Abstract
It has been suggested that proteasome system has a role in initiation, progression, and complication stages of atherosclerosis. Although there is still controversy, there has been no research that compares the expression of proteasome in tissue and serum at each of these stages. This study aimed to investigated the expression of proteasome at different stages of atherosclerosis using rat model. We measured the expression of aortic proteasome by immunohistochemical analyses and were then analyzed using ImageJ software for percentage of area and integrated density. We used Photoshop version 3.0 to analyze aortic proteasome expression as a comparison. We measured serum proteasome expression by enzyme linked immunosorbents assays. Kruskal-Wallis test was used to compare mean value of percentage of area and serum proteasome. Analysis of variance test was used to compare mean value of integrated density. Correlation test between vascular proteasome expression and serum proteasome expression was made using Spearman test. A P-value of 0.05 was considered statistically significant. Compared with normal, percentage of area was higher in initiation, progression, and complication. Compared with normal, integrated density was higher in initiation and further higher in progression and complication. Data from Image J is similar with data from Photoshop. Serum proteasome expression was higher in initiation compared with normal, and further higher in progression and complication. It was concluded that there were different vascular proteasome expression and serum proteasome expression at the stages of atherosclerosis. These results may be used in research into new marker and therapeutic target in atherosclerosis.
Currently, atherosclerosis is a growing problem because it has high risk cardiovasculer diseases (CVD). CVDs contribute 30% cause of death in worldwide and 35% cause of death at United States [1]. In United States, mortality rate occurs in 1/39 second in average because of CVD [2]. Atherosclerosis is inflamation process, which mainly affects medium size arteries. The decreased of shear stress and turbulence of blood flow can lead to atherogenesis. Therefore, typical atherosclerotic plaques occur in branching regions, winding vessels and areas where there are some changes in velocity and direction of sudden blood flow. Blood vessels with similar characteristics include the carotid artery, coronary, large branches of the thoracic and abdominal aortic, and great vessels of the lower limb [34].
Atherosclerosis begins with a long asymptomatic phase which is recognized from the apperance of fatty streaks in early adulthood, and the progression lead to atheromatous plaques in fourth and fifth decade. Further, this disease progression may results in complicated plaques with the risk of rupture and thrombosis [5]. Therefore, it is important to figure out the pathogenesis of atherosclerosis, so that atherosclerosis can be detected earlier and can be treated then it may not causes complication [6].
At the initiation stage of atherosclerosis, endothelial dysfunction and endothelial activation occurs due to the increase of oxidative stress and inflammation. Stage progression of atherosclerosis is characterized by the formation of a layer of foam cells and vascular smooth muscle cells (VCMCs) proliferation. Stage complication of atherosclerosis is characterized by plaque rupture, thrombus formation, and blockage of blood vessels which is complete or not complete. This happens due to a decrease in cell viability and an increase in the destructive inflammatory response [7].
There are three predominat mechanisms at each stage of atherosclerosis, called inflammation, proliferation and apoptosis mechanism. To analyze whether those processes have been occured, a particular parameter is required to reflect these three parameters. Literatures reveal, proteasome, a subcellular particle has an important role in those processes [8]. The research is also demonstrate that proteasome is not only function in intracell but also extracell such as plasma/serum [9]. Many researches also support proteasome's role in initiation, progression and atheroslerosis complication stages [8].
Proteasome is an enzyme complex that responsible for the non-lysosomal degradation of the majority of intracellular proteins, that plays a crucial role in the regulation of many cellular processes. This complex is composed by a 20S core particle which embodies the catalytic activity and two 19S regulatory particles. Proteasome plays a role in cell cycle regulation, apoptosis, cell division, growth, and signal transduction. In addition, the proteasome also plays a role in the regulation of protein quality by destroying damaged, oxidized and misfolded protein. These processes play an important role in the initiation, progression, and complication of atherosclerosis, although some controversion are still exist [10].
Rats are used in this research as experimental animal. Various experimental animals have been used for the study of atherosclerosis such as primates, pigs, rabbits, guinea pigs, rats, and mice. Small experimental animals such as rat is promising for use in the study of atherosclerosis. Rats have some advantages as a practical, easy to obtain and are omnivorous diet rats, more like humans. Using rats as the experimental animal has more advantages than using rabbits. Although the rats are resistant to atherosclerosis, but by conducting some modifications such as providing tiourasil, vitamin D3 or adrenaline in conjunction with high-cholesterol diet, the rat can be induced to be the atherosclerosis [111213]. The predilection of blood vessels location where the atherosclerosis occurs in rat is the aorta [1415].
Conflicting results of in vitro and in vivo studies have remainded some in questions with regard to the feasibility of proteasome inhibitor in treatment of atherosclerosis. Various studies show the role of the proteasome at every stage of atherosclerosis. There is no study comparing the role of the proteasome in each of these stages [16]. The current study has been designed to figure out more about the proteasome expression in atherosclerotic lesions and serum at different stages of atherosclerosis. We examined the protesome expression of normal and atherosclerosis regions of rat's abdominal aortic by immunochemical analyses. Serum proteasome expression was measured using enzyme linked immunosorbents assays (ELISA) to figure out more about the proteasome activity in serum.
Twenty-eight healthy male Wistar rats, 10 weeks of age, obtained from Faculty of Science, Andalas University. Rats were treated in accordance with the Helsinki convention. Ethical approval was obtained from the Research Ethics Committee of the Faculty of Medicine, Andalas University. We aimed to minimized the number of animal used and avoid animal suffering.
Rats were placed in cages in a room with proper ventilation, room temperature between 20–26℃ and humidity. Lighting of the room was regulated light and dark for 12 hours. Rat cages were cleaned every day and the rat health was well maintained. All rats were fed with standard diet for 2 weeks to be adapted for the environment. Rats were randomly divided into 4 groups. Group 1, control group, were fed with standard diet. Group 2, 3, and 4, rats fed with high lipid diet (2% cholesterol, 5% goat fat, 0.2% cholic acid, Sigma, St. Louis, MO, USA) and standard diet up to 100% and were given vitamin D3 (700,000 IU/kg, Biotech Pharmacal, Salt Lake City, UT, USA) in the first day by intragastric feeding [1718]. The treatment duration for high lipid diet was 2 days for group 2, 4 days for group 3, and 2 weeks for group 4.
At the end of the experiment, the rats were sacrificed with ether anasthesia. Subsequently, abdominal aortic tissues were rapidly excised. We fixed the aortic sample in 10% neutralized formaldehyde in 0.1 M phosphate buffer and embedded in paraffin. A hematoxylin and eosin (H&E) staining was performed to observe the morphological changes in the abdominal aortic in rats. H&E slides were examined by two independent pathologists in order to classify them as the normal, initiation stage, progression stage, and complication stage. In the aorta, atherosclerosis was scored as follows: 0, normal; 1, widening of elastic fibers with few foam cells; 2, fragmentation of elastic lamellae with numerous foam cells and fibrosis/calsification, VCMCs proliferation, medial lipid infiltration; and 3, ulcerated plaque/thrombus [19] with some modification. Determination of the stage taken from the highest score of 9 field of view at ×400 magnification: 0, normal; 1, initiation; 2, proliferation; and 3, complication [5] with some modification. Images were obtained using a light microscope equipped with a digital camera (Leica, Wetzlar, Germany) connected to a PC monitor. Experimental animal that did not show atherosclerosis were excluded from the study.
Those paraffin-embedded tissues were processed by giving immunohistochemical staining (IHC) which follows manufacturer's procedure (Santa Cruz Biotechnology Inc., Santa Cruz, CA, USA). Antibody used in this study was policlonal antibody anti proteasome 20S (C-19, Santa Cruz Biotechnology Inc.). Positive control was taken from paraffin-embedded colon carcinoma with positive IHC for proteasome 20S. Paraffin-embedded tissue of atherosclerosis without antibody was used as negative control.
All slides were observed under light microscopy at ×400 magnification. Two-dimensional images were taken as many as 10 pictures each slide using microscope camera (Leica). Expression of abdominal aortic proteasome was assessed by calculation with ImageJ software for percentage of area and integrated density (staining intensity). The percentage area presented the comparison area containing proteasome with a whole cross section of the aorta, which is assumed to be width expression. Density is often assumed to be a representation of concentration. We have used Photoshop image analysis software to analyze vascular proteasome expression as a comparison.
Blood was collected via cardiac puncture. Serum was obtained by centrifugation of blood at 1,000 ×g for 15 minutes. Serum 20S-proteasome concentration was assessed using kits ELISA (E-EL-R1003, Elabscience Biotechnology Co., Ltd, Wuhan, China).
The statistical software SPSS version 17.0 (SPSS Inc., Chicago, IL, USA) was used to analyze the data. Kruskal-Wallis test was used to compare mean value of percentage area and concentration of serum proteasome. Mann-Whitney test was used as post hoc analysis. Analysis of variance test was used to compare mean value of integrated density and post hoc analysis with least significane difference. The correlation between vascular proteasome expression and serum proteasome expression was made using Spearman test. A P-value of 0.05 was considered statistically significant.
The H&E staining revealed that the blood vessel endothelium in the normal group was integrated, the tunica media had fusiform-shaped smooth muscle cell, and the structure of elastic fibers was clear and integrated (Fig. 1A). The tunica intima of the disease region in the model group thickened significantly. The vascular endothelium was not integrated. The aortic lesion showed accumulation of foam cells in the interlamelar spaces (Fig. 1B) with atherosclerosis score of 1 (Table 1), abundance of collagen fibers, proliferated and disoriented smooth muscle cells, calcification (Fig. 1C) with atherosclerosis score of 2 (Table 1), ulcerated plaque (Fig. 1D) with atherosclerosis score of 3 (Table 1). All the results demonstrated that the different stages of atherosclerosis rat model was succesfully created by vitamin D3 and feeding with a high lipid diet and cholic acid.
In this study, the extension of proteasome percentage area were obtained in all atherosclerosis stages, if they were compared to normal. The highest percentage area was obtained in the stage of progression and the lowest was in the normal group (Table 2, Fig. 2). Nonetheless, statistical tests only got a significant difference among all stages of atherosclerosis with normal, but there was no difference among the percentage of area stages of atherosclerosis significantly.
Assessment result of integrated density study was different with area percentage assessment result. Although with similar rank which are: the highest rank was progression group and the lowest rank was normal group, but significant differences were occurred between the initiation versus progression, progression versus normal and complication versus normal (Table 2, Fig. 2). It shows that although each stage of atherosclerosis proteasome was expressed relatively in the same area, but there was an increasing proteasome concentration at the progression and complication stages. The increasing number of concentrations of the proteasome at this progression stages had significant differences with initiation stages. Whereas, there was just the increasing of width expression area in initiation stage. This experiment show that the results acquired by ImageJ similar with the results of Photoshop (Table 3).
Although changes in the VCMCs in atherosclerosis occurs predominantly at the stage of progression, this study found increased expression of proteasome in VCMCs has begun to occur at the stage of initiation (Fig. 2B).
Compared with normal, serum proteasome expression was higher in initiation, progression, and complication. The increase of proteasome serum expression in progression group and complication group was significantly different with normal group. There were significant differences among atherosclerosis stages, except between initiation and complication (Table 4).
Our data showed significant correlation (P=0.03) between the expression of proteasome serum with the percentage area and integrated density, the correlation coefficient is 0.587 and 0.558, respectively. The increase of serum proteasome expression parallel with the increase of expression of tissue proteasome at every stage of atherosclerosis.
Understanding the pathophysiology and treatment of atherosclerosis in human beings has been limited by the lack of satisfactory animal model. The ideal animal model for atherosclerosis should reflect all aspects of the etiopathogenesis of human atherosclerosis and the typical histological findings of its different stages. Until now, there was no such animal model. The experiment of present study were designed with references to studies [121718] with some modification, in order to establish a new method for generating the atherosclerosis model with different stages in a short experimental period. Here, we developed atherosclerosis model by high lipid diet, cholic acid, and vitamin D3. Cholic acid induced changes in plasma high-density lipoprotein levels [20] and increase of absorption of cholesterol [21]. Vitamin D3 treatment is clearly associated with increased arterial calcification and VCMC proliferation through a vascular endothelial growth factor–mediated pathway [17].
These results demonstrated that the intragastric feeding of 700,000 IU/kg combined with cholic acid and high lipid diet succesfully produce a rat model at different stages of atherosclerosis with 86%–100% success rate. Based on the results, groups 2, 3, and 4 were considered as different stages of atherosclerosis, that are initiation, progression, and complication.
In this study, the highest expression of tissue proteasome in atherosclerosis was in progression stage, and the lowest was in initiation stage. The increasing expression of either width or density or both would be resulted in an increasing of enzyme activity of the proteasome, unless there are certain conditions which make enzyme activity not optimal. As an increase of proteasome expression in tissue, it also occurs in serum.
Proteasome plays a role in various biological processes such as inflammation, proliferation, and apoptosis. The processes are an important part in the initiation stage, progression stage and complication stage of atherosclerosis [8]. Mostly, by arranging nuclear factor κB (NF-κB) which further regulates the expression of various genes. In the other side, the proteasome also protects cells by destroying damaged proteins (protein quality control). In fact, it is kind of dualism, where proteasome role is related to oxidative stress. Low oxidative stress will increase the activity of the proteasome, whereas high levels of oxidative stress will inhibit proteasome activity [7].
At the initiation stage of atherosclerosis, endothelial dysfunction and endothelial activation were occured, due to the increasing stress of oxidative and inflammation. Endothelial dysfunction associated with impaired vasorelaxation due to lack of availability of NO•. Endothelial activation is associated with the increasing of expression of adhesion molecules and NF-κB [7]. The increasing activity of proteasome resulted in reducting of endothelial nitric oxide synthase (eNOS) activity and increasing activity of NF-κB, but on the other hand, there may also a protective effect to destroy damaged proteins. The increasing of proteasome expression in the initiation stage of this study is similar with the study of Tan et al. [22] who obtain an increasing activity of proteasome in the early stages of atherosclerosis, so there are possibility that it is developed as a treatment or preventive targets.
This study found increased expression of the proteasome in VCMCs has begun to occur at the stage of initiation. These results are similar with a study by Herrmann et al. [23] obtained on extention of ubiquitinated substrates in the coronary artery, primarily in media of VCMCs in early atherogenesis. Ubiquitin is a marker protein for proteasome substrates. Increased ubiquitin occur because increased proteasome substrate exceeds the ability of the proteasome degradation [23].
This study obtained the expression of the tissue proteasome at the stage of progression increased significantly compared to the initiation stage. Progression stage of atherosclerosis is characterized by the formation of the layer of foam cells and VCMCs proliferation. Proteasome may has contribute significantly to foam cell formation. This is based on the research that aggregated low-density lipoprotein (LDL) that induce ubiquitin conjugating enzymes E2-25K in human monocytes. Expression of this enzyme leads to the increase of ubiquitination and the subsequent degradation of proapoptotic protein (p53) [24]. The same effect also occurs due to oxidized LDL (oxLDL), although high concentrations of oxLDL can inhibit the proteasome [25]. Proteasome also plays a role in the differentiation of monocytes into foam cells, it is through NF-κB. that regulate gene expression of macrophage colony stimulating factor, resulting in the differentiation of monocytes into foam cells. In addition, NF-κB also plays a role to regulate the expression of other genes that play a role in the transformation [26].
Proliferation of smooth muscle cell occurs due to the increased degradation of p21 resulting in increased cyclin cyclin-dependent kinase activity. Proteasome carried out degradation of p21. In addition, the proteasome also plays a role in the transformation of VCMCs from a contractile into a metabolic phenotype through improvement of myocardin degradation by proteasome [7]. Metabolic type smooth muscle cells secrete extracellular matrix proteins such as collagen interstitial. This process resulted in transforming lipid-rich plaque to fibrosis and eventually become calcified plaque which cause stenosis. Proteasome also plays a role in the migration of VCMCs of the tunica media to the intima which is mediated by NF-κB. These transcription factors increase the expression of matrix metalloproteinase genes which play a role in degradation of extracellular matrix [26].
Tissue proteasome expression in complication stage was decrease if compared to progression stage, even though this decrease was not statistically significant. Decreased proteasome activity is likely due to prolonged oxidative stress and subsequent inhibit activation of NF-κB activation by inhibiting the degradation of IκB [27]. Van Herck et al. [28] showed that giving Bortezomib (proteasome inhibitor) for 4 weeks to mice leads to rupture of atherosclerotic plaque. But, Marfella et al. [29] found proteasome activity in atherosclerotic lesions was higher in symptomatic than asymptomatic patient. Such improvements are on the plaque, macrophages and monocytes [29]. The difference in the results of the research on the stage of atherosclerotic complication is likely because the proteasome has a dualism role. Disturbance of cell viability was associated with the decreased activity of the proteasome which leads to the accumulation of oxidative stress that further cause apoptosis. On the other hand, the increase of proteasome activity in plaque inflammation not only causes plaque but also maintain cell viability. But, the predominat role of the proteasome is in causing apoptosis [7].
There was moderate agreement between immunohistochemical and serum expression. The serum level of proteasome does not always reflect its expression and production in tissue, and there are several factors affecting of serum level. Various stimulus that activate T cell is known taking a role in improving circulation of proteasome [30]. Immunity cell such as thrombocyte, dendritic cell, and other antigen presenting cell is also potential as a source of serum proteasome [31].
Differences of proteasome expression between initiation stage and progression stage of the atherosclerotic lesion open an opportunity to do therapy by inhibiting/reducing the activity of the proteasome. Moreover, it also open opportunities for using the proteasome as a marker of atherosclerosis stage, by considering that the difference proteasome expression can also be measured in circulation. Eventhough, it needs further research to consider dualism role of proteasome related to stress oxidative.
In conclusion, increased expression of tissue proteasome occurs at all stages of atherosclerosis. Tissue proteasome expression in progression stage is significantly higher than in initiation stage, but there was no significant difference between progression stage and complication stage. The difference of serum proteasom expression at atherosclerosis stage occurs in all groups, except initiation and complication.
Figures and Tables
Fig. 1
Microscopic aorta abdominal of rats (H&E staining, ×400). (A) Normal group. (B) Initial stage. (C) Progression stage. (D) Complication stage. 1, vascular tunica intima; 2, vascular tunica media ; 3, vascular tunica externa; 4, foam cells in interlamelar spaces; 5, abundence of collagen fibers; 6, calcification; 7, ulcerated plaque. Scale bars=50 µm.
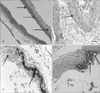
Fig. 2
Immunoreactivity of proteasome in abdominal aorta from rats: normal (A), initiation stage (B), progression stage (C), and complication stage (D). Diaminobenzidine stains the proteasome brown. Slides were counterstained with H&E. Scale bars=50 µm.
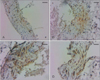
Table 1
Distribution of aortic atherosclerosis in 4 groups
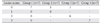
Lesion scores | Group 1 (n=7) | Group 2 (n=7) | Group 3 (n=7) | Group 4 (n=7) |
---|---|---|---|---|
0 | 7 | 1 | 1 | - |
1 | 0 | 6 | - | - |
2 | 0 | - | 6 | - |
3 | 0 | - | - | 7 |
Table 2
Tissue proteasome expression at different stages of atherosclerosis (by ImageJ)

Table 3
Tissue proteasome expression at different stages of atherosclerosis (by Photoshop)

Acknowledgements
This research was partially sponsored by Hibah Doktor scheme of Directorate General of Higher Education, Ministry of Education and Culture, Republic of Indonesia with grant number (343/UN.19.2/PL/2014).
References
1. World Health Organization. Cardiovascular diseases fact sheet [Internet]. Geneva: World Health Organization;2009. cited 2009 Aug 17. Available from: http://www.who.int/mediacentre/factsheets/fs317/en/print.html.
2. Lloyd-Jones D, Adams R, Carnethon M, De Simone G, Ferguson TB, Flegal K, Ford E, Furie K, Go A, Greenlund K, Haase N, Hailpern S, Ho M, Howard V, Kissela B, Kittner S, Lackland D, Lisabeth L, Marelli A, McDermott M, Meigs J, Mozaffarian D, Nichol G, O'Donnell C, Roger V, Rosamond W, Sacco R, Sorlie P, Stafford R, Steinberger J, Thom T, Wasserthiel-Smoller S, Wong N, Wylie-Rosett J, Hong Y. American Heart Association Statistics Committee and Stroke Statistics Subcommittee. Heart disease and stroke statistics: 2009 update: a report from the American Heart Association Statistics Committee and Stroke Statistics Subcommittee. Circulation. 2009; 119:e21–e181.
3. Pyle AL, Young PP. Atheromas feel the pressure: biomechanical stress and atherosclerosis. Am J Pathol. 2010; 177:4–9.
4. Stocker R, Keaney JF Jr. Role of oxidative modifications in atherosclerosis. Physiol Rev. 2004; 84:1381–1478.
5. Stary HC, Chandler AB, Dinsmore RE, Fuster V, Glagov S, Insull W Jr, Rosenfeld ME, Schwartz CJ, Wagner WD, Wissler RW. A definition of advanced types of atherosclerotic lesions and a histological classification of atherosclerosis. A report from the Committee on Vascular Lesions of the Council on Arteriosclerosis, American Heart Association. Circulation. 1995; 92:1355–1374.
6. Fonarow GC. Aggressive treatment of atherosclerosis: the time is now. Cleve Clin J Med. 2003; 70:431–434. 437–438. 440
7. Herrmann J, Lerman LO, Lerman A. On to the road to degradation: atherosclerosis and the proteasome. Cardiovasc Res. 2010; 85:291–302.
8. Herrmann J, Ciechanover A, Lerman LO, Lerman A. The ubiquitin-proteasome system in cardiovascular diseases: a hypothesis extended. Cardiovasc Res. 2004; 61:11–21.
9. Sixt SU, Beiderlinden M, Jennissen HP, Peters J. Extracellular proteasome in the human alveolar space: a new housekeeping enzyme? Am J Physiol Lung Cell Mol Physiol. 2007; 292:L1280–L1288.
10. Marfella R, D'Amico M, Di Filippo C, Siniscalchi M, Sasso FC, Ferraraccio F, Rossi F, Paolisso G. The possible role of the ubiquitin proteasome system in the development of atherosclerosis in diabetes. Cardiovasc Diabetol. 2007; 6:35.
11. Russell JC, Proctor SD. Small animal models of cardiovascular disease: tools for the study of the roles of metabolic syndrome, dyslipidemia, and atherosclerosis. Cardiovasc Pathol. 2006; 15:318–330.
12. Li J, Chen CX, Shen YH. Effects of total glucosides from paeony (Paeonia lactiflora Pall) roots on experimental atherosclerosis in rats. J Ethnopharmacol. 2011; 135:469–475.
13. Vinitha R, Thangaraju M, Sachdanandam P. Effect of tamoxifen on lipids and lipid metabolising marker enzymes in experimental atherosclerosis in Wistar rats. Mol Cell Biochem. 1997; 168:13–19.
14. Dhanya SP, Hema CG. Small animal models of atherosclerosis. Calicut Med J. 2008; 6:e4.
15. Davis HR Jr, Lowe RS, Neff DR. Effects of ezetimibe on atherosclerosis in preclinical models. Atherosclerosis. 2011; 215:266–278.
16. Wilck N, Ludwig A. Targeting the ubiquitin-proteasome system in atherosclerosis: status quo, challenges, and perspectives. Antioxid Redox Signal. 2014; 21:2344–2363.
17. Pang J, Xu Q, Xu X, Yin H, Xu R, Guo S, Hao W, Wang L, Chen C, Cao JM. Hexarelin suppresses high lipid diet and vitamin D3-induced atherosclerosis in the rat. Peptides. 2010; 31:630–638.
18. Murwani S, Ali M, Muliartha K. Diet aterogenik pada tikus putih (Rattus novergicus strain Wistar) sebagai model hewan aterosklerosis. J Kedokt Brawijaya. 2006; 22:6–12.
19. Bennani-Kabchi N, Kehel L, El Bouayadi F, Fdhil H, Amarti A, Saidi A, Marquie G. New model of atherosclerosis in insulin resistant sand rats: hypercholesterolemia combined with D2 vitamin. Atherosclerosis. 2000; 150:55–61.
20. Srivastava RA, Srivastava N, Averna M. Dietary cholic acid lowers plasma levels of mouse and human apolipoprotein A-I primarily via a transcriptional mechanism. Eur J Biochem. 2000; 267:4272–4280.
21. Abo El-Khair DM, El-Safti Fel N, Nooh HZ, El-Mehi AE. A comparative study on the effect of high cholesterol diet on the hippocampal CA1 area of adult and aged rats. Anat Cell Biol. 2014; 47:117–126.
22. Tan C, Li Y, Tan X, Pan H, Huang W. Inhibition of the ubiquitinproteasome system: a new avenue for atherosclerosis. Clin Chem Lab Med. 2006; 44:1218–1225.
23. Hermann J, Gulati R, Napoli C, Woodrum JE, Lerman LO, Rodriguez-Porcel M, Sica V, Simari RD, Ciechanover A, Lerman A. Oxidative stress-related increase in ubiquitination in early coronary atherogenesis. FASEB J. 2003; 17:1730–1732.
24. Kikuchi J, Furukawa Y, Kubo N, Tokura A, Hayashi N, Nakamura M, Matsuda M, Sakurabayashi I. Induction of ubiquitin-conjugating enzyme by aggregated low density lipoprotein in human macrophages and its implications for atherosclerosis. Arterioscler Thromb Vasc Biol. 2000; 20:128–134.
25. Vieira O, Escargueil-Blanc I, Jürgens G, Borner C, Almeida L, Salvayre R, Nègre-Salvayre A. Oxidized LDLs alter the activity of the ubiquitin-proteasome pathway: potential role in oxidized LDL-induced apoptosis. FASEB J. 2000; 14:532–542.
26. Dąbek J, Kułach A, Gąsior Z. Nuclear factor kappa-light-chain-enhancer of activated B cells (NF-kappaB): a new potential therapeutic target in atherosclerosis? Pharmacol Rep. 2010; 62:778–783.
27. Wu M, Bian Q, Liu Y, Fernandes AF, Taylor A, Pereira P, Shang F. Sustained oxidative stress inhibits NF-kappaB activation partially via inactivating the proteasome. Free Radic Biol Med. 2009; 46:62–69.
28. Van Herck JL, De Meyer GR, Martinet W, Bult H, Vrints CJ, Herman AG. Proteasome inhibitor bortezomib promotes a rupture-prone plaque phenotype in ApoE-deficient mice. Basic Res Cardiol. 2010; 105:39–50.
29. Marfella R, D'Amico M, Di Filippo C, Baldi A, Siniscalchi M, Sasso FC, Portoghese M, Carbonara O, Crescenzi B, Sangiuolo P, Nicoletti GF, Rossiello R, Ferraraccio F, Cacciapuoti F, Verza M, Coppola L, Rossi F, Paolisso G. Increased activity of the ubiquitin-proteasome system in patients with symptomatic carotid disease is associated with enhanced inflammation and may destabilize the atherosclerotic plaque: effects of rosiglitazone treatment. J Am Coll Cardiol. 2006; 47:2444–2455.
30. Bochmann I, Ebstein F, Lehmann A, Wohlschlaeger J, Sixt SU, Kloetzel PM, Dahlmann B. T lymphocytes export proteasomes by way of microparticles: a possible mechanism for generation of extracellular proteasomes. J Cell Mol Med. 2014; 18:59–68.
31. Bellavista E, Santoro A, Galimberti D, Comi C, Luciani F, Mishto M. Current understanding on the role of standard and immunoproteasomes in inflammatory/immunological pathways of multiple sclerosis. Autoimmune Dis. 2014; 2014:739705.