Abstract
Mesenchymal stem cells (MSCs) offer significant promise as a multipotent source for cell-based therapies and could form the basis for the differentiation and cultivation of tissue grafts to replace damaged tissue. However, no gene expression follow up analysis has been undertaken to characterize the in vitro adipogenic differentiated MSCs. The main goal of this study was to focus on MSCs and to analyze their differentiation capacity. To achieve this aim, bone marrow MSCs from sprague dawely rats were isolated, expanded in monolayer culture and characterized with respect to their cluster of differentiation (CD) and ability for adipogenic differentiation capacity. The expression of CD44, CD45, CD29, CD34, and CD90 on bone marrow derived MSCs was characterized using flow cytometry. Adipogenesis was determined by staining with oil-red O and reverse transcription polymerase chain reaction assessments of lipoprotein lipase, leptin, adiponectin and adipocyte genes at different time intervals, after 4, 7, 14, and 21 days. Our results revealed that the pattern of CD marker expression was highly positive significant with CD29, CD44, and CD90 when compared with CD34 and CD45. MSCs showed proliferative potential and were capable of adipogenic differentiation characterized by reddish brown-droplets following staining with oil-red O and expression of molecular bands of genes. These results demonstrate, at the morphological, immunophenotyping and gene expression levels, the multipotency of MSCs and thus highlight their potential therapeutic value for cell-based tissue engineering.
Stem cells are defined as undifferentiated cells from the embryo, fetus or adult that have the unique potential to generate various differentiated tissue cells under appropriate biochemical, hormonal and mechanical stimuli in vitro and in vivo [1]. Adult stem cells in particular, represent a promising model for regenerative medicine and tissue engineering because the use of embryonic and fetal stem cells is limited by ethical considerations [2]. In contrast to embryonic and fetal stem cells, which are pluripotent, adult stem cells are multipotent, unspecialized cells that have been identified in various tissues and organs. They can serve as a multipotent reservoir to replenish specific tissue cells when they die [3].
Adult stem cells have been isolated from a variety of tissues and organs including peripheral blood [4], adipose tissue [5], central nervous system [6], muscle [7], and bone marrow [8]. Bone marrow hosts not only hematopoetic stem cells but it also contains a mesenchymal fibroblastic cell type that can differentiate into bone, fat and cartilage in vitro [9]. There are no mesenchymal stem cell (MSC)-specific cellular markers, therefore their identification is achieved through their ability to adhere to plastic in vitro, through their multilineage differentiation potential in vitro and through a combination of positive expression or distinct lack of defined cell surface markers [10]. These markers include CD105+, CD73+ and CD90+, whereas MSCs should lack CD45, CD34, and several other hematopoetic stem cell markers [10].
The correct balance between cell proliferation and differentiation is critical, especially for adult MSCs. First discovered and characterized by Friedenstein et al. [10], MSC are defined as adherent colony-forming unit fibroblasts (CFU-F) and self-renewing progenitor cells with a multilineage potential [11]. They are able to differentiate into a variety of cell types including, osteoblasts, chondrocytes and adipocytes and may be isolated from different sources [12].
The differentiation of MSCs in vitro largely depends on the culture conditions. Growth factors, such as the transforming growth factor-β family, result in chondrogenic differentiation [13]. Osteogenic differentiation of MSCs in vitro is induced by the presence of dexamethasone, ascorbic acid and β-glycerol phosphate [9], while MSCs cultured with dexamethasone, insulin, isobutyl methyl xanthine, and indomethacin will differentiate down an adipogenic lineage [14].
Current studies have mainly focused on the adipogenic potential of MSCs in vitro and in vivo [15]. However, the additional knowledge of differentiating and manipulating MSCs into various other tissues holds a promising key for cell based tissue repair and tissue engineering. Therefore, in order to assess the potential of MSCs in future clinical applications, it is essential to gain more insight into their differentiation capacity and evaluate the tissues formed by these cells at the morphological, immunophenotyping and gene expression levels.
In the present study, we investigated the morphological and immunophenotyping characteristics, furthermore monitoring in different time's intervals gene expression of the newly formed tissue by rat bone marrow derived MSCs induced towards adipogenic lineages.
Adult outbred Sprague Dawley (SD) rats that weighing 180 to 210 g were served as bone marrow donors. Bone marrow was obtained from the femurs and tibia of adult SD rats [16]. Rat bone marrow cells were cultured in basic media (Dulbecco's modified Eagle's medium [DMEM], Sigma Chemical Co., St. Louis, MO, USA) containing 10% fetal bovine serum (FBS; Sigma). The effluent was collected in sterile tubes. Gentle pipetting resulted in obtaining of a single cell suspension. Bone marrow cells were counted and plated with a concentration of 10×106/ml in T-75 flasks. The cells were then cultured in DMEM containing 10% FBS, penicillin (100 U/ml) and streptomycin (100 µg/ml) at 37℃ in a humidified atmosphere that contained 5% CO2. Medium was changed after 4 days and every 3 days thereafter. Nonadherent hematopoietic cells were removed when medium was changed. After a mean of 7 days, cells reached subconfluence and was detached with trypsin/EDTA, reseeded at 4×103 cells/cm2, and used for experiments after the third passage. MSC features were demonstrated by typical spindle-shaped morphology.
Fibroblast like colony growth was evaluated on primary cells grown on tissue culture six-well dishes [17]. Total bone marrow-derived cells were plated at the density of 25×106 cells/well. After 7 days, the capability of MSC to form fibroblast-like colonies was assessed. Images that showed MSC morphology were acquired by contrast-phase microscope.
The cells were centrifuged at 1,200 rpm for 5 minutes. and then solved in phosphate buffered saline (PBS) at the concentration of (1×106/ml). The cells were stained with different fluorescently labeled monoclonal antibodies (mAb). In brief, 100 µl of cell suspension was mixed with 10 µl of the fluorescently labeled mAb and incubated in the dark at room temperature for 30 minutes. Washing with PBS containing 2% bovine serum albumin was done twice and the pellet was resuspended in PBS and analyzed immediately on flow cytometry. The mAbs were used in different combinations of fluorochromes; namely fluorescein isothiocyanate, phycoerythrin and phycoerythrin-cyanine 5. Different combinations of mAb were used against various antigens. The immunophenotyping was performed on EPICS-XL flow cytometry (Coulter, Miami, FL, USA). The cells were analyzed with the most appropriate gate FBS using the combination of forward and side scatters.
Adipogenic differentiation was performed in monolayer culture in six-well-plates as described by Pittenger et al. [9]. Briefly, 20,000 cells were seeded per well and cultured until confluency. The cells were then treated for 72 hours with the adipogenic induction medium consisting of DMEM base medium, 10% FBS, penicillin (100 U/ml) and streptomycin (100 µg/ml), 1 µM dexamethasone (Sigma), 0.2 mM indomethacin (Sigma), 0.1 mg/ml insulin (Sigma), 1 mM 3-isobutyl-1-methylxanthin (Sigma) and afterwards for 24 hours with adipogenic maintenance medium consisting of DMEM base medium, 10% FBS, penicillin/streptomycin solution penicillin (100 U/ml) and streptomycin (100 µg/ml) and 0.1 mg/ml insulin. This cycle was repeated four times. Afterwards the cells were cultured for one more week in adipogenic maintenance medium. For the negative control cells were cultured in cell culture medium containing 10% FBS. Samples were taken from adipogenic differentiated stem cell at different time intervals, after 4, 7, 14, and 21 days for light microscopy and reverse transcription polymerase chain reaction (RT-PCR) study.
Cells were cultured in petri dishes for 4, 7, 14, and 21 days in DMEM plus 0.5 mM isobutylmethylxanthine, 1 µM dexamethasone, 10 µM insulin, and 200 µM indomethacin [9, 18]. Dishes were washed in PBS and cells were fixed in 3.7% formaldehyde for 30 minutes, followed by staining with oil-red O for 30 minutes oil-red O was prepared by diluting a stock solution (0.5 g of oil-red O [Sigma-Aldrich] in 100 ml of isopropanol) with water (6:4) followed by filtration. After staining, plates were washed twice in water and photographed [19]. Adipogenic differentiation were quantified by counting adipocytes in five randomly selected visual fields at magnification (×100) for each specimen (n=5) and calculating the percentage of adipogenic cells (number of cells with oil-red O positive intracellular vesicles/total number of cells).
Total RNA was extracted from adipogenic differentiated MSCs at 4, 7, 14, and 21 days by using RNeasy Mini kit (Qiagen, Valencia, CA, USA) and treated with deoxyribonuclease to remove contaminating genomic DNA, following the manufacturer's instructions. Reverse transcription was performed with 1 µg RNA in a total volume of 20 µl per reaction. In order to display adiponectin expression, we used primers forward 5'-GGGATTACTGCAACCGAAGG-3' and reverse 5'-CCATCCAACCTGCACAAGTTT-3'. For adipocyte lipid-binding protein (ALBP), primers were forward 5'-CATACATAAAGTCCTTCCCGCTG-3' and reverse 5'-TTGTCTGTTGTCTTTCCTGTCAAGA-3'. For leptin, primers were forward 5'-TTCACACACGCAGTCGGTATC-3' and reverse 5'-GTGAAGCCCGGGAATGAAG-3'. For lipoprotein lipase (LPL) forward primers were 5'-GTACAGTCTTGGAGCCCATGC-3' and reverse 5'-GCCAGTAATTCTATTGACCTTCTTGTT-3'. The RT-PCR procedure was performed using the One Step RT-PCR kit (Qiagen), beginning at 50℃ for 30 minutes and 95℃ for 15 minutes for reverse transcription, then followed by 35 cycles, with each cycle consisting of denaturion at 94℃ for 1 minute, annealing at 57℃ for 1 minute, elongation at 72℃ for 1 minute, and the final extension at 72℃ for 10 minutes. The amplified DNA fragments were visualized through 2% agarose gel electrophoreses and photographed under UV light [20]. In addition real-time PCR was performed with 25 µl amplification reactions contained primers at 0.5 µm, deoxynucleotide triphosphates (0.2 mm each) in PCR buffer, and 0.03 U Taq polymerase along with SYBR-green (Molecular Probes, Inc., Eugene, OR, USA) at 1:150,000. Aliquots of cDNA were diluted 5- to 5,000-fold to generate relative standard curves with which sample cDNA was compared. Standards and samples were run in triplicate, and use glyceraldehyde-3-phosphatedehydrogenase was also included as an internal control. PCR products from all species were normalized for the amount of 18S amplicons in the reverse transcription sample, which was standardized on a dilution curve. Reactions were performed on a 7000 Real-Time PCR System (ABI PRISM, Applied Biosystem, Foster City, CA, USA). A model introduced by Pfaffl was used for calculation [21, 22].
We harvested the MSCs from the donor rat bone marrow; purify them in culture media with different passages. MSCs were generated by stander procedures and grown for at least two passages in culture. Contaminating hematopoietic cells were depleted during passage 1 and MSCs were morphologically defined by a fibroblast-like appearance (Fig. 1A, B) and were able to form fibroblast like colonies. Colony formation was observed after 3-5 days in culture (Fig. 2). Cells actively searched for cell-to-cell contacts. After 6-7 days in culture, the colonies became confluent and were passaged for the first time.
Immunophenotypes of MSC surface markers was tested by flow cytometric analysis (Fig. 3). All isolated MSC were negative for CD45 and CD34 while they were positive for CD29, CD44, and CD90. These results as shown in Fig. 4 indicate that, the majority of the bone marrow derived cells were mesenchymal.
Adipogenic cells isolated from MSCs of rat bone marrow were shown to be able to proliferate in culture. During propagation, the cell morphology changed markedly, from spindle-shaped cells to flat ones. This morphological change was accompanied by a change in cell proliferation ability and lipid vesicles could be observed. Adipocyte differentiation from rat bone marrow was markedly flourished at 7 days post in vitro culturing then slightly increased. The MSCs derived earlier from rat bone marrow were firstly appeared dedifferentiated and spindle-shaped semi-like fibroblast cells. However, at 4 days, where adipocyte was still less differentiated, a population of both fibroblastoid and non-fibroblastoid cell types were identified. Fibroblastoid cell population was still existed after enzymatic digestion (Fig. 5A-C). Oil-red O staining of adipocyte revealed discrete spots of cytoplasmic oil droplete within adipocytes derived from rat bone marrow (Fig. 6).
Gene expression profiles throughout the differentiation process were examined in adipogenic differentiated genes at 4, 7, 14, and 21 days. Markers for the transcription factors for ALBP gene was expressed at 90 bp, however LPL, leptin, and adiponectin genes was expressed at 80 bp, 250 bp, and 85 bp respectively. Moreover, gel electrophoresis for rat mRNA expression of adiponectin at day 4 showed that, band was absent (Fig. 7). In addition to that, real time-PCR data for some genes in cells from adipogenic differentiated MSCs demonstrated that, at 4, 7, 14, and 21 days after differentiation, LPL gene expression was between 0.12% and 2.3% (Fig. 8), while ALBP gene expression was between 0.1% and 1.2% of rat adipocyte (Fig. 9).
As mentioned before in literature murine MSCs were obtained from the femurs and tibias of rat [16]. The present study demonstrated that after placing whole bone marrow cells in plastic culture dishes with medium bone marrow contains hematopoietic non-adherent cells (hematopoietic stem cells) along with a rare population of plastic-adherent cells (MSCs). After a few days, these adherent cells, of heterogeneous appearance, start to proliferate. The initial clones of adherent cells expanded into round-shaped colonies composed of fibroblastoid cells, thus the term of CFU-F. These results are consistent with those reported in many studies [8, 16].
The next point in the current study was to investigate cell-surface markers. De Macedo Braga et al. [23] and Ayatollahi et al. [24] demonstrated that rat bone marrow-MSCs express of cell-surface markers (CD markers) such as CD29, CD44, CD90, and CD106. In the contrary, Ayatollahi et al. [24] found that rat bone marrow-MSCs were negative for CD45 a cell-surface marker that typically identifies hematopoietic cells.
Our findings supported the work done by De Macedo Braga et al. [23] and Ayatollahi et al. [24]. Flow cytometry analysis data revealed that rat bone marrow-MSCs express of surface antigens (CD markers) such as CD29, CD44, and CD90 as markers for MSCs and lacking of the hematopoietic stem cell markers CD45 and CD34.
For developing cell-based tissue engineering and autologous connective tissue transplants, the most important requirement is gaining sufficient numbers of differentiated cells. The best candidate cells for this purpose are MSCs, because they are multipotent and have a high proliferative capacity. Because there is no definitive marker to identify MSCs, the gold standard procedure to prove their stem cell identity is 1) their adherence on cell culture plates after isolation, 2) their expression of specific markers, and 3) their differentiation potential to osteoblasts, adipocytes, and chondrocytes in vitro [14]. The first step to demonstrate the MSC character of our isolated rat bone marrow cells was therefore, to show that these MSCs express the putative MSC markers. Like other studies [25, 26] we could show this important MSC characteristic of our isolated stem cells.
Our study revealed that the rat bone marrow claimed that they possess stem cell potential, capable of differentiating into adipocyte cells. These represent the major source of multilineage of cells [27, 28, 29].
The MSCs derived earlier from rat bone marrow was firstly appeared dedifferentiated and spindle-shaped semi-like fibroblast cells. However, at 4 days, where adipocyte was still less differentiated, a population of both fibroblastoid and non-fibroblastoid cell types were identified. Fibroblastoid cell population was still existed after enzymatic digestion. Also, gene expression profiles throughout the differentiation process were examined in adipogenic differentiated genes at 4, 7, 14, and 21 days. Markers for the transcription factors for ALBP gene was expressed at 90 bp, however LPL, leptin, and adiponectin genes was expressed at 80 bp, 250 bp, and 85 bp respectively. Moreover, gel electrophoresis for rat mRNA expression of adiponectin at day 4 showed that, band was absent. In addition to that, real time-PCR data for some genes in cells from adipogenic differentiated MSCs demonstrated that, at 4, 7, 14, and 21 days after differentiation, LPL gene expression was between 0.12% and 2.3%, while ALBP gene expression was between 0.1% and 1.2% of rat adipocyte.
Adiponectin is an adipose-secreted protein that exerts both anti-atherogenic and insulin-sensitizing effects, and a reduced production of adiponectin is closely coupled to insulin resistance [30, 31]. The plasma concentration of adiponectin in obese subjects is lower than that in non-obese subjects and inversely correlates with body mass index [32]. Hypoadiponectinemia is associated with reduced endothelium-dependent dilatation in both diabetic and nondiabetic human subjects [33]. So the present findings suggest that adipose tissue can be an important therapeutic target in the protection of vascular dysfunction in diabetes by producing and releasing anti-inflammatory vasoactive hormones, among which adiponectin plays an indispensable role in protecting vascular function. Furthermore bone marrow MSCs may be a good candidate for use in tissue engineering research because they could be isolated in abundant quantities.
In conclusion, the data presented has demonstrated, for the first time, that MSCs possess multilineage potential and, when cultivated with the appropriate induction components, differentiate into highly specialized and functionally competent adipocytes. The resulting differentiated cells display structural features similar to resident cells in the original tissues as determined by light microscopy and gene expression (RT-PCR) techniques. A deeper insight into the differentiation potential of MSCs may help to promote the future use of MSCs in regenerative medicine, since this tissue engineering approach has the benefit of creating an alternative biomaterial to be used as connective tissue substitutes for cell-based autologous reconstructive surgery.
Figures and Tables
Fig. 1
(A, B) Cultured rat bone marrow mesenchymal stem cells at (P3) were morphologically defined by a fibroblast-like appearance. Scale bars=200 µm (A), 100 µm (B).
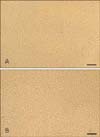
Fig. 2
Rat bone marrow mesenchymal stem cells after one week of culture grow in colonies (arrow). Scale bar=200 µm.
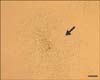
Fig. 3
Phenotypic characteristic of rat bone marrow mesenchymal stem cells, flow cytometry analysis revealed that their expression of surface antigens CD29, CD90, and CD44 (Passage 3) was strongly positive; while CD45 and CD34 was negative. BMSC, bone marrow stromal cell.
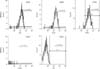
Fig. 4
Show the statistical analysis and mean value of flow cytometric surface markers for the undifferentiated mesenchymal stem cells. Each result represents the mean±SD of 5 replicates. Total CD29, CD44, and CD90 were significant at P<0.05.
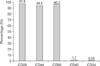
Fig. 5
Mesenchymal stem cells (MSC) showing differentiative potential at different time's intervals 7 days (A), 14 days (B), and 21 days (C). Adipocyte differentiation is visualized by highly refractive intracellular lipid vacuoles and droplets (arrows) appear as cherry red spheres within the cells and by oil-red O staining. Scale bars=50 µm (A-C).
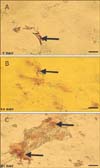
Fig. 6
Adipocyte differentiation from rat bone marrow (RBM). Each result represents the mean±SD of 5 replicates. Total mesenchymal stem cells (MSCs) and RBM significant at P<0.05.
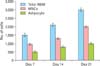
Fig. 7
Gel electrophoresis of adipogensis related gene for rat bone marrow mesenchymal stem cells (MSCs) at 4, 7, 14, and 21 days. Reverse transcriptase-polymerase chain reaction was performed for lipoprotein lipase (LPL), leptin, adipocytes lipid-binding protein (ALBP), adiponectin, and glyceraldehyde-3-phosphatedehydrogenase (GAPDH) reference gene.
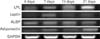
Fig. 8
Real-time polymerase chain reaction-based expression data normalized to the internal standard glyceraldehyde-3-phosphatedehydrogenase mRNA. Lipoprotein lipase (LPL) gene expression data at different times, 4, 7, 14, and 21 days from adipogenic differentiated mesenchymal stem cells. Data are mean±SD of triplicate independent experiments.
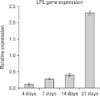
Fig. 9
Real-time polymerase chain reaction-based expression data normalized to the internal standard glyceraldehyde-3-phosphatedehydrogenase mRNA. Adipocytes lipid-binding protein (ALBP) gene expression data at different times, 4, 7, 14, and 21 days from adipogenic differentiated mesenchymal stem cells. Data are mean±SD of triplicate independent experiments.
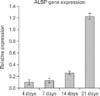
Acknowledgements
This work was supported by a grant from Science & Technology Development Fund (STDF) for basic and applied research grants in Egypt. Author is grateful for the help of manger (prof. Dr. Mohamed Abdel-kader Sobh) and all members of Medical and Experimental Research Center (MERC), Faculty of Medicine, Mansoura University, Egypt.
References
1. Caplan AI, Bruder SP. Mesenchymal stem cells: building blocks for molecular medicine in the 21st century. Trends Mol Med. 2001; 7:259–264.
2. Leo AJ, Grande DA. Mesenchymal stem cells in tissue engineering. Cells Tissues Organs. 2006; 183:112–122.
3. Caplan AI, Dennis JE. Mesenchymal stem cells as trophic mediators. J Cell Biochem. 2006; 98:1076–1084.
4. Till JE, McCulloch EA. Hemopoietic stem cell differentiation. Biochim Biophys Acta. 1980; 605:431–459.
5. Wickham MQ, Erickson GR, Gimble JM, Vail TP, Guilak F. Multipotent stromal cells derived from the infrapatellar fat pad of the knee. Clin Orthop Relat Res. 2003; (412):196–212.
6. Alexanian AR, Sieber-Blum M. Differentiating adult hippocampal stem cells into neural crest derivatives. Neuroscience. 2003; 118:1–5.
7. Williams JT, Southerland SS, Souza J, Calcutt AF, Cartledge RG. Cells isolated from adult human skeletal muscle capable of differentiating into multiple mesodermal phenotypes. Am Surg. 1999; 65:22–26.
8. Caplan AI. Mesenchymal stem cells. J Orthop Res. 1991; 9:641–650.
9. Pittenger MF, Mackay AM, Beck SC, Jaiswal RK, Douglas R, Mosca JD, Moorman MA, Simonetti DW, Craig S, Marshak DR. Multilineage potential of adult human mesenchymal stem cells. Science. 1999; 284:143–147.
10. Friedenstein AJ, Petrakova KV, Kurolesova AI, Frolova GP. Heterotopic of bone marrow. Analysis of precursor cells for osteogenic and hematopoietic tissues. Transplantation. 1968; 6:230–247.
11. Stockl S, Bauer RJ, Bosserhoff AK, Gottl C, Grifka J, Grassel S. Sox9 modulates cell survival and adipogenic differentiation of multipotent adult rat mesenchymal stem cells. J Cell Sci. 2013; 126:2890–2902.
12. Mackay AM, Beck SC, Murphy JM, Barry FP, Chichester CO, Pittenger MF. Chondrogenic differentiation of cultured human mesenchymal stem cells from marrow. Tissue Eng. 1998; 4:415–428.
13. Jaiswal N, Haynesworth SE, Caplan AI, Bruder SP. Osteogenic differentiation of purified, culture-expanded human mesenchymal stem cells in vitro. J Cell Biochem. 1997; 64:295–312.
14. Dominici M, Le Blanc K, Mueller I, Slaper-Cortenbach I, Marini F, Krause D, Deans R, Keating A, Prockop D, Horwitz E. Minimal criteria for defining multipotent mesenchymal stromal cells. The International Society for Cellular Therapy position statement. Cytotherapy. 2006; 8:315–317.
15. Kadiyala S, Young RG, Thiede MA, Bruder SP. Culture expanded canine mesenchymal stem cells possess osteochondrogenic potential in vivo and in vitro. Cell Transplant. 1997; 6:125–134.
16. Lennon DP, Caplan AI. Isolation of rat marrow-derived mesenchymal stem cells. Exp Hematol. 2006; 34:1606–1607.
17. Phinney DG, Kopen G, Isaacson RL, Prockop DJ. Plastic adherent stromal cells from the bone marrow of commonly used strains of inbred mice: variations in yield, growth, and differentiation. J Cell Biochem. 1999; 72:570–585.
18. Green H, Meuth M. An established pre-adipose cell line and its differentiation in culture. Cell. 1974; 3:127–133.
19. Hauner H, Schmid P, Pfeiffer EF. Glucocorticoids and insulin promote the differentiation of human adipocyte precursor cells into fat cells. J Clin Endocrinol Metab. 1987; 64:832–835.
20. Peng HH, Wang TH, Chao AS, Chang SD. Isolation and differentiation of human mesenchymal stem cells obtained from second trimester amniotic fluid; experiments at Chang Gung Memorial Hospital. Chang Gung Med J. 2007; 30:402–407.
21. Rubin J, Murphy TC, Fan X, Goldschmidt M, Taylor WR. Activation of extracellular signal-regulated kinase is involved in mechanical strain inhibition of RANKL expression in bone stromal cells. J Bone Miner Res. 2002; 17:1452–1460.
22. Pfaffl MW. A new mathematical model for relative quantification in real-time RT-PCR. Nucleic Acids Res. 2001; 29:e45.
23. de Macedo Braga LM, Lacchini S, Schaan BD, Rodrigues B, Rosa K, De Angelis K, Borges LF, Irigoyen MC, Nardi NB. In situ delivery of bone marrow cells and mesenchymal stem cells improves cardiovascular function in hypertensive rats submitted to myocardial infarction. J Biomed Sci. 2008; 15:365–374.
24. Ayatollahi M, Salmani MK, Geramizadeh B, Tabei SZ, Soleimani M, Sanati MH. Conditions to improve expansion of human mesenchymal stem cells based on rat samples. World J Stem Cells. 2012; 4:1–8.
25. Mageed AS, Pietryga DW, DeHeer DH, West RA. Isolation of large numbers of mesenchymal stem cells from the washings of bone marrow collection bags: characterization of fresh mesenchymal stem cells. Transplantation. 2007; 83:1019–1026.
26. Mareddy S, Crawford R, Brooke G, Xiao Y. Clonal isolation and characterization of bone marrow stromal cells from patients with osteoarthritis. Tissue Eng. 2007; 13:819–829.
27. Bayati V, Hashemitabar M, Gazor R, Nejatbakhsh R, Bijannejad D. Expression of surface markers and myogenic potential of rat bone marrow- and adipose-derived stem cells: a comparative study. Anat Cell Biol. 2013; 46:113–121.
28. Jin HJ, Bae YK, Kim M, Kwon SJ, Jeon HB, Choi SJ, Kim SW, Yang YS, Oh W, Chang JW. Comparative analysis of human mesenchymal stem cells from bone marrow, adipose tissue, and umbilical cord blood as sources of cell therapy. Int J Mol Sci. 2013; 14:17986–18001.
29. Gimble JM, Katz AJ, Bunnell BA. Adipose-derived stem cells for regenerative medicine. Circ Res. 2007; 100:1249–1260.
30. Kadowaki T, Yamauchi T, Kubota N, Hara K, Ueki K, Tobe K. Adiponectin and adiponectin receptors in insulin resistance, diabetes, and the metabolic syndrome. J Clin Invest. 2006; 116:1784–1792.
31. Zhu W, Cheng KK, Vanhoutte PM, Lam KS, Xu A. Vascular effects of adiponectin: molecular mechanisms and potential therapeutic intervention. Clin Sci (Lond). 2008; 114:361–374.
32. Arita Y, Kihara S, Ouchi N, Takahashi M, Maeda K, Miyagawa J, Hotta K, Shimomura I, Nakamura T, Miyaoka K, Kuriyama H, Nishida M, Yamashita S, Okubo K, Matsubara K, Muraguchi M, Ohmoto Y, Funahashi T, Matsuzawa Y. Paradoxical decrease of an adipose-specific protein, adiponectin, in obesity. Biochem Biophys Res Commun. 1999; 257:79–83.
33. Torigoe M, Matsui H, Ogawa Y, Murakami H, Murakami R, Cheng XW, Numaguchi Y, Murohara T, Okumura K. Impact of the high-molecular-weight form of adiponectin on endothelial function in healthy young men. Clin Endocrinol (Oxf). 2007; 67:276–281.