Abstract
Macrophages play an important role in aging-related muscle atrophy (i.e., sarcopenia). We examined macrophage density in six striated muscles (cricopharyngeus muscle, posterior cricoarytenoideus muscle, genioglossus muscle, masseter muscle, infraspinatus muscle, and external anal sphincter). We examined 14 donated male cadavers and utilized CD68 immunohistochemistry to clarify macrophage density in muscles. The numbers of macrophages per striated muscle fiber in the larynx and pharynx (0.34 and 0.31) were 5–6 times greater than those in the tongue, shoulder, and anus (0.05–0.07) with high statistical significance. Thick muscle fibers over 80 µm in diameter were seen in the pharynx, larynx, and anal sphincter of two limited specimens. Conversely, in the other sites or specimens, muscle fibers were thinner than 50 µm. We did not find any multinuclear muscle cells suggestive of regeneration. At the beginning of the study, we suspected that mucosal macrophages might have invaded into the muscle layer of the larynx and pharynx, but we found no evidence of inflammation in the mucosa. Likewise, the internal anal sphincter (a smooth muscle layer near the mucosa) usually contained fewer macrophages than the external sphincter. The present result suggest that, in elderly men, thinning and death of striated muscle fibers occur more frequently in the larynx and pharynx than in other parts of the body.
Aging in humans is accompanied by loss of striated muscle mass, strength and function with excitation-contraction uncoupling, a process known as sarcopenia [12]. Although not necessarily an example of sarcopenia, Cruse et al. [3] first demonstrated degeneration of the pharyngeal muscle in specimens obtained by cricopharyngeal myotomy from elderly individuals. However, their description was not based on degenerating muscle fibers themselves but on regenerating muscle fibers with multiple nuclei. Direct demonstration of pharyngeal muscle fiber degeneration appears to be limited to Mu et al. [4], who demonstrated pharyngeal muscle pathology (reduction of fiber thickness and density, accompanying a change in myosin isoforms) using specimens from eight patients with Parkinson disease. It is well known that muscle fiber degeneration is closely related to macrophage invasion from vessels in Duchenne muscular dystrophy [567]. Studies of experimental rotator cuff tears have also yielded similar results [8910].
Sarcopenia or fatty degeneration after atrophy of striated muscle is accompanied by marked accumulation of macrophages [9111213]. T lymphocytes are not required for the development of fatty degeneration [10]. In the present study, we examined the density of macrophages in striated muscles obtained from donated cadavers of elderly individuals without evident neurological diseases or macroscopic pathology in the rotator cuff. For the present analysis, we chose the cricopharyngeus muscle, posterior cricoarytenoideus muscle, genioglossus muscle, masseter muscle, infraspinatus muscle, and external anal sphincter. The cricopharyngeus muscle (a part of the inferior constrictor) is a striated muscle sphincter situated at the pharyngoesophageal junction. The muscle has been considered a major tone generator of the upper esophageal sphincter [1415]. The main function of this muscle is to control flow between the pharynx and esophagus [16]. In laryngeal muscles, the posterior cricoarytenoideus muscle is the strong and limited opener of the rima glottidis especially for forced respiration. The infraspinatus and genioglossus muscles are strongest of the rotator cuff muscles and external lingual muscles, respectively. The external anal sphincter, a striated muscle surrounding the lower end of the alimentary canal, has been one of our major focuses of anatomical study [1718] and we are familiar with the necessary dissection procedures. Consequently, to provide better understanding of sarcopenia, the aim of this study was to compare macrophage density in striated muscles with quite different functions.
The study was performed in accordance with the provisions of the Declaration of Helsinki 1995 (as revised in Edinburgh 2000). We examined 14 donated male cadavers ranging in age from 72 to 93 years, with a mean age of 83 years. The cause of death had been ischemic heart failure. These cadavers had been donated to Tokyo Dental College for research and education on human anatomy, and their use for research was approved by the university ethics committee. The donated cadavers had been fixed by arterial perfusion with 10% v/v formalin solution and stored in 50% v/v ethanol solution for more than 3 months. From the left or right side of each cadaver, we obtained six striated muscles: (1) the cricopharyngeus muscle, (2) the posterior cricoarytenoideus muscle, (3) the genioglossus muscle, (4) the masseter muscle, (5) the infraspinatus muscle, and (6) the external anal sphincter muscle. Muscles Nos. 1 and 2 were found at the level of the cricothyroid joint (Fig. 1), muscle No. 3 near the origin from the mandible, muscles Nos. 4 and 5 near the nerve entry, and muscle No. 6 on the lateral side of the thickest part of the internal anal sphincter. After routine procedures for paraffin embedded histology, we examined the cross-section histology (4 µm in thickness) of the striated muscle fibers.
Parts of the present histological sections of the lower pharynx and larynx including the cricoid cartilage (Fig. 1) had been prepared for our recent studies of the cricothyroid joint [1920]. For this preparation, the specimens were decalcified by incubating them (1) at room temperature using Plank-Rychlo solution (AlCl2/6H2O, 7.0 w/v%; HCl, 3.6; HCOOH, 4.6) for 2–4 days (specimens from 15 cadavers) or (2) at 4℃ in 0.5 mol/l EDTA solution (pH 7.5; decalcifying solution B, Wako, Tokyo, Japan) for 7–14 days (specimens from 15 cadavers).
We prepared 2–3 adjacent sections for hematoxylin and eosin or elastica Masson staining as well as for immunohistochemistry for macrophages. The primary antibody used was mouse monoclonal anti-human CD68 KP1 (1:100, Dako M0814, Glostrup, Denmark). The secondary antibody (Dako Chem Mate Envison Kit, Dako) was labeled with horseradish peroxidase (HRP), and antigen-antibody reactions were detected by the HRP-catalyzed reaction with diaminobenzidine. Counterstaining with hematoxylin was performed on the same samples. A negative control without the first antibody was set up for each of the specimens. Osteoclasts in the bony cricoid were used as a positive control for the immunostaining. Observations and photography were usually performed with a Nikon Eclipse 80 (Nikon, Tokyo, Japan). On photographs including a hot spot (an area with the highest density of target cells) [21] taken using a ×20 objective (providing a field measuring 0.6×0.45 mm), we manually counted CD68-positive macrophages. We also counted the numbers of muscle fiber cross-sections and measured the muscle fiber diameter in the same photo. To assess statistical significance, we used the Tukey's test.
Striated muscle fibers were consistently packed densely in the shoulder and tongue, but often loosely in the pharynx, larynx, and anal sphincter. Muscle fibers were usually thinner than 50 µm in all six muscles examined. Conversely, thick muscle fibers over 80 µm in diameter were seen in the cricopharyngeal muscle and posterior cricoarytenoid muscles of limited two specimens (from men aged 87 and 91 years) as well as in the external anal sphincter of one specimen (from a man aged 91 years; the same as above). We did not find any multinuclear muscle cells suggestive of regeneration at any of the sites examined. Likewise, we did not find any pathologic features in peripheral nerves such as onion-like peeling of the nerve sheath. Depending on the amount of loose connective tissue between muscle fiber bundles, there was considerable individual variation in the numbers of muscle fibers included in the visual field at an objective magnification of ×20: 62–182 muscle fibers for the cricopharyngeus muscle, 94–208 for the posterior cricoarytenoid, 155–319 for the genioglossus, 100–267 for the masseter, 212–290 for the infraspinatus, and 171–554 for the external anal sphincter.
CD68-positive macrophages were usually small than 10 µm at the maximum diameter, but larger macrophages were sometimes admixed in the cricopharyngeus, posterior cricoarytenoideus, and external anal sphincter muscles (Fig. 2). These larger macrophages were accumulated along the periosteum of the bony cricoid cartilage to which the posterior cricoarytenoideus was attached. The numbers of macrophages per visual field varied between specimens because most macrophages were located in the loose connective tissues rather than adjacent to individual muscle fibers. Therefore, we expressed the number of macrophages as that for each single striated muscle fiber (Table 1). Notably, the number of macrophages per muscle fiber in the larynx and pharynx (0.34 and 0.31) were 5–6 times greater than in the tongue, shoulder and anus (0.07–0.05) with high statistical significance (P<0.01). In the cricopharyngeus and posterior cricoarytenoideus muscle (more than 4-fold) this parameter varied significantly between individuals, in contrast to only a small difference (less than 2-fold) in the other muscles.
No clear age-dependent difference was identified at any of the muscles examined, since the ages of the cadavers examined ranged from 72 to 93 years. At the beginning of this study, we suspected that mucosal macrophages would have invaded into the muscle layer of the larynx and pharynx, but we found no evidence of inflammation in the mucosa. Likewise, the internal anal sphincter (a smooth muscle layer between the mucosa and external anal sphincter) usually contained fewer macrophages than the external sphincter (data not shown).
Most of the morphological features that allow identification of muscle degeneration seem difficult to evaluate quantitatively. Cook et al. [22] described increased incidences of ragged red fibers, nemaline bodies and segmental necrosis with macrophage myophagia. However, in cadaveric studies, we suspected that the appearance of ragged red fibers and nemaline bodies would likely depend on postmortem changes after death. Because only two cadavers had thick muscle fibers, most of the present muscle specimens appeared to exhibit thinning due to muscle degeneration. However, to our knowledge, no information about muscle fiber thickness in young adults is available for comparison. Likewise, any difference in muscle fiber density between individuals or between sites was also difficult to evaluate. Nevertheless, the specifically high macrophage density per muscle fiber suggested that, in elderly men, cell death occurs more frequently in the larynx and pharynx than in other parts of the body. It has been suggested that high macrophage density results from ultrastructural damage to muscle fibers [11]. Macrophage migration inhibitory factor (MIF) is a proinflammatory cytokine secreted by activated T cells and macrophages that has antiapoptotic, proproliferative, and chemotactic effects. According to the study done by Reimann et al. [23], MIF is a skeletal muscle cytokine with probable functions beyond inflammatory pathology in the complex regenerative response to muscle fiber damage. Another explanation might be a possible difference in muscle vascular density, since macrophages are likely to migrate along vessels: a higher vascular density might accelerate migration and accumulation of macrophages. Indeed, laryngeal and pharyngeal muscles tend to show higher density of intramuscular vessels than the shoulder muscles (unpublished data). However, according to the present observations, macrophages were not accumulated along vessels but scattered in the muscle.
According to Sivarao and Goyal [15], pharyngeal muscles are tonically active, have a high degree of elasticity, do not develop maximal tension, and are composed of a mixture of slow- and fast-twitch fibers, with the former predominating. These features enable the striated muscles to maintain a resting tone while remaining able to stretch open under forces such as a swallowed food bolus. This passive action due to a bolus seems to be quite different from a muscle action against resistance. Likewise, a maintaining of the tonus as a muscle mass seems to be important in functions of the external anal sphincter. The posterior cricoarytenoideus muscle, the strong and limited opener of the rima glottidis, also seems to need the continuous action against the other laryngeal muscles for closure. Conversely, the genioglossus, masseter and infraspinatus muscles seem to be able to react against increasing resistance although, to our regret, experimental data from the resistance exercise may be absent for these muscles. Therefore, a continuous or tonic action mode of the muscle might be a factor connecting with atrophy in the elderly.
The size of the masseter muscle has been used as an indicator of masticatory function, especially temporomandibular joint dysfunction [2425]. The masseter muscle is composed of types 2A and 2X fibers and does not contain fatigue-resistant type 1 fibers [26]. Thus, the function of the masseter seems to be rather different from the possible tonic action of the pharyngeal and laryngeal muscles. In fact, the present study revealed a low macrophage density in the masseter muscle, being similar to the infraspinatus muscle of the shoulder. Conversely, as seen in animal experiments [27], immobilization due to temporomandibular joint dysfunction would increase the number of macrophages in the masseter. Overall, the present result suggest that, in elderly men, thinning and death of striated muscle fibers occur more frequently in the larynx and pharynx than in other parts of the body. However, further morphometrical studies of muscles seem to be necessary to strengthen the possible muscle action-dependent difference in sarcopenia.
A major limitation of the present study was that macrophage heterogeneity was not evaluated. In contrast to the usual situation in the musculoskeletal system, larger macrophages were seen in the pharynx, larynx, and anus. In a study of Duchenne muscular dystrophy, Desguerre et al. [6] have reported an increase of CD206-positive activated macrophages and a relative decrease of CD56-positive satellite cells. However, these markers were not available in the present cadaveric specimens (unpublished data).
Muscle aging was associated with elevations of anti-inflamatory M2a macrophages that can increase muscle fibrosis [28]. These macrophages are activated to the M2 phenotype by IL-10 [2930]. Moreover, Wang et al. [31] calimed that muscles of old mice receiving young bone marrow cells showed fewer M2a macrophages. Thus, the age-related increase in M2a macrophages in aging muscle and the associated muscle fibrosis are determined in part by the age of bone marrow cells. On the other hands, according to Siriett et al. [12], antagonism of myostatin led to satellite cell activation, increased Pax7 and MyoD protein levels, and greater myoblast and macrophage cell migration, resulting in enhanced muscle regeneration after notexin injury in aged mice. The results from this case indicates that the antagonism of myostatin has significant therapeutic potential in the alleviation of sarcopenia. In present study, the number of macrophages per striated muscle fiber in larynx and pharynx were 5–6 times greater than those in the tongue, shoulder, and anus. Therefoer, there is a possibility that macrophage cell migration inhibits muscle aging in larynx and pharynx.
Figures and Tables
Fig. 1
Histological section for assessment of the pharyngeal and laryngeal striated muscles. A 72-year-old man. Cross-sections (elastica Masson staining) of the striated muscles are easily found in tilted horizontal sections including the cricothyroid joint (CT joint). CC, cricoid cartilage; CPM, cricopharyngeal muscle; ICTC, inferior cornu of the thyroid cartilage; PCAM, posterior cricoarytenoid muscle; TYG, thyroid gland. Scale bar=5 mm.
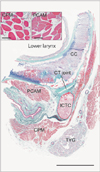
Fig. 2
Macrophages in cross-sections of striated muscles. All panels are prepared at the same magnification (scale bar in panel B=0.1 mm). Each panel corresponds to almost half the area of the visual field employing a ×20 objective for counting of macrophages. Arrows and arrowheads indicate CD68-positive macrophages: some macrophages (arrowheads) are large and appear to be active. Pharynx, the cricopharyngeal muscle (A); larynx, the posterior cricoarytenoid muscle (B); tongue, the genioglossus muscle (C); face, masseter muscle (D); shoulder, the infraspinatus muscle (E); anus, the external anal sphincter muscle (F).
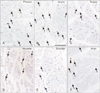
Table 1
Macrophage numbers in a striated muscle per visual field at ×200
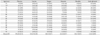
Numbers in parenthesis indicate macrophage numbers per 1 muscle fiber. The pharynx or larynx showed a statistically significant difference (P<0.01) from each of the tongue, masseter, shoulder and anal sphincter. Pharynx, cricopharyngeal muscle; Larynx, posterior cricoarytenoid muscle; Tongue, genioglossus muscle; Shoulder, infrasupinatus muscle; Anal sphincter, external anal sphincter muscle. a)Each muscle of the specimen 74 is shown in Fig. 1.
Acknowledgements
We are grateful to the individuals who donated their bodies after death to Tokyo Dental College for research and education on human anatomy without any economic benefit. We also thank their families for agreeing to the donation as well as their patience in waiting for the return of their remains after study.
References
1. Ryan M, Butler-Browne G, Erzen I, Mouly V, Thornell LE, Wernig A, Ohlendieck K. Persistent expression of the alpha1S-dihydropyridine receptor in aged human skeletal muscle: implications for the excitation-contraction uncoupling hypothesis of sarcopenia. Int J Mol Med. 2003; 11:425–434.
2. Pietrangelo T, Puglielli C, Mancinelli R, Beccafico S, Fanò G, Fulle S. Molecular basis of the myogenic profile of aged human skeletal muscle satellite cells during differentiation. Exp Gerontol. 2009; 44:523–531.
3. Cruse JP, Edwards DA, Smith JF, Wyllie JH. The pathology of a cricopharyngeal dysphagia. Histopathology. 1979; 3:223–232.
4. Mu L, Sobotka S, Chen J, Su H, Sanders I, Adler CH, Shill HA, Caviness JN, Samanta JE, Beach TG. Arizona Parkinson's Disease Consortium. Altered pharyngeal muscles in Parkinson disease. J Neuropathol Exp Neurol. 2012; 71:520–530.
5. Yeung D, Kharidia R, Brown SC, Górecki DC. Enhanced expression of the P2X4 receptor in Duchenne muscular dystrophy correlates with macrophage invasion. Neurobiol Dis. 2004; 15:212–220.
6. Desguerre I, Mayer M, Leturcq F, Barbet JP, Gherardi RK, Christov C. Endomysial fibrosis in Duchenne muscular dystrophy: a marker of poor outcome associated with macrophage alternative activation. J Neuropathol Exp Neurol. 2009; 68:762–773.
7. Kobayashi K, Izawa T, Kuwamura M, Yamate J. The distribution and characterization of skeletal muscle lesions in dysferlin-deficient SJL and A/J mice. Exp Toxicol Pathol. 2010; 62:509–517.
8. Gumucio JP, Davis ME, Bradley JR, Stafford PL, Schiffman CJ, Lynch EB, Claflin DR, Bedi A, Mendias CL. Rotator cuff tear reduces muscle fiber specific force production and induces macrophage accumulation and autophagy. J Orthop Res. 2012; 30:1963–1970.
9. Gumucio J, Flood M, Harning J, Phan A, Roche S, Lynch E, Bedi A, Mendias C. T lymphocytes are not required for the development of fatty degeneration after rotator cuff tear. Bone Joint Res. 2014; 3:262–272.
10. Gumucio JP, Korn MA, Saripalli AL, Flood MD, Phan AC, Roche SM, Lynch EB, Claflin DR, Bedi A, Mendias CL. Aging-associated exacerbation in fatty degeneration and infiltration after rotator cuff tear. J Shoulder Elbow Surg. 2014; 23:99–108.
11. Evans WJ. Effects of exercise on senescent muscle. Clin Orthop Relat Res. 2002; 403 Suppl. S211–S220.
12. Siriett V, Salerno MS, Berry C, Nicholas G, Bower R, Kambadur R, Sharma M. Antagonism of myostatin enhances muscle regeneration during sarcopenia. Mol Ther. 2007; 15:1463–1470.
13. Colombo E, Bedogni F, Lorenzetti I, Landsberger N, Previtali SC, Farina C. Autocrine and immune cell-derived BDNF in human skeletal muscle: implications for myogenesis and tissue regeneration. J Pathol. 2013; 231:190–198.
14. Lang IM, Shaker R. An overview of the upper esophageal sphincter. Curr Gastroenterol Rep. 2000; 2:185–190.
15. Sivarao DV, Goyal RK. Functional anatomy and physiology of the upper esophageal sphincter. Am J Med. 2000; 108:Suppl 4a. 27S–37S.
16. Ertekin C, Aydogdu I. Electromyography of human cricopharyngeal muscle of the upper esophageal sphincter. Muscle Nerve. 2002; 26:729–739.
17. Ishiyama G, Hinata N, Kinugasa Y, Murakami G, Fujimiya M. Nerves supplying the internal anal sphincter: an immunohistochemical study using donated elderly cadavers. Surg Radiol Anat. 2014; 36:1033–1042.
18. Kim JH, Kinugasa Y, Yu HC, Murakami G, Abe S, Cho BH. Lack of striated muscle fibers in the longitudinal anal muscle of elderly Japanese: a histological study using cadaveric specimens. Int J Colorectal Dis. 2015; 30:43–49.
19. Kawamoto A, Honkura Y, Suzuki R, Abe H, Abe S, Murakami G, Katori Y. Cricothyroid articulation in elderly Japanese with special reference to morphology of the synovial and capsular tissues. J Voice. 2016; 30:538–548.
20. Serikawa M, Yamamoto M, Kawamoto A, Katori Y, Kinoshita H, Matsunaga S, Abe S. The cricothyroid joint in elderly Japanese individuals. Anat Sci Int. 2016; 91:250–257.
21. Weidner N, Semple JP, Welch WR, Folkman J. Tumor angiogenesis and metastasis: correlation in invasive breast carcinoma. N Engl J Med. 1991; 324:1–8.
22. Cook IJ, Blumbergs P, Cash K, Jamieson GG, Shearman DJ. Structural abnormalities of the cricopharyngeus muscle in patients with pharyngeal (Zenker's) diverticulum. J Gastroenterol Hepatol. 1992; 7:556–562.
23. Reimann J, Schnell S, Schwartz S, Kappes-Horn K, Dodel R, Bacher M. Macrophage migration inhibitory factor in normal human skeletal muscle and inflammatory myopathies. J Neuropathol Exp Neurol. 2010; 69:654–662.
24. Pereira LJ, Gavião MB, Bonjardim LR, Castelo PM, van der Bilt A. Muscle thickness, bite force, and craniofacial dimensions in adolescents with signs and symptoms of temporomandibular dysfunction. Eur J Orthod. 2007; 29:72–78.
25. Ozaki M, Kaneko S, Soma K. Masseter muscular weakness affects temporomandibular synovitis induced by jaw opening in growing rats. Angle Orthod. 2008; 78:819–825.
26. Sano R, Tanaka E, Korfage JA, Langenbach GE, Kawai N, van Eijden TM, Tanne K. Heterogeneity of fiber characteristics in the rat masseter and digastric muscles. J Anat. 2007; 211:464–470.
27. Bar-Shai M, Carmeli E, Coleman R, Rozen N, Perek S, Fuchs D, Reznick AZ. The effect of hindlimb immobilization on acid phosphatase, metalloproteinases and nuclear factor-kappaB in muscles of young and old rats. Mech Ageing Dev. 2005; 126:289–297.
28. Wehling-Henricks M, Jordan MC, Gotoh T, Grody WW, Roos KP, Tidball JG. Arginine metabolism by macrophages promotes cardiac and muscle fibrosis in mdx muscular dystrophy. PLoS One. 2010; 5:e10763.
29. Villalta SA, Rinaldi C, Deng B, Liu G, Fedor B, Tidball JG. Interleukin-10 reduces the pathology of mdx muscular dystrophy by deactivating M1 macrophages and modulating macrophage phenotype. Hum Mol Genet. 2011; 20:790–805.
30. Deng B, Wehling-Henricks M, Villalta SA, Wang Y, Tidball JG. IL-10 triggers changes in macrophage phenotype that promote muscle growth and regeneration. J Immunol. 2012; 189:3669–3680.
31. Wang Y, Wehling-Henricks M, Samengo G, Tidball JG. Increases of M2a macrophages and fibrosis in aging muscle are influenced by bone marrow aging and negatively regulated by muscle-derived nitric oxide. Aging Cell. 2015; 14:678–688.