1617].
Since the embryonic life of each person, cerebrospinal fluid (CSF) flows within the ventricles and plays an important role in brain development, drainage of nervous system, metabolic materials and critical signaling factors in different parts of the central nervous system (CNS). As well, CSF affects neuroectodermal cells during development [181920]. Due to direct contact of CSF with the choroid plexus and neural stem cells in embryonic time, it can accommodate and trade many substances, including growth factors, cytokines, extracellular matrix proteins, adhesion molecules and other active ingredients which are efficient in proliferation and differentiation [2021]. It is demonstrated that of CSF contains many neurotrophic and growth factors [151619202122]. For example, secreted poly-peptides from fetal choroid plexus into the CSF are interlukin-6, ciliary neurotrophic factor (CNTF), transforming growth factor β, nerve growth factor (NGF), insulin growth factor, brain derived neurotrophic factor (BDNF), and neurotrophin 3 which are essential for differentiation, proliferation, repair of nerves and brain development [182122].
The in vitro studies suggest CSF, as media culture, is capable of generating neural stem cell neurosphere in a culture with no other additional factors [16]. Moreover, according to the critical role of CSF in the process of brain development and the essential metabolic contents, it is likely to be effective in the differentiation of stem cells into glial cells and mature nerve cells [21]. Although many studies on CSF has been performed, the mechanism and differential effects are yet to be identified.
The aim of this study was to investigate the effect of two steps differentiation manner with CSF and RA on hDPSCs. By optimizing the differentiation of these cells in laboratory conditions, a normal developmental progression to mature and electrically active neurons, and also appropriate autologous cells for neurological disease treatment is obtained.
In this study hDPSCs was extracted from human third molar. Healthy teeth with no cavities of patients 18–25 years old were collected from dental clinic of Mazandaran University of Medical Sciences and were transferred in phosphate-buffered saline (PBS) to the research laboratory. In order to disinfect teeth, iodine (povidone iodine 10%, Pejnan, Iran) was used for 5 minutes. Then, separator and surgery cutter were utilized for dividing the root and dentin and pink pulp tissue separated by sterile forceps. Mechanical and enzymatic digestion were conducted by using scalpel and trypsin 0.25% (Gibco, Gran Island, NY, USA), respectively. In the next step, tissues with trypsin and DMEM/F12 (Gibco-Life Technologies, Invitrogen, Paisley, Scotland) were poured in 15-ml falcon tube and kept in incubator for 5 minutes. After this stage, the tissue pieces and trypsin in the Falcon tube was centrifuged, the supernatant was removed and tissues were transferred into 25-ml cell culture flasks containing medium and incubated at 37℃ and 5% CO2 and humidity conditions of 95%. The cell culture flask was examined every 2–3 days under an inverted microscope. To purify hDPSCs, passages 3–4 were used [11].
CSF was taken from cisterna magna of newborn rat race Sprague-Dawley with glass micropipettes and transferred to the sterile micro tubes. Then samples were centrifuged at 1,400 rpm to remove cells and, debris from the fluid or blood clot and the supernatant were transferred into another sterile microtube and stored at −80℃ [151623].
Dose response and time course were assessed to obtain optimal CSF and RA concentration by evaluating the viability rate of cells by dimethylthiazolyldiphenyl-tetrazoliumbromide (MTT) assay. To find the best dose and the best time of using CSF, the hDPSCs were exposed to culture medium containing the CSF concentrations of in 0%, 2.5%, 5%, 10%, and 20% for 8 days. In the next step, the 96-well plates were incubated with MTT (Sigma-Aldrich, St. Louis, MO, USA; 5 mg/ml in PBS) for 3 hours at 37℃. Then formazon insoluble cristals was solved in dimethyl sulfoxide (Sigma) and finally the absorbance of formazon products was determined by Eliza plate reader (Synergy H11, BioTek, Winooski, VT, USA) at 570 nm [20].
For induction process hDPSCs, were plated into 6-well plates. After the adhesion of cells to the bottom of the plate, CSF (based on the results of a viability test) and RA (Sigma-Aldrich) were added to the wells for 8 days as follows. The hDPSCs were cultured in medium (control group). RA 10−7 µm (RA group) and CSF 10% (CSF group) were added to some wells separately. Some of wells were treated with RA and CSF 10% for 8 days (RA/CSF group). Morphological assessment of differentiating cells were analyzed in different groups by inverted microscope (Eclipse-TS100, Nikon, Tokyo, Japan) on a daily basis.
The expression of nestin and microtubule-associated protein 2 (MAP2) neuronal markers and GFAP glial marker were examined by immunocytochemical assessment. Cell groups were washed with PBS for 5 minutes, then were fixed in 4% paraformaldehyde for 15 minutes and after that the cells were washed with PBS again. In the next step, hDPSCs were permeabilized with 1% Triton X-100 (Sigma-Aldrich) for 30 minutes in room temperature and blocked with bovine serum albumin (Sigma-Aldrich) for 45 minutes. Then the cells were incubated with nestin (rabbit polyclonal to nestin, ab92391, Abcam System, Cambridge, UK), MAP2 (rabbit monoclonal to MAP2, ab183830, Abcam System) and GFAP (goat polyclonal to GFAP, ab53554, Abcam System) primary antibodies for 1 hour. After three times washes with PBS, appropriate secondary antibodies (donkey anti-rabbit phycoerythrin, ab7007, Abcam System and donkey anti-goat FITC, ab6881, Abcam System) were added for 1 hour at 37℃. Finally, DAPI (Sigma-Aldrich) were used for cell nuclear staining and examined by fluorescent microscopy (Eclipse-TE600, Nikon) [56722].
In order to identify differentiated cells, two specific staining was used as follow.
The Nissl bodies were identified by crystal violet specific staining that was performed at 8 days. Then, the previously created hDPSCs containing pink Nissl bodies, were studied with light microscope.
The length measurement of the cell axons (neurites) was examined by Bielschowsky's silver impregnation method. The differentiated cells were washed with distilled water. Then, silver nitrate and ammoniacal silver nitrate solutions were used for 15 and 30 minutes at 40℃, respectively. The cell plates were exposed to the developer solution (mixture of formaldehyde, citric acid, nitric acid, ammonia, and water) and ammonium hydroxide for 1 minute and then washed in distilled water. To stop the reaction, sodium thiosulfate solution was poured for 5 minutes and then the cells were washed again. The differentiated cells with dark brown to black neuritis were considered to measure the length of largest axons by the software Image J 1.41 [24].
After extraction process, viable cells with elongated shapes were detected 1 day after the onset of culturing. These cells with colony formation, filled bottom of the flask and then subcultured (first passage). The subcultured cells gradually became flattened and acquired an indistinguishable fibroblast-like morphology. The hDPSCs had polymorphic shape in first passage (Fig. 1A).
Morphological changes were observed in all groups. These include the appearance of cells with bipolar and multipolar cell body, large euchromatin core with clear nuclei and aggregation of granules in cytoplasm. During the induction course in some group's fusiform cells with axon like and dendritic processes or neuritis in differentiated cells were seen (Fig. 1B).
The results of Bielschowsky's silver impregnation method showed the presence of axon like and dendritic processes or neuritis in differentiated cells (Fig. 1C). The hDPSCs and transdifferentiated hDPSCs with positive reaction of Nissl bodies were detected in all groups by crystal violet staining (Fig. 1D). But the mean percentage of cells with neuronal phenotype were significantly higher in the RA/CSF group than the hDPSCs groups (Fig. 2). In addition the most neuronal phenotype cells were observed in the RA/CSF group that is significantly higher than the other groups (Fig. 2).
The mean absorbance of treated cells shown a higher viability in control group compared to treated group. As well, after 8 days CSF 10% gave a significant increase in viability compared to those observed in other experimental groups (Fig. 3).
Nestin, MAP2, and GFAP markers were used for identification of neural progenitor, mature nerve and astrocyte cells, respectively. In addition, the number of positive neural and glial cells were assessed by ImageJ software in different groups (Fig. 4). The maximum percentage of nestin marker expression was in RA-CSF/4 days and CSF/8 days group at fourth and eighth days [2526]. As well, the evidence of MAP2 marker showed that in RA-CSF/8 days group were significantly higher than other groups. GFAP markers in RA-CSF/8 days and RA/8 days groups, respectively were significantly higher than other groups (Figs. 4, 5).
Neurogical disorders are one of the serious abnormalities which causes severe complications and disability for people. One of the important strategies for these patients is stem cell therapy. Modern science of cell therapy is solving special problems, including use of allogeneic cells and cell differentiation into active nerve cells [1023242728].
The hDPSCs could provide new hope in this area. These cells have been the focus of attention because of some features including easy access and non-invasive extraction with no ethical problems. In addition, due to lack of stimulating lymphocytes of immune, the hDPSCs are suitable for autologous transplantation. Additionally, because of neural crest origin of these ectomesenchymal cells and embryological proximity with the nervous system, it is likely these cells have higher potential than other mesenchymal cells to become neurons and glia. Therefore, they introduce as the ideal source to treat diseases and disorders of the nervous system [125678].
In this study, the hDPSCs were used. In order to assess the efficacy of suitable neural differentiation, two step neural induction were used. The experimental results showed that the fibroblast-like phenotype, spindle-shaped cells, high adhesion and proliferation, and high-quality colony formation of these cells, were maintained during sequential passages.
In 2011, a study was conducted to evaluate the capability of hDPSCs proliferation and differentiation. The results showed that these cells survive in successive passages without losing their ability to proliferate. In addition, differentiated neuron-like cells with various forms appear shortly after the interval adding inducing factors in culture [89101416].
CSF is in direct contact with stem cell niche of CNS, thus this liquid contains some compounds including growth and neurotrophic factors, cytokines, binding molecules and extracellular matrix proteins and could play a significant role in the evolution of CNS [2324272829]. Therefore, our study showed that adding CSF in culture as inducer medium provided suitable nutrient microenvironment for survival, proliferation and differentiation of hDPSCs. Overall, results of the present study suggest that the cytotoxicity of the CSF will affect the survival rate of the cells in a dose-dependent pattern [202122].
These findings are consisted with previous studies conducted. In this connection, Lehtinen et al. in 2011 [16] showed that the CSF has proliferation-inducing factors that could contribute to proliferation and differentiation of neural progenitor cells. In addition, there are numerous reports indicating that macro-molecules available in the CSF lead to the formation of the signal cascade altering the expression of transcription factors and genes and impact on some of the vital functions of cells. The hDPSCs secret neurotrophic growth factors in culture media such as BDNF, CNTF, and NGF that cause neuronal survival and neurite growth in vitro which, in turn, positive impacts of CSF is doubled in vitro [1415161718192021].
In addition, the results of immunohistochemical findings demonstrate that the use of neurogenic inducer factors improve neural differentiation pathways, as neural-like differentiated cells from hDPSCs express specific markers such as nestin and MAP2 that is proved by immunofluorescence staining and cell count. Nestin marker expressing cells were observed in control and experimental groups indicating that that the inductor elements used in this study have the capacity of neurogenesis stimulation. However, the presence of neural progenitor cells in the control group were not expected that maybe due to the nature of neural crest-derived stem cells from human dental pulp which was appeared in the media spontaneously. Maximum neural progenitor cells were observed in the RA/CSF group.
In the present study, the possible cause of increase in nestin-expressing cells in RA/CSF group compared to other groups, was the expression of a set of neurogenesis associated genes such as Sonic hedgehog and transcription factors such as Pax6 and Pax7. These events are under effect of RA in culture that cause primary proliferation and differentiation of neural progenitor cells derived from hDPSCs [1314151620212728]. Additionally, due to the neural cell purification of RA, it is desirable in pre-induction stage. In this subject, the current research is in agreement with the results obtained by Darabi et al. in 2013 [7].
In the process of neurogenic induction in this study, the most remarkable factor is MAP2 expressing cells as mature nerve cells in the groups and interestingly most of them were in presence of CSF, and especially in the induction phase of RA/CSF groups. But, the main factors that are responsible for the behavior of CSF distinction are bone morphogenic proteins (BMPs). They activate or inhibit specific molecular pathways within cells. It can be noted that BMP4 activate the protein chain called extracellular signal-regulated kinases (ERK). These proteins (ERK) act as signal transmitter from cell surface receptors to the cell nucleus and nucleic acid, leadings to the expression of different enzymes and proteins and providing the neural differentiation of neural progenitor cells [2122232427282930].
However, one of the most important things that must be considered in vitro induction of neural differentiation, is simulation of neuroectodermal differentiation condition in the fetal stages. In other words, the cell microenvironment plays an important role in the differentiation of mesenchymal stem cells into nerve cells [19242728]. However, the less presence of neural progenitor cells in the CSF medium and the presence of mature nerve cells in the induction phase of RA/CSF group can attribute to the important function of CSF similar to the embryonic period and neurotrophic factors and other compounds in the CSF that causes multiple intracellular molecular pathway cascades. Therefore, the cells pass the pre-neuronal stage and differentiate into mature neurons in induction culture.
Cytological evaluation results confirmed the neural phenotype of the cells. In the present study, specific staining was used to examine the forms of differentiated cells and identify cellular neuritis that changed during differentiation. The results showed the twin effect of RA and CSF on the human ectomesenchymal cells and caused Nissl body aggregations and created long cellular neuritis that expresses the neural characteristics of differentiated cells.
In associated with glial differentiation, it would be noted that the cells with different shapes, expressing the GFAP marker and negative response to crystal violet and silver nitrate staining were higher in the experimental group. In this way, Buddensiek et al. in 2010 [3] were conducted a study on the effects of CSF distinction [2930]. They showed that CSF is a strong astroglial stimulator on neural stem cells (NSC) in culture. They used adult CSF and observed high survival rate of NSCs, related with survival results of this study. On the other hand, BMP7 is one of the factors in the CSF that plays a significant role in neuritis elongation. Thus, induction of neurogenesis differentiation in vitro (similar to in vivo), the need to conflict or interfere molecules, different neurotrophic factors and special and consecutive pathways [15162324272830].
In this study, it has been shown that using a differentiation inducing factors including RA and CSF, can remove other unwanted cells of differentiation and help the homogenization of neurons and glial cells and in this way the neurogenesis and gliogenesis induction could be optimized in vitro.
Figures and Tables
Fig. 1
Phase contrast photography. (A) Fibroblast-like morphology and colony derived human dental pulp stem cells (hDPSCs) at the first passage. (B) Neural differentiation of hDPSCs in experiment groups with retinoic acid (RA) and cerebrospinal fluid (CSF) after 8 days. (C) Bielschowsky's silver impregnation method in experiment groups with RA and CSF after 8 days. (D) Cresyl violet specific staining method in experiment groups with RA and CSF after 8 days. Scale bars=50 µm.
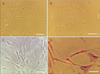
Fig. 2
Presents the histogram of the mean percentage of the human dental pulp stem cells (hDPSCs) and transdifferentiated hDPSCs using cerebrospinal fluid (CSF) and retinoic acid (RA) as an inducers by crystal violet staining method. hDPSCs and transdifferentiated hDPSCs with positive reaction of Nissl bodies were detected in all groups. Asterisk showed the most neuronal phenotype cells was observed in the RA/CSF group that is significantly higher than the other groups (*P<0.05).
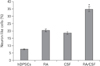
Fig. 3
Protective effects of cerebrospinal fluid (CSF) treatment on cell viability. The dose–response human dental pulp stem cells (hDPSCs)–derived neural stem cell viability at different concentrations of CSF (2.5%, 5%, 10%, and 20%) after 8 days detected by dimethylthiazolyldiphenyl-tetrazoliumbromide (MTT) assay. Results show the mean percent viability (relative to untreated cells). Each bar represents the average measurement from five replicates.
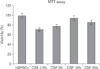
Fig. 4
Immunohistochemistry of transdifferentiated human dental pulp stem cells (hDPSCs) using cerebrospinal fluid and retinoic acid as an inducers. (A) Transdifferentiated hDPSCs immunostained with anti–microtubule-associated protein 2 antibody (primary) and incubated with Alexa Fluor (red) secondary antibody. (B) Transdifferentiated hDPSCs immunostained with mouse anti–glial fibrillary acidic protein monoclonal antibody (primary) and incubated with rabbit anti-mouse IgG antibody conjugated with FITC (green). (C) Transdifferentiated hDPSCs immunostained with mouse anti-nestin monoclonal antibody (primary), and incubated with goat anti-mouse IgG to nestin (Alexa Fluor) secondary antibody. DAPI (Sigma-Aldrich) were used for cell nuclear staining and examined by fluorescent microscopy (Nikon, Eclipse-TE600, Japan). Scale bars=200 µm.
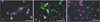
Fig. 5
Presents the histogram of the mean percentage of the immunoreactive cells to nestin, microtubule-associated protein 2 (MAP2), and glial fibrillary acidic protein (GFAP) of the human dental pulp stem cells (hDPSCs) and hDPSCs induced with with 10−7 µM retinoic acid (RA) and cerebrospinal fluid (CSF) 10% using the time course (4 and 8 days). Asterisk shows significantly different from the other groups with the same antibody (P<0.05).
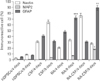
Acknowledgements
This project was funded by a grant from Mazandaran University of Medical Sciences, Sari, Iran (grant No.1394- 140) and Iranian council of stem cell technology.
References
1. Arthur A, Rychkov G, Shi S, Koblar SA, Gronthos S. Adult human dental pulp stem cells differentiate toward functionally active neurons under appropriate environmental cues. Stem Cells. 2008; 26:1787–1795.
2. Nazm Bojnordi M, Movahedin M, Tiraihi T, Javan M. Alteration in genes expression patterns during in vitro differentiation of mouse spermatogonial cells into neuroepithelial-like cells. Cytotechnology. 2013; 65:97–104.
3. Buddensiek J, Dressel A, Kowalski M, Runge U, Schroeder H, Hermann A, Kirsch M, Storch A, Sabolek M. Cerebrospinal fluid promotes survival and astroglial differentiation of adult human neural progenitor cells but inhibits proliferation and neuronal differentiation. BMC Neurosci. 2010; 11:48.
4. Chang CC, Chang KC, Tsai SJ, Chang HH, Lin CP. Neurogenic differentiation of dental pulp stem cells to neuron-like cells in dopaminergic and motor neuronal inductive media. J Formos Med Assoc. 2014; 113:956–965.
5. Darabi S, Tiraihi T, Delshad A, Sadeghizadeh M. A new multistep induction protocol for the transdifferentiation of bone marrow stromal stem cells into GABAergic neuron-like cells. Iran Biomed J. 2013; 17:8–14.
6. Bojnordi MN, Azizi H, Skutella T, Movahedin M, Pourabdolhossein F, Shojaei A, Hamidabadi HG. Differentiation of spermatogonia stem cells into functional mature neurons characterized with differential gene expression. Mol Neurobiol. 2016; 09. 19. [Epub]. DOI: 10.1007/s12035-016-0097-7.
7. Darabi S, Tiraihi T, Ruintan A, Abbaszadeh HA, Delshad A, Taheri T. Polarized neural stem cells derived from adult bone marrow stromal cells develop a rosette-like structure. In Vitro Cell Dev Biol Anim. 2013; 49:638–652.
8. Engberg N, Kahn M, Petersen DR, Hansson M, Serup P. Retinoic acid synthesis promotes development of neural progenitors from mouse embryonic stem cells by suppressing endogenous, Wnt-dependent nodal signaling. Stem Cells. 2010; 28:1498–1509.
9. Gato A, Moro JA, Alonso MI, Bueno D, De La Mano A, Martín C. Embryonic cerebrospinal fluid regulates neuroepithelial survival, proliferation, and neurogenesis in chick embryos. Anat Rec A Discov Mol Cell Evol Biol. 2005; 284:475–484.
10. Gronthos S, Mankani M, Brahim J, Robey PG, Shi S. Postnatal human dental pulp stem cells (DPSCs) in vitro and in vivo. Proc Natl Acad Sci U S A. 2000; 97:13625–13630.
11. Huang AH, Snyder BR, Cheng PH, Chan AW. Putative dental pulp-derived stem/stromal cells promote proliferation and differentiation of endogenous neural cells in the hippocampus of mice. Stem Cells. 2008; 26:2654–2663.
12. Karaöz E, Demircan PC, Sağlam O, Aksoy A, Kaymaz F, Duruksu G. Human dental pulp stem cells demonstrate better neural and epithelial stem cell properties than bone marrow-derived mesenchymal stem cells. Histochem Cell Biol. 2011; 136:455–473.
13. Karaoz E, Dogan BN, Aksoy A, Gacar G, Akyüz S, Ayhan S, Genç ZS, Yürüker S, Duruksu G, Demircan PC, Sariboyaci AE. Isolation and in vitro characterisation of dental pulp stem cells from natal teeth. Histochem Cell Biol. 2010; 133:95–112.
14. Laino G, d'Aquino R, Graziano A, Lanza V, Carinci F, Naro F, Pirozzi G, Papaccio G. A new population of human adult dental pulp stem cells: a useful source of living autologous fibrous bone tissue (LAB). J Bone Miner Res. 2005; 20:1394–1402.
15. Lehtinen MK, Walsh CA. Neurogenesis at the brain-cerebrospinal fluid interface. Annu Rev Cell Dev Biol. 2011; 27:653–679.
16. Lehtinen MK, Zappaterra MW, Chen X, Yang YJ, Hill AD, Lun M, Maynard T, Gonzalez D, Kim S, Ye P, D'Ercole AJ, Wong ET, LaMantia AS, Walsh CA. The cerebrospinal fluid provides a proliferative niche for neural progenitor cells. Neuron. 2011; 69:893–905.
17. Lei M, Li K, Li B, Gao LN, Chen FM, Jin Y. Mesenchymal stem cell characteristics of dental pulp and periodontal ligament stem cells after in vivo transplantation. Biomaterials. 2014; 35:6332–6343.
18. Martens W, Bronckaers A, Politis C, Jacobs R, Lambrichts I. Dental stem cells and their promising role in neural regeneration: an update. Clin Oral Investig. 2013; 17:1969–1983.
19. Mashayekhi F, Azari M, Moghadam LM, Yazdankhah M, Naji M, Salehi Z. Changes in cerebrospinal fluid nerve growth factor levels during chick embryonic development. J Clin Neurosci. 2009; 16:1334–1337.
20. Nabiuni M, Rasouli J, Parivar K, Kochesfehani HM, Irian S, Miyan JA. In vitro effects of fetal rat cerebrospinal fluid on viability and neuronal differentiation of PC12 cells. Fluids Barriers CNS. 2012; 9:8.
21. Nakashima M, Iohara K, Sugiyama M. Human dental pulp stem cells with highly angiogenic and neurogenic potential for possible use in pulp regeneration. Cytokine Growth Factor Rev. 2009; 20:435–440.
22. Nazm Bojnordi M, Ghasemi HH, Akbari E. Remyelination after lysophosphatidyl choline-induced demyelination is stimulated by bone marrow stromal cell-derived oligoprogenitor cell transplantation. Cells Tissues Organs. 2015; 200:300–306.
23. Tehranipour M, Baharara J, Mostafaee M. The neuroprotective effect of CSF intraperitoneal injection on alpha motor degeneration after sciatic nerve compression in rat. Arak Med Univ J. 2009; 12:101–108.
24. Otify DY, Youssef EA, Nagy NB, Marei MK, Youssif MI. Transdifferentiation of bone marrow mesenchymal stem cells into neural cells via cerebrospinal fluid. Biomed Biotechnol. 2014; 2:66–79.
25. Nazm Bojnordi M, Movahedin M, Tiraihi T, Javan M, Ghasemi Hamidabadi H. Oligoprogenitor cells derived from spermatogonia stem cells improve remyelination in demyelination model. Mol Biotechnol. 2014; 56:387–393.
26. Ghasemi Hamidabadi H, Rezvani Z, Nazm Bojnordi M, Shirinzadeh H, Seifalian AM, Joghataei MT, Razaghpour M, Alibakhshi A, Yazdanpanah A, Salimi M, Mozafari M, Urbanska AM, Reis RL, Kundu SC, Gholipourmalekabadi M. Chitosan-intercalated montmorillonite/poly(vinyl alcohol) nanofibers as a platform to guide neuronlike differentiation of human dental pulp stem cells. ACS Appl Mater Interfaces. 2017; 9:11392–11404.
27. Osathanon T, Nowwarote N, Pavasant P. Basic fibroblast growth factor inhibits mineralization but induces neuronal differentiation by human dental pulp stem cells through a FGFR and PLC-gamma signaling pathway. J Cell Biochem. 2011; 112:1807–1816.
28. Salehi Z, Mashayekhi F, Naji M, Pandamooz S. Insulin-like growth factor-1 and insulin-like growth factor binding proteins in cerebrospinal fluid during the development of mouse embryos. J Clin Neurosci. 2009; 16:950–953.
29. Yu J, He H, Tang C, Zhang G, Li Y, Wang R, Shi J, Jin Y. Differentiation potential of STRO-1+ dental pulp stem cells changes during cell passaging. BMC Cell Biol. 2010; 11:32.
30. Zappaterra MW, Lehtinen MK. The cerebrospinal fluid: regulator of neurogenesis, behavior, and beyond. Cell Mol Life Sci. 2012; 69:2863–2878.