Abstract
This study was carried out to explore the effect of DNA hypomethylation on chondrocytes phenotype, in particular the effect on chondrocyte hypertrophy, maturation, and apoptosis. Chondrocytes derived from caudal region of day 17 embryonic chick sterna were pretreated with hypomethylating drug 5-aza-2'-deoxycytidine for 48 hours and then maintained in the normal culture medium for up to 14 days. Histological studies showed distinct morphological changes occurred in the pretreated cultures when compared to the control cultures. The pretreated chondrocytes after 7 days in culture became bigger in size and acquired more flattened fibroblastic phenotype as well as a loss of cartilage specific extracellular matrix. Scanning electron microscopy at day 7 showed chondrocytes to have increased in cell volume and at day 14 in culture the extracellular matrix of the pretreated cultures showed regular fibrillar structure heavily embedded with matrix vesicles, which is the characteristic feature of chondrocyte hypertrophy. Transmission electron microscopic studies indicated the terminal fate of the hypertrophic cells in culture. The pretreated chondrocytes grown for 14 days in culture showed two types of cells: dark cells which had condense chromatin in dark patches and dark cytoplasm. The other light chondrocytes appeared to be heavily loaded with endoplasmic reticulum indicative of very active protein and secretory activity; their cytoplasm had large vacuoles and disintegrating cytoplasm. The biosynthetic profile showed that the pretreated cultures were actively synthesizing and secreting type X collagen and alkaline phosphatase as a major biosynthetic product.
Endochondral bone formation is the process by which the long bone continues to grow under the influence of growth factors and hormones [1]. During the embryonic limb development, mesenchyme cells differentiate into chondrocytes. A primary ossification center appears in the middle which is followed by secondary ossification center at both of the extremities [23]. The primary ossification center expands and is soon replaced by bone. The chondrocytes in the growth plate are arranged in morphological distinct states: resting, proliferative, and hypertrophic stages. This reflects the dynamic changes in the morphology and function of these cells. The resting cells are small rounded cells randomly distributed in the cartilage specific extracellular matrix (ECM). These chondrocytes secrete mainly type II and IX collagen, as well as large chondroitin sulphate proteoglycans (aggrecan) which provides osmotic resistance for the cartilage to resist compressive loads [4]. The proliferative zone contains cells that are undergoing rapid mitosis resulting in a stack of cells arranged in longitudinal column. The hypertrophic cells are rounded but their volumes are dramatically increased and start synthesizing type X collagen and alkaline phosphatase which helps in the mineralization process [56]. The hypertrophic stage is considered to be the terminal stage of the chondrocytes which is soon replaced by osteocytes, osteoblasts, and bone marrow cells resulting in the replacement of cartilage with the bone. However the fate of the hypertrophic chondrocytes remains highly controversial. Most of the current data suggested that hypertrophic chondrocytes degenerate, and die by apoptosis and are replaced by osteoblasts during mammalian bone development. However, studies from avian bone development state that the opposite may be true: that hypertrophic chondrocytes may instead join the cohort of newly differentiated osteogenic cells, participating in bone formation [789]. An important step in apoptosis of cells is phagocytosis by macrophages or adjacent cells. In cartilage, this is not possible due to the absence of such specialized phagocytic cells and the presence of chondrocytes within lacunae that are separated from each other by abundant ECM, making it difficult for these cells to be phagocytized by the adjacent cells.
The concept of asymmetrical cell division proposed by Horvitz and Herskowitz [10], states that this type of cell division results in two types of cells with different fates. When the hypertrophic chondrocytes derived from the tibia of a 14-day-old chick embryo were examined by simple microscopic and transmission electron microscopy, as well as different apoptotic markers, they found two distinct cell types of different morphology within one lacuna. One cell was osteogenic (darker cytoplasm), which was viable and actively synthesizing proteins as indicated by many ribosomes and rough endoplasmic reticulum (RER), and the other type of cell remained chondrogenic with pale cytoplasm which appeared to disintegrate.
5-Azacytidine and 5-aza-2'-deoxycytidine are potent demethylation agents and shown to initiate differentiation in a number of cell types [1112]. Pretreatment of mesynchymal stem cells with 5-azacytidine significantly facilities osteogenic differentiation and caused hypomethylating of genome DNA and increased gene expression [13]. Altered DNA methylation in chondrocytes could results a shift in cartilage homeostasis towards degradation thus causing the onset of osteoarthritis [1415]. The purpose of this study was to elucidate the effect of hypomethylation of DNA on chondrocyte phenotype.
About 10 sterna of day 17 chick embryos were dissected and removed from the surrounding tissues. The cephalic and caudal (one third of the tip region) sterna were dissected as described [16]. Tissues were finely diced and preincubated for 30 minutes at 37℃ with Bacterial collagenase type 1A (10 mg/ml, Sigma, Dorset, UK) and trypsin (0.4 mg/ml, GibcoBRL in minimal essential medium, Grand Island, NY, USA). The resulting supernatant containing the perichondria fibroblasts were removed and tissues were further incubated for 60 minutes under the same conditions as above. Released chondrocytes were washed three times with Dulbecco's modified Eagle's medium (DMEM)+10% fetal calf serum (FCS; GibcoBRL). Chondrocytes were cultured in 75-cm2 plastic tissue culture dishes or in multiwall tissue culture plates with the seeding density of 1×105 cells/cm2. All the cultures were incubated at 37℃ in an atmosphere of 95% air and 5% CO2 in DMEM which was buffered with 10 mM HEPES and 0.37% sodium bicarbonate and supplemented with 100 µg/ml ascorbate, 100 units/ml penicillin, 0.1 mg/ml streptomycin, 100 µg/ml glutamine, and 10% FCS.
One hour after plating the cultures, they were treated with 5-aza-2'-deoxycytidine (15 µg/ml in DMEM containing 10% FCS) for 48 hours. The medium was then removed and the cultures were maintained in DMEM+10% FCS which was replaced after every other day throughout the culture period. Control cultures were set up without the addition of 5-aza-2'-deoxycytidine to the culture medium.
Each well was washed twice with phosphate-buffered saline (PBS). The chondrocytes were released by treatment with trypsin (0.4 mg/ml) and bacterial collagenase type IA (10 mg/ml) at 37℃ for 1 hour. After centrifugation at 250 ×g for 3 minutes, the cells were re-suspended in 5 ml of DMEM and counted using a Coulter counter. This procedure was repeated five times per plate.
Cultures were washed three times with PBS. The cells were then fixed in ice-cold methanol for 10 minutes, rinsed in 0.05 M TBS, and then stained with alcian blue (pH 2.5) for 15 minutes. The cells were counterstained with 1% aqueous neutral red and mounted with Loctite adhesive.
The cells were fixed with formal saline (10% formaldehyde in PBS) for 30 minutes, washed twice with PBS and stained with acridine orange as described [17].
Another stain used for staining proteoglycans was toluidine blue. The fixed cells were stained with 0.5% toluidine blue and 0.05 M HCl, pH 1.0 for 15 minutes. all the samples were mounted in water under a cover slip and examined by Leitz Orthoplan Fluorescent microscope (Leica, Deerfield, IL, USA) employing violet illumination and a yellow filter [18].
Cultures were washed three times with PBS and fixed in 1.25% glutaraldehyde in 0.1 M sodium cacodylate buffer (pH 7.3), which had been pre-warmed to 37℃ before use for at least 1 hour. Samples were dehydrated with the descending series of 50% alcohol to absolute alcohol. Using 100% ethanol as the intermediate solvent, the cover slips containing fixed cells were placed in Polaron critical point dryer (CPD) and flushed thoroughly with CO2 for 2 hours to remove all traces of residual ethanol [19]. Following CPD the samples were mounted on aluminum stubs, gold coated, and examined in 301 EM (Philips, Hillsboro, OR, USA).
Cultures were washed three times with PBS and fixed in 1.25% glutaraldehyde in 0.1 M sodium cacodylate buffer (pH 7.3), which had been pre-warmed to 37℃ before use. After 2 hours, cultures were washed several times in the sodium cacodylate buffer with added 0.3 mM CaCl3 and then carefully peeled from the culture dishes, cut into small rectangles (approximately 3.5–4 mm), and transferred to glass vials for processing. The tissue was postfixed in 1% osmium tetroxide in 0.05 M sodium cacodylate buffer (pH 7.3) for 1 hour at 4℃, rinsed in buffer, and dehydrated in an ascending alcohol series. After two 15-minute incubations in propylene oxide, the tissue was infiltrated with a 1:1 mixture of propylene oxide and TAAB epoxy resin (TAAB Laboratories Equipment Ltd., Aldermaston, UK) for 1 hour, and then left overnight in a 1:3 mixture of propylene oxide resin at 4℃ on a rotator. The next day, the tissues were given three 1-hour changes of neat resin at 48℃, placed in flat embedding molds with three or four rectangles overlying each other, and polymerized at 60℃ for 72 hours. Ultra-thin pale gold sections were cut and mounted on copper grids and contrasted with uranyl acetate and lead citrate. Sections were examined by an electron microscope EM 301 (Philips) at an accelerating voltage of 60 kV.
Chondrocytes cultured for 14 days were used to localize type X collagen in culture. Control and pretreated sternal caudal region chondrocytes (10×106) were fixed with formal saline (10% formaldehyde in PBS) for 30 minutes, and washed twice with PBS. The cultures were then incubated with a polyclonal antibody known to recognize the helical domain of type X collagen for 1 hour. Bound antibody was reacted with a second antibody IgG-linked to alkaline phosphatase for 60 to 90 minutes and subsequent color reaction at the site of type X collagen in the culture was identified by red staining.
Medium proteins from control and treated chondrocyte cultures at day 7 and day 14 were recovered by 30% ammonium sulphate precipitation. The precipitate was dialyzed extensively against PBS buffer and mixed with 4× sample buffer, boiled (5 minutes), then run on 8% sodium dodecyl sulfate polyacrylamide gel electrophoresis and transferred to poly(vinylidene difluoride) membrane. Membranes were blocked (30 minutes) in 5% dried milk, then treated with primary antibody (1 hour), washed 3 times (PBS/0.05% Tween 20), and incubated (1 hour) with secondary horseradish peroxidase-conjugated antibody. Sign insert citation al was developed with enhanced chemiluminescence and visualized by autoradiography.
Alkaline phosphatase was determined by a modification of the method of Lowry [20]. 10×106 Chondrocytes were washed with PBS three times. Five hundred microliters of ice cold extraction buffer which contains 0.01 M Tris-HCl was added to the petri dishes and the cells were lysed by freezing in a liquid ethanol-dry-ice bath and thawed at 37℃ for three times. The cell lysate was centrifuged at 10.000 ×g for 20 minutes at 4℃ and cell pellet used to estimate the DNA content while supernatant were removed for alkaline phosphatase activity. Alkaline phosphatase activity was assayed by measuring the release of p-nitrophenol from p-nitrophenylphosphate at 37℃. The assay mixture (100 µl) contains 0.2 M diethanolamine-HCl (pH 9.8), 1 mM MgCl2 and 1 mg/ml p-nitrophenylphsphate.
The chondrocytes were treated with varying doses of 5-aza-2'-deoxycytidine for 48 hours and then maintained in the 5-aza-2'-deoxycytidine depleted culture conditions for up to 14 days. The effect of 5-aza-2'-deoxycytidine on cell number was assessed after 72 hours, 7 days, and 14 days in a culture by brief trypsinization and collagenase digestion of the cultured cells grown on multiwall culture plates in triplicates followed by counting on automated coulter counter. A dose dependent cytotoxic effect of 5-aza-2'-deoxycytidine was observed on chondrocytes in culture. Percentage cell viability was calculated with respect to the control cultures grown at the same conditions at same time without any treatment with 5-aza-2'-deoxycytidine. Initially after 72 hours there was a loss of 29.5% cell viability at 15 µg/ml as compared to the control cultures. However, by day 7 the pretreated culture showed 75% viability as compared to the control cultures which actually reached to 90% recovery from 15 µg/ml treatment at day 14 in culture.
The effect of treatment with 5-aza-2'-deoxycytidine at 15 µg/ml on cell morphology of caudal region chondrocytes was assessed. This concentration of the cytosine analogue had been shown in preliminary studies to produce an optimal dose response with respect to changes in gene expression in the chondrocytes. Preliminary experiments also indicated that not only dose but the time and duration of treatments within the cell cycle were also crucial. When the cells were treated after one week of being fully confluence, they were completely refractive to treatment. Exposure to the treatment proved most effective one or two hours after plating, before they actually adhere to the surface and start to proliferate. Once the cells were settled and committed to the chondrocytes morphology, treatment with the hypomethylating drug was ineffective.
When caudal region chondrocytes were treated with 5-aza-2'-deoxycytidine, they exhibited changes in morphology. From day 3 onward, treated cells were larger in size than the untreated cultures. To quantify the relative increase in cell volume during the culture period, cells were released from ECM by brief trypsinization and collagenase treatment. They were viewed under phase contrast microscope on hemocytometer. The diameter of both treated and untreated cells were measured directly from photograph taken at regular time points (Fig. 1).
The control and 5-aza-2'-deoxycytidine treated cultures were stained with acridine orange, which showed that upon treatment, most of the cells lost their rounded morphology and became flattened with long cell processes projecting radially. Many multinucleated cells were also observed in the pretreated cultures (Fig. 2A, B). The chondrocytes without any treatment remain rounded and grew in colonies forming a cartilage nodule. In addition, the pretreated cells appeared to have increased cell size and extensively vacuolated, a feature thought to be a characteristic of hypertrophic chondrocytes [2122].
Chondrocytes cultured to day 7 were also stained with alien blue. The control cultures were stained extensively with this dye, providing evidence that the ECM secreted by these cells was rich in sulphate proteoglycans. The pretreated cultures, however, showed negative staining indicating the absence of cartilage-specific proteoglycans (Fig. 2C, D).
Toluidine blue (cationic dye that stains a variety of proteoglycans) was also used to stain the control and pretreated chondrocytes at day 7 in cultures (Fig. 2E, F). Two cell populations were identified in the treated cultures on the basis of their staining abilities. There were chondrocytes cells which stained with toluidine blue and fibroblastic cells which showed negative staining. This was in contrast to control cultures, which showed a homogeneous population of chondrocytes with toluidine positive metachromatic matrix.
Scanning electron microscopic studies provided further insight into the details of various aspects of cellular morphology including the extracellular fibrillar assembly. The control and pretreated cultures were compared at day 7 in culture with respect to their cell shape and size. The diameter of the control and pretreated cells were remarkably different. The cell volume increased to 2-fold in the treated samples (Fig. 3A, B). A comparison of the fibrillar assembly of control and pretreated cells at day 14 in culture showed the arrangement of the fibrillar structure to be similar in both cases, but the most striking feature of the treated cultures were the presence of electron dense particles about 80 to 100 nm in length which were extensively distributed in the ECM (Fig. 3C, D). This electron dense material was found to be associated with fibrillar structure and represented matrix vesicles since the length of these particles is same as that of matrix vesicles in other electron microscope studies [23]. When the same cells were analyzed for enzyme alkaline phosphatase activity, it was found to be greatly enhanced (Fig. 4). Matrix vesicles are thought to be involved actively in extracellular remodeling and preparing it for subsequent mineralization [2425].
For transmission electron microscopic (TEM) studies control and pretreated chondrocytes cultures grown to day 14 were fixed in cacodylate buffer to preserve their cell shape and morphology. In the control cultures, all the cells looked healthy and viable (Fig. 5A, C), there were no signs of nuclear condensation and the cytoplasm looked healthy with vacuoles and other cytoplasmic organelles such as mitochondria and storage vesicles.
The pretreated cultures however (Fig. 5B, D) clearly showed two types of chondrocytes. One with condensed nuclei packed with electron dense materials with comparatively dark cytoplasm, the type of cells which had been described as dark chondrocytes in a number of other studies [782627]. The fact that they were loaded with a number of ribosomes and extensive network of RER indicated their enhanced biosynthetic activity. The other types of chondrocytes observed in TEM were with disintegrating nucleus, with the presence of a number of large vacuoles which is considered to be the marker of hypertrophic chondrocytes. Their cytoplasm was packed with RER indicating a highly active protein synthesis in these types of cells. Another striking feature of these pretreated cells was the presence of perforated cytoplasm indicating the disintegration of the cytoplasmic content. These types of cells which were referred to as light chondrocytes were thought to be the hypertrophic cells undergoing terminal differentiation as described in the studies above. The fact that they were heavily loaded with RER indicated that they were undergoing a last burst of protein synthesis and release which could ultimately results in their own destruction and replacement by oesteogenic cells as described in other studies [23].
Control and pretreated chondrocytes cultures grown to day 14 were fixed in their culture dishes and type X collagen was localized by the monoclonal antibody which recognizes the helical domain of type X collagen. The control cultures showed negative staining while the treated cultures showed positive staining which was localized in the cell aggregates (Fig. 6A). This was further confirmed by the immunoblotting technique, which showed the expression of type X collagen at day 7 which became the major biosynthetic product at day 14 in culture (Fig. 6B).
Culture conditions plays a profound role on cell morphology and differentiation [28]. It has been proposed that epigenetics is involved in the phenotypic modulation that articular cartilage undergoes in osteoarthritis, which results in extensive degradation of cartilage specific ECM replacing it with the hypertrophy of the joint capsule [212930]. In this study, we used the hypomethylating drug 5-aza-2'-deoxycytidine on the embryonic chick sternal caudal region chondrocytes and studied the phenotypic and biosynthetic modifications resulted with the pretreatment. We found that pretreatment caused profound changes in the gene expression which had been reflected in their morphology and the ECM secretion.
It was also interesting to note that unlike 5-azacytidine, which can intercalate in both DNA and RNA could be effective in inducing differentiation and morphological changes to the cells at any stage of cell cycle and development. 5-Aza-2'-deoxycytidine has the ability to only intercalate in DNA, so it is most effective at the replicative stage of cells. This analogue replaces normal cytosine and forms irreversible adducts with dimethyl triptamine (DMTs), thus inhibiting genome wide methylation [11]. Once the methylation pattern of the growing cells was altered by the treatment of the demethylation drug 5-aza-2'-deoxycytidine, growing in its absence would make no difference to the cells as the change in pattern of methylation was conserved and successfully maintained in their daughter cells [3132].
During endochondral ossification, the differentiation of growth plate chondrocytes is accompanied by an expression of a number of genes which resulted in the ECM remodeling. The histological studies were carried out in order to confirm that not only the chondrocytes phenotypes was altered on pretreatment, but the ECM secreted by them was also altered, with a loss of cartilage specific ECM. Scanning electron microscopy studies further shed light on the fine details of the ECM secreted by both control and pretreated chondrocytes and indicated the presence of matrix vesicles in the ECM of the pretreated cultures which is a characteristic feature of the hypertrophic chondrocytes.
The ultimate fate of the hypertrophic chondrocytes remained a highly controversial issue. Some studies indicated that terminal hypertrophic remains alive and change into osteoblasts and actively participate in tissue remodeling changing it from cartilage specific ECM to osteogenic ECM [82733]. However, there is substantial evidence suggesting the apoptosis to be the final fate of the terminal hypertrophic chondrocytes [3435]. Our TEM studies supported the notion of two types of cells termed as dark cell and light cells, which has been reported in other avian growth plate studies [836], which undergoes its own course of terminal hypertrophic differentiation and apoptosis.
This study further emphasizes the role of methylation as an important epigenetic mechanism in the differentiation of chondrocytes towards its terminal fate.
Our results support the notion that epigenetic mechanisms such as methylation play an important role in chondrocyte maturation pathway. The culture system described here proved to be the exact mimic of the processes taking place during endochondral ossification and thus potentially used as in-vitro model of bone formation. This culture system could be further exploited to study different regulatory pathways and molecular mechanisms that initiate the entry of epiphyseal chondrocytes into differentiation pathway, which could help us in better understanding of various pathological states of EO.
Figures and Tables
Fig. 1
Effect of 5-aza-2'-deoxycytidine on cell size with time in culture. The diameter of 5-aza-2'-deoxycytidine treated and untreated cells were measured at each indicated time point. Their cell volumes were calculated on the assumption that chondrocytes were spherical in shape. One hundred cells were measured at each time point in each culture. This was repeated in 10 cultures. Value represent mean±SEM (100 cells were measured for each time point in 10 experiments).
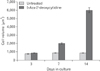
Fig. 2
Morphological effect of decitabine on chondrocyte morphology. The control and pretreated with 5-aza-2'-deoxycytidine caudal region chick sternal chondrocytes at day 7 in culture were stained with alcian blue, acridine orange and toluidine blue. (A) The control cultures stained with Acridine orange. (B) The pretreated chondrocytes cultures stained with Acridine orange (A and B, ×80). (C) The control cells stained with Alcian blue. (D) The pretreated cultures stained with Alcian blue (C and D, ×103). (E) The control cells stained with toluidine blue. (F) The pretreated cultures stained with toluidine blue (E and F, ×80). The arrows indicated the toluidine blue staining negative cells in the cultures.
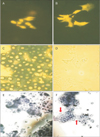
Fig. 3
Scanning electron microscopic photomicrographs of the chondrocytes in culture. (A) Control chondrocytes at day 7 in culture. (B) Pretreated chondrocytes at day 7 in culture. (C) Extracellular matrix secreted by control chondrocytes at day 14 in culture. (D) Extracellular matrix secreted by pretreated chondrocytes at day 14 as described in "Materials and Methods." Note the presence of electron dense particles extensively present in the pretreated cultures. Scale bars=4.90 µm (A), 4.99 µm (B), 2 µm (C, D).
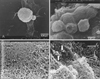
Fig. 4
Histogram showing the relative proportion of alkaline phosphatase (ALP) enzyme activity in the control and pretreated cultures at day 7 and day 14. ALP enzyme assay was done on triplicate samples as described in "Materials and Methods" section and expressed as per microgram of DNA.
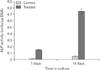
Fig. 5
Transmission electron microscopic photomi crograph of the control and treated chondrocytes. The control and pretreated chondrocytes at day 14 were examined under the transmission electron microscope. (A) Control chondroc ytes. (B) Pretreated chondrocyte. (C) The control chondrocytes. (D) Pretreated chondrocytes. Note the presence of extensive and well defined rough endoplasmic reticulum (RER) and euchromatic nucleus and condensed chromatin in the pretreated cultures. Scale bars=2.01 µm (A, B), 2 µm (C, D). N, nucleus; V, vesicle; M, mitochodria.
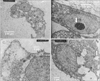
Fig. 6
(A) Immunolocalization of type X collagen in chondrocyte cultures. Caudal region sternal chondrocytes pretreated with 5-aza-2'-deoxycytidine (5-aza-dC) and grown for day 14 in culture were fixed and the type X collagen localized in the culture by treating with a monoclonal antibody known to recognize the helical domain of type X collagen. Bound antibody was reacted with a second antibody IgG-linked to alkaline phosphatase and after a subsequent color reaction the site of type X collagen in the culture could be identified by red staining. (B) Western blotting with type X monoclonal antibody and fibronectin antibody. Medium proteins of the 14-day control and pretreated cultures were recovered after 30% ammonium sulphate precipitation and run on 8% sodium dodecyl sulfate polyacrylamide gel electrophoresis. The proteins were transferred onto a nitrocellulose membrane and immune-blotted with fibronectin and type X monoclonal antibody.
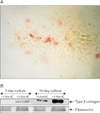
Acknowledgements
This research was supported by Deanship of Research Centre for Scientific and Medical Colleges, King Saud University.
References
1. Marino R. Growth plate biology: new insights. Curr Opin Endocrinol Diabetes Obes. 2011; 18:9–13.
2. Chen KS, Tatarczuch L, Mirams M, Ahmed YA, Pagel CN, Mackie EJ. Periostin expression distinguishes between light and dark hypertrophic chondrocytes. Int J Biochem Cell Biol. 2010; 42:880–889.
3. Mackie EJ, Tatarczuch L, Mirams M. The skeleton: a multi-functional complex organ: the growth plate chondrocyte and endochondral ossification. J Endocrinol. 2011; 211:109–121.
4. McDevitt CA. Biochemistry of articular cartilage. Nature of proteoglycans and collagen of articular cartilage and their role in ageing and in osteoarthrosis. Ann Rheum Dis. 1973; 32:364–378.
5. Bruckner P, Hörler I, Mendler M, Houze Y, Winterhalter KH, Eich-Bender SG, Spycher MA. Induction and prevention of chondrocyte hypertrophy in culture. J Cell Biol. 1989; 109:2537–2545.
6. Wu LN, Genge BR, Wuthier RE. Association between proteoglycans and matrix vesicles in the extracellular matrix of growth plate cartilage. J Biol Chem. 1991; 266:1187–1194.
7. Bianco P, Cancedda FD, Riminucci M, Cancedda R. Bone formation via cartilage models: the "borderline" chondrocyte. Matrix Biol. 1998; 17:185–192.
8. Roach HI, Erenpreisa J, Aigner T. Osteogenic differentiation of hypertrophic chondrocytes involves asymmetric cell divisions and apoptosis. J Cell Biol. 1995; 131:483–494.
9. Adams CS, Shapiro IM. The fate of the terminally differentiated chondrocyte: evidence for microenvironmental regulation of chondrocyte apoptosis. Crit Rev Oral Biol Med. 2002; 13:465–473.
10. Horvitz HR, Herskowitz I. Mechanisms of asymmetric cell division: two Bs or not two Bs, that is the question. Cell. 1992; 68:237–255.
11. Christman JK. 5-Azacytidine and 5-aza-2'-deoxycytidine as inhibitors of DNA methylation: mechanistic studies and their implications for cancer therapy. Oncogene. 2002; 21:5483–5495.
12. Brank AS, Eritja R, Garcia RG, Marquez VE, Christman JK. Inhibition of HhaI DNA (Cytosine-C5) methyltransferase by oligodeoxyribonucleotides containing 5-aza-2'-deoxycytidine: examination of the intertwined roles of co-factor, target, transition state structure and enzyme conformation. J Mol Biol. 2002; 323:53–67.
13. Zhou GS, Zhang XL, Wu JP, Zhang RP, Xiang LX, Dai LC, Shao JZ. 5-Azacytidine facilitates osteogenic gene expression and differentiation of mesenchymal stem cells by alteration in DNA methylation. Cytotechnology. 2009; 60:11.
14. Moazedi-Fuerst FC, Hofner M, Gruber G, Weinhaeusel A, Stradner MH, Angerer H, Peischler D, Lohberger B, Glehr M, Leithner A, Sonntagbauer M, Graninger WB. Epigenetic differences in human cartilage between mild and severe OA. J Orthop Res. 2014; 32:1636–1645.
15. Rushton MD, Reynard LN, Barter MJ, Refaie R, Rankin KS, Young DA, Loughlin J. Characterization of the cartilage DNA methylome in knee and hip osteoarthritis. Arthritis Rheumatol. 2014; 66:2450–2460.
16. D'Angelo M, Pacifici M. Articular chondrocytes produce factors that inhibit maturation of sternal chondrocytes in serum-free agarose cultures: a TGF-beta independent process. J Bone Miner Res. 1997; 12:1368–1377.
17. Von Bertalanffy L, Masin M, Masin F. A new and rapid method for diagnosis of vaginal and cervical cancer by fluorescence microscopy. Cancer. 1958; 11:873–887.
18. Steven FS, Suresh U, Wong TL, Griffin MM. The role of inhibitors in the fluorescent staining of benign naevus and malignant melanoma cells with 9-amino acridine and acridine orange. J Enzyme Inhib. 1987; 1:275–287.
19. Pashley DH, Tao L, Boyd L, King GE, Horner JA. Scanning electron microscopy of the substructure of smear layers in human dentine. Arch Oral Biol. 1988; 33:265–270.
20. Lowry OH, Rosebrough NJ, Farr AL, Randall RJ. Protein measurement with the Folin phenol reagent. J Biol Chem. 1951; 193:265–275.
21. Cheung JO, Hillarby MC, Ayad S, Hoyland JA, Jones CJ, Denton J, Thomas JT, Wallis GA, Grant ME. A novel cell culture model of chondrocyte differentiation during mammalian endochondral ossification. J Bone Miner Res. 2001; 16:309–318.
22. Buckwalter JA, Mower D, Ungar R, Schaeffer J, Ginsberg B. Morphometric analysis of chondrocyte hypertrophy. J Bone Joint Surg Am. 1986; 68:243–255.
23. Poole CA, Flint MH, Beaumont BW. Chondrons in cartilage: ultrastructural analysis of the pericellular microenvironment in adult human articular cartilages. J Orthop Res. 1987; 5:509–522.
24. D'Angelo M, Billings PC, Pacifici M, Leboy PS, Kirsch T. Authentic matrix vesicles contain active metalloproteases (MMP): a role for matrix vesicle-associated MMP-13 in activation of transforming growth factor-beta. J Biol Chem. 2001; 276:11347–11353.
25. Golub EE. Role of matrix vesicles in biomineralization. Biochim Biophys Acta. 2009; 1790:1592–1598.
26. Roach HI, Clarke NM. "Cell paralysis" as an intermediate stage in the programmed cell death of epiphyseal chondrocytes during development. J Bone Miner Res. 1999; 14:1367–1378.
27. Roach HI, Clarke NM. Physiological cell death of chondrocytes in vivo is not confined to apoptosis. New observations on the mammalian growth plate. J Bone Joint Surg Br. 2000; 82:601–613.
28. Younesi E, Bayati V, Hashemitabar M, Azandeh SS, Bijannejad D, Bahreini A. Differentiation of adipose-derived stem cells into Schwann-like cells: fetal bovine serum or human serum? Anat Cell Biol. 2015; 48:170–176.
29. Blanco FJ, Rego-Pérez I. Editorial: Is it time for epigenetics in osteoarthritis? Arthritis Rheumatol. 2014; 66:2324–2327.
30. Barter MJ, Bui C, Young DA. Epigenetic mechanisms in cartilage and osteoarthritis: DNA methylation, histone modifications and microRNAs. Osteoarthritis Cartilage. 2012; 20:339–349.
31. Chen T, Ueda Y, Dodge JE, Wang Z, Li E. Establishment and maintenance of genomic methylation patterns in mouse embryonic stem cells by Dnmt3a and Dnmt3b. Mol Cell Biol. 2003; 23:5594–5605.
32. Bird A. DNA methylation patterns and epigenetic memory. Genes Dev. 2002; 16:6–21.
33. Pound JC, Green DW, Roach HI, Mann S, Oreffo RO. An ex vivo model for chondrogenesis and osteogenesis. Biomaterials. 2007; 28:2839–2849.
34. Gibson G, Lin DL, Roque M. Apoptosis of terminally differentiated chondrocytes in culture. Exp Cell Res. 1997; 233:372–382.
35. Cheung JO, Grant ME, Jones CJ, Hoyland JA, Freemont AJ, Hillarby MC. Apoptosis of terminal hypertrophic chondrocytes in an in vitro model of endochondral ossification. J Pathol. 2003; 201:496–503.
36. Ahmed YA, Tatarczuch L, Pagel CN, Davies HM, Mirams M, Mackie EJ. Physiological death of hypertrophic chondrocytes. Osteoarthritis Cartilage. 2007; 15:575–586.