Abstract
We examined morphological differences between the sublingual and submandibular glands with special reference to their innervation. The sublingual gland contained abundant periodic acid Schiff-positive mucous acini: some lobules were composed of purely mucous acini, while others were purely serous or mixed. However, in the submandibular gland, the area of mucous acini was very limited. Notably, in the sublingual gland, immunohistochemistry for neuron-specific enolase demonstrated that the serous acini carried a higher density of nerve elements than the mucous acini. However, no such difference was evident in the submandibular gland, possibly due to the small areas of the mucous acini. In both types of gland, neuronal nitric oxide synthase-positive parasympathetic nerves as well as tyrosine hydroxylase-positive sympathetic nerves were observed in the interlobular tissue, but we were unable to trace these thin fibers to the acini. Myoepithelial cells expressed smooth muscle actin, but were negative for S100B protein, glial fibrillary acidic protein and neuron-specific enolase. However, antibody against S100A stained some of the myoepithelial cells and ductal cells in the sublingual gland. Cells positive for peripheral myelin protein 22 were seen in some of the ductal cells in the submandibular gland, but not in the sublingual gland. Therefore, with regard to the neurogenic features of the gland cells, S100B reactivity might disappear first in postnatal life, whereas S100A reactivity is likely to remain as aging progresses. The sublingual gland in elderly individuals seems to provide a good model for comparison of the nerve supply between mucous and serous acini.
The major and minor salivary glands express nerve markers such as glial fibrillary acidic protein (GFAP; one of the intermediate filament proteins), S100 protein (a well known marker of glial and Schwann cells) and neuron-specific enolase (NSE) under normal conditions in the absence of cancer [1, 2, 3, 4]. These neural markers, as well as protein markers of smooth muscle (e.g., alpha smooth muscle actin; SMA) are usually used for classification of salivary gland tumors [5, 6, 7]. Using human midterm fetuses, Katori et al. [8] reported that, in contrast to the nearby submandibular gland, the sublingual gland has a neurogenic immunohistochemical character similar to that of small glands such as the palatal, pharyngeal and lingual glands.
Several studies using rats have indicated that a difference between the submandibular and sublingual glands is evident not only in the gland tissue itself but also in its innervation. Ekström et al. [9] described that, in contrast to the sublingual gland, the usual co-presence of the sensory nerve markers, calcitonin gene-related peptide and substance P, is absent in the rat submandibular gland. In a pharmacological study using rats, Mirfendereski et al. [10] reported a much stronger parasympathetic nerve response (to pituitary adenylate cyclase activating peptide) in the submandibular gland than the sublingual gland. Likewise, Takai et al. [11] reported a much denser supply of neuronal nitric oxide synthase (nNOS)-positive parasympathetic neuronal nitric in the submandibular gland than the sublingual gland of rats. Finally, also in rats, Tobin et al. [12] described that muscarinic receptor subtype M1 is predominant in the sublingual gland, in contrast to predominance of M5 in the submandibular gland, although M1 induces indirect effects via nitric oxide. These differences were not demonstrated in human specimens, possibly due to experimental difficulties.
Consequently, using specimens from elderly donated cadavers, the primary aim of this study was to comprehensively examine the immunohistochemical reactivity of usual marker proteins in the submandibular and sublingual glands. In addition to the aforementioned neural markers (GFAP, S100 protein, NSE, and nNOS), we also studied peripheral myelin protein 22 (PMP22), which has been used for staining of peripheral nerves (e.g., Gregson et al. [13]), and shows mutations in some forms of human neuropathy such as Charcot-Marie-Tooth disease [14]. Our secondary aim was to compare the expressions of these proteins between mucous and serous areas in mixed glands, for which we considered the best strategy would be to compare between mucous acini-predominant lobules with serous acini-predominant lobules.
The study was performed in accordance with the provisions of the Declaration of Helsinki 1995 (as revised in Edinburgh 2000). We examined 15 donated cadavers (7 men and 8 women) ranging in age from 78 to 95 years, with a mean age of 88 years. The cause of death had been ischemic heart failure or intracranial bleeding. These cadavers had been donated to Tokyo Dental College for research and education on human anatomy, and their use for research had been approved by the university ethics committee. The donated cadavers had been fixed by arterial perfusion of 10% v/v formalin solution and stored in 50% v/v ethanol solution for more than 3 months. From each of the cadaveric heads, we prepared 2 tissue blocks (the submandibular gland and sublingual glands). For easy discrimination of sections, the sublingual gland contained a small area of the oral mucosa. Thus, the sublingual gland was cut almost along the long axis, in contrast to the submandibular gland, which was cut transversely. After performing routine procedures for paraffin-embedded histology, 9-10 serial sections were prepared: two of them were stained with hematoxylin and eosin and with periodic acid Schiff reaction (PAS), respectively, while the others were used for immunohistochemistry (IHC).
The primary antibodies used for IHC were (1) rabbit polyclonal anti-human GFAP (1:100, Z0334, Dako Cytomation, Kyoto, Japan); (2) rabbit polyclonal anti-human S100 protein (1:100, N1573 or Z0311, Dako, Glostrup, Denmark); (3) mouse monoclonal anti-human NSE (1:100, N1557, Dako); (4) rabbit polyclonal anti-human PMP22 (1:1,000, p0078, Sigma-Aldrich, St. Louis, MO, USA); (5) rabbit polyclonal anti-human neuronal nNOS (1:200, Cell Signaling Technology, Beverly, MA, USA); (6) rabbit polyclonal anti-human tyrosine hydroxylase (1:500, ab152, Millipore-Chemicon, Temecula, CA, USA); and (7) mouse monoclonal anti-human alpha SMA (1:100, M0851, Dako). Among these antibodies, Nos. 1-6 were for demonstration of nerve supply to the glands, while No. 7 was for identification of myoepithelial cells. Except for the antibodies against S100 and SMA, antigen retrieval was performed using microwave treatment (500 W, 15 minutes, pH 6). The secondary antibody (incubation for 30 minutes, 1:1,000, Histofine Simple Stain Max-PO, Nichirei, Tokyo, Japan) was labeled with horseradish peroxidase (HRP), and antigen-antibody reactions were detected by HRP-catalyzed reaction with diaminobenzidine (incubation for 3-5 minutes; Histofine Simple Stain DAB, Nichirei). All samples were counterstained with hematoxylin. Samples without the primary antibody were used as negative controls. The present S100 antibody, N1573, reacts strongly with S100B protein but weakly or very weakly with S100A protein, whereas Z0311 is specific for S100A protein. The present anti-SMA antibody reacts strongly with the endothelium of arteries and veins [15]. We counted NSE-positive nerve elements in the whole sectional area of both mucous and serous lobules using ×10 or ×20 objectives. The data was evaluated using t test.
PAS staining clearly demonstrated a mosaic pattern formed by the mucous and serous acini in the sublingual and submandibular glands (Figs. 1, 2, 3). The sublingual gland was comprised of 30-60 round or triangular lobules in each section, while the submandibular gland had 60-120 quadrate, triangular or irregularly shaped lobules per section. Although the present observations were not three-dimensional, the lobules of the sublingual gland were classifiable into three patterns: (1) lobules composed purely of mucous acini; (2) lobules composed purely of serous acini; and (3) lobules containing both types of acini (i.e., actual mixed glands). The mucous lobules numbered 3-8 per section (around 10%), the serous lobules 5-15 (around 20%), and the others-accounting for the majority-were regarded as mixed lobules. However, in the submandibular gland, the mucous acini, if present, were restricted to a small area in each lobule. Thus, the majority of lobules were serous, and mixed lobules accounted for less than 10% of the total. In the interlobar tissue, nNOS-positive as well as TH-positive sympathetic nerves were easily found. However, we were unable to trace them to areas around the acini because of the limited nature of the present IHC (Fig. 3).
In both types of gland, myoepithelial cells expressed SMA, but IHC showed them to be negative for S100B (N1573 antibody), GFAP, and NSE (Figs. 4, 5). However, S100A antibody (Z0311) stained some of the myoepithelial cells and ductal cells in the sublingual gland (Fig. 3C). The positive part of the duct appeared to be intercalated, but most of the duct was negative. Thin nerve fibers in the arterial wall expressed GFAP (Figs. 4C, D, 5E, F). Blood cells as well as some of the submandibular ductal cells expressed PMP22 (Fig. 4F, I, J). The PMP22-positive part of the duct appeared to be the striated part, but most of the striated duct was PMP22-negative. In the interlobular tissue, thick myelinated nerve fibers expressed PMP (Fig. 4K) as well as S100 (Figs. 4A, B, 5C, D). Therefore, in the lobule, nerve elements along and around each of the acini were easily identified by IHC for NSE rather than for S100. SMA reactivity of myoepithelial cells was seen in both mucous and serous acini.
In the sublingual gland, the lobule containing serous acini showed a higher density of NSE-positive nerve elements than that containing mucous acini (Fig. 1B, C). The difference in density reached 10-fold at maximum: 300-800 NSE-positive cells per 1 mm2 in the serous lobule vs. 50-200 per 1 mm2 in the mucous lobule (Table 1). The difference in the sublingual gland was statistically significant (P<0.0001). Likewise, in the mixed lobule where serous and mucous acini were present in the same section, we found a clear demarcation between nerve-rich and nerve-poor areas (Fig. 1D). However, in the submandibular gland, where mucous acini were very sparse, the nerve elements were distributed almost evenly along and around the acini in any lobule (400-900 positive cells per 1 mm2). We examined and compared the NSE-positive nerve elements in the whole sectional area of both mucous and serous lobules using ×10 or ×20 objectives. Conversely, when we counted positive cells within a small area under a ×40 objective, limited nerve-rich areas were likely to be found even in lobules containing mucous acini. Finally, we did not find either a gender difference or a difference depending on ages.
A well-known textbook [16] states that S100 expression is evident in the intercalated duct and myoepithelial cells of the major salivary glands. Although Katori et al. [8] expected that the adult sublingual gland would show strong neurogenic immunoreactivity of myoepithelial cells, the present study demonstrated negativity for S100B, GFAP, and NSE. In contrast, S100A antibody (Z0311) stained some of the myoepithelial cells and ductal cells. In the sublingual gland, the fetal neurogenic characteristics of myoepithelial cells, such as expression of S100 or GFAP, seemed to become reduced or disappear during postnatal life and/or with aging. Lee et al. [3] and Chi [17] stated that S100B reactivity was limited to fetuses, and was not present in adults. Likewise, Mori et al. [18] and Dardick et al. [19] reported that myoepithelial cells of the adult major salivary glands were negative for S100. As suggested by Korean pathologist [3, 17], the varying results for S100 immunoreactivity in references were probably due to poor discrimination between S100A and S100B by the antibodies employed. In the sublingual gland, S100B reactivity might disappear first during postnatal life, whereas S100A reactivity is likely to be retained during subsequent aging. We found PMP22-positive ducts in the submandibular gland. The positivity appeared to correspond to the striated part of the duct, but most of the duct was negative. Thus, PMP22 positivity may have reflected a specific physiological condition of the ducts, such as degeneration. Taken together, our findings suggested that a proportion of salivary gland cells retain neurogenic features during postnatal life.
To our knowledge, Kusakabe et al. [20] are the only group to have reported a difference in innervation between the mucous and serous acini in the human submandibular gland. In their excellent IHC study using cryostat sections of specimens from relatively younger patients, they found that neuropeptide Y-positive nerve fibers (candidate sympathetic nerves) as well as vasoactive intestinal polypeptide-positive nerve fibers (candidate parasympathetic nerves) were more densely distributed around mucous acini than around serous acini. They considered that such peptidergic nerves may play a more active role in regulating the secretory mechanisms in the former than in the latter. Although the difference we noted was not in the submandibular gland but the sublingual gland, the findings of Kusakabe et al. [20] differed from our present result. It seems difficult to evaluate differences in very thin nerves between adjacent small areas because irregular staining is liable to occur, especially in cryostat sections.
Careful evaluation of IHC results is necessary, using not only high-magnification but also lower-magnification views. In fact, when we counted NSE-positive cells within a small area under a ×40 objective, nerve-rich areas tended to be revealed even in lobules occupied by mucous acini. Therefore, it was not possible to effectively compare mucous and serous acini in the submandibular gland of elderly individuals because of the very small mucous area surrounded by a large serous area. In contrast, the sublingual gland of elderly individuals provided a good model for such a comparison because purely mucous lobules were present with purely serous lobules in each section. Using this model, we intend to further examine differences in nerve supply. The present counting of NSE-positive cells revealed a statistically significant difference between the serous and mucus lobules (Table 1). However, the positive cells were not always nerve-associated cells but they were likely to include neurogenic mesenchymal cells. The present method, counting cells, might not be the best way for a quantitative analysis of nerves.
Figures and Tables
Fig. 1
Sublingual gland obtained from an 83-year-old female cadaver. (A) Distribution of the mucous and serous glands: dark violet indicates the mucous glands (periodic acid Schiff staining). One lobule (with square B) is composed of mucous acini, whereas the other lobule (with square C) contains only serous acini. Panels (B-D) show higher-magnification views of the squares marked B, C and D in panel (A), respectively (immunohistochemistry for neuron-specific enolase or neuron-specific enolase [NSE]). The lobule containing serous acini (C) shows a higher density of NSE-positive neural elements than the lobule containing mucous acini (B). (D) The border between the mucous and serous gland areas in a lobule (marked D in panel A) containing both types of acini. Scale bars=1 mm (A), 0.1 mm (B-D).
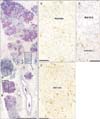
Fig. 2
Submandibular gland obtained from an 83-year-old female cadaver. (A) The distribution of the mucous and serous glands (periodic acid Schiff staining). The mucous gland acini are restricted to a small area in each lobule. Panels (B) and (C) show higher-magnification views of the squares marked B and C in panel (A), respectively (immunohistochemistry for neuron-specific enolase or neuron-specific enolase [NSE]). There is no clear difference in the density of NSE-positive neural elements between the area rich in mucous acini (B) and that rich in serous acini (C). Scale bars=1 mm (A), 0.1 mm (B, C).
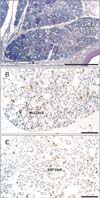
Fig. 3
Innervation of the sublingual and submandibular glands obtained from a 78-year-old male cadaver. Using near sections, panels (A), (C), (E), (G), and (I) (or B, D, F, H, and J) display the sublingual (or submandibular) gland. Panels (C), (E), (G), and (I) (or D, F, H, and J) are higher-magnification views of the square in panel (A) (or panel B). Panels (A) and (B) show periodic acid Schiff staining: the sublingual gland contains an area of serous acini or lobules (serous in panel A), whereas in the submandibular gland the area of mucous acini is very limited (mucous in panel B). Immunohistochemistry for S100A protein (Z0311) (C, D), neuron-specific enolase (NSE) (E, F), neuronal nitric oxide synthase (NOS) (G, H), and tyrosine hydroxylase (TH) (I, J). Some of the myoepithelial cells and ductal cells are positive for S100 (arrowheads in panel C). Interlobar nerves express both NOS (arrows in panels G and H) and TH (arrows in panels I and J), but some ducts are also positive for both markers (open stars in panels G, H, and J). Scale bars=1 mm (A, B), 0.1 mm (C-J).
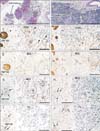
Fig. 4
Immunohistochemistry of myoepithelial cells in the lingual and submandibular glands from an 83-year-old female cadaver. The same specimen as that shown in Figs. 1 and 2. Using near sections, panels (A), (C), (E), and (G) (or B, D, F, and H) display the sublingual (or submandibular) gland. Open stars indicate the same acinus in corresponding panels in the sublingual gland, while closed stars indicate the corresponding sites in the submandibular gland. In both glands, most of the myoepithelial cells are positive for smooth muscle actin (SMA) (arrows in panels G and H), but negative for either S100 protein (mainly S100B, N1573) (A, B), glial fibrillary acidic protein (GFAP) (C, D) or peripheral myelin protein 22 (PMP) (E, F). Thin nerve fibers in the arterial wall express GFAP (arrowheads in panels C and D). Blood cells (arrows in panel F) as well as some of the submandibular ductal cells express PMP (circle marked I in panel F). Panel (I) shows a higher-magnification view of the PMP-positive duct in panel (F), and panel (J) shows positive ducts in another section of the same specimen. In interlobular tissues of both glands, thick myelinated nerve fibers express PMP strongly (K). Scale bars=0.1 mm (A-K).
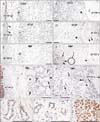
Fig. 5
Immunohistochemistry of myoepithelial cells in the lingual and submandibular glands from a 78-year-old male cadaver. The same specimen as that shown in Fig. 3. Using near sections, panels (A), (C), (E), and (G) (or B, D, F and H) display the sublingual (or submandibular) gland. Open stars indicate the same acinus in corresponding panels in the sublingual gland, while closed stars indicate the corresponding sites in the submandibular gland. In both glands, most of the myoepithelial cells are positive for smooth muscle actin (SMA) (arrows in panels G and H), but negative for either neuron specific enolase (NSE) (A, B), S100 protein (mainly S100B, N1573) (C, D) or glial fibrillary acidic protein (GFAP) (E, F). Thin nerve elements around the acini tend to express NSE strongly, while thick nerves are stained dark for S100. Thin nerve fibers in the arterial wall express GFAP (arrows in panels E and F). Scale bars=0.1 mm (A-H).
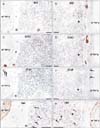
Table 1
Neuron-specific enolase-positive cell number in the mucus or serous lobule in the sublingual gland
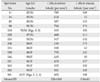
Specimen No. | Age (y)/Gender | Cells in serous lobule (per mm2) | Cells in mucus lobule (per mm2) |
---|---|---|---|
11 | 82/M | 785 | 169 |
16 | 92/M | 624 | 52 |
82 | 85/M | 587 | 125 |
94 | 91/M | 794 | 174 |
119 | 78/M (Figs. 3, 5) | 315 | 195 |
120 | 87/M | 468 | 111 |
153 | 93/M | 347 | 135 |
108 | 89/F | 510 | 175 |
115 | 84/F | 310 | 62 |
130 | 86/F | 755 | 142 |
146 | 90/F | 645 | 85 |
151 | 91/F | 433 | 127 |
163 | 95/F | 325 | 195 |
182 | 93/F | 381 | 105 |
191 | 83/F (Figs. 1, 2, 4) | 605 | 188 |
Mean±SD | 526±168 | 136±45 |
Acknowledgements
We are grateful to people who donated their bodies after their death to Tokyo Dental College for research and education on human anatomy without any economical benefit. We also thank to their families for agreement of the donation as well as patience for waiting the return their bones after study.
References
1. Nakazato Y, Ishida Y, Takahashi K, Suzuki K. Immunohistochemical distribution of S-100 protein and glial fibrillary acidic protein in normal and neoplastic salivary glands. Virchows Arch A Pathol Anat Histopathol. 1985; 405:299–310.
2. Gustafsson H, Virtanen I, Thornell LE. Glial fibrillary acidic protein and desmin in salivary neoplasms. Expression of four different types of intermediate filament proteins within the same cell type. Virchows Arch B Cell Pathol Incl Mol Pathol. 1989; 57:303–313.
3. Lee SK, Kim EC, Chi JG, Hashimura K, Mori M. Immunohistochemical detection of S-100, S-100 alpha, S-100 beta proteins, glial fibrillary acidic protein, and neuron specific enolase in the prenatal and adult human salivary glands. Pathol Res Pract. 1993; 189:1036–1043.
4. Okura M, Hiranuma T, Tominaga G, Yoshioka H, Aikawa T, Shirasuna K, Matsuya T. Expression of S-100 protein and glial fibrillary acidic protein in cultured submandibular gland epithelial cells and salivary gland tissues. Histogenetic implication for salivary gland tumors. Am J Pathol. 1996; 148:1709–1716.
5. Ogawa I, Nishida T, Miyauchi M, Sato S, Takata T. Dedifferentiated malignant myoepithelioma of the parotid gland. Pathol Int. 2003; 53:704–709.
6. Angiero F, Sozzi D, Seramondi R, Valente MG. Epithelial-myoepithelial carcinoma of the minor salivary glands: immunohistochemical and morphological features. Anticancer Res. 2009; 29:4703–4709.
7. Santos EP, Cavalcante DR, Melo AU, Pereira JC, Gomes MZ, Albuquerque RL Jr. Plasmacytoid myoepithelioma of minor salivary glands: report of case with emphasis in the immunohistochemical findings. Head Face Med. 2011; 7:24.
8. Katori Y, Hayashi S, Takanashi Y, Kim JH, Abe S, Murakami G, Kawase T. Heterogeneity of glandular cells in the human salivary glands: an immunohistochemical study using elderly adult and fetal specimens. Anat Cell Biol. 2013; 46:101–112.
9. Ekstrom J, Ekman R, Håkanson R, Sjögren S, Sundler F. Calcitonin gene-related peptide in rat salivary glands: neuronal localization, depletion upon nerve stimulation, and effects on salivation in relation to substance P. Neuroscience. 1988; 26:933–949.
10. Mirfendereski S, Tobin G, Håkanson R, Ekström J. Pituitary adenylate cyclase activating peptide (PACAP) in salivary glands of the rat: origin, and secretory and vascular effects. Acta Physiol Scand. 1997; 160:15–22.
11. Takai N, Uchihashi K, Higuchi K, Yoshida Y, Yamaguchi M. Localization of neuronal-constitutive nitric oxide synthase and secretory regulation by nitric oxide in the rat submandibular and sublingual glands. Arch Oral Biol. 1999; 44:745–750.
12. Tobin G, Giglio D, Gotrick B. Studies of muscarinic receptor subtypes in salivary gland function in anaesthetized rats. Auton Neurosci. 2002; 100:1–9.
13. Gregson NA, Zhang G, Pritchard J, Wang A, Sanvito L, Hayday AC, Hughes RA. Characterization of a monoclonal antibody specific for human peripheral myelin protein 22 and its use in immunohistochemical studies of the fetal and adult nervous system. J Peripher Nerv Syst. 2007; 12:2–10.
14. Rosso G, Negreira C, Sotelo JR, Kun A. Myelinating and demyelinating phenotype of Trembler-J mouse (a model of Charcot-Marie-Tooth human disease) analyzed by atomic force microscopy and confocal microscopy. J Mol Recognit. 2012; 25:247–255.
15. Hayashi S, Murakami G, Ohtsuka A, Itoh M, Nakano T, Fukuzawa Y. Connective tissue configuration in the human liver hilar region with special reference to the liver capsule and vascular sheath. J Hepatobiliary Pancreat Surg. 2008; 15:640–647.
16. Martínez-Madrigal F, Bosq J, Casiraghi O. Major salivary glands. In : Sternberg SS, editor. Histology for Pathologists. 2nd ed. Philadelphia: Lippincott Williams & Wilkins;1997. p. 405–429.
17. Chi JG. Prenatal development of human major salivary glands. Histological and immunohistochemical characteristics with reference to adult and neoplastic salivary glands. J Korean Med Sci. 1996; 11:203–216.
18. Mori M, Murase N, Hosaka M, Orito T. Immunohistochemical expression of S-100 protein in reactive and neoplastic myoepithelial cells of variant salivary pleomorphic adenomas. Acta Histochem Cytochem. 1986; 19:231–240.
19. Dardick I, Stratis M, Parks WR, DeNardi FG, Kahn HJ. S-100 protein antibodies do not label normal salivary gland myoepithelium. Histogenetic implications for salivary gland tumors. Am J Pathol. 1991; 138:619–628.
20. Kusakabe T, Matsuda H, Kawakami T, Syoui N, Kurihara K, Tsukuda M, Takenaka T. Distribution of neuropeptide-containing nerve fibers in the human submandibular gland, with special reference to the difference between serous and mucous acini. Cell Tissue Res. 1997; 288:25–31.