Abstract
Peroxisome proliferator-activated receptor γ (PPARγ) has various actions including the regulation of adipocyte differentiation, lipid metabolism and glucose homeostasis. In the present study, we examined the changes of PPARγ immunoreactivity and protein levels in the gerbil dentate gyrus (DG) after transient global cerebral ischemia using immunohistochemistry and western blot analysis. PPARγ immunoreactivity was gradually increased from 1 day after ischemia-reperfusion. PPARγ immunoreactivity, in accordance with protein level, was highest at 2 days after ischemia-reperfusion and was detected in microglia at this time. Thereafter, both PPARγ immunoreactivity and protein level were decreased with time in the ischemic DG. These results indicate that PPARγ may be related to the ischemia-induced microglial activation and neuronal damage/death in the DG after transient global cerebral ischemia.
Transient global cerebral ischemia results in the insidious degeneration of specific vulnerable neurons in the brain. Especially, the hippocampus has been known to be one of the most vulnerable regions after ischemic damage [1, 2]. In the hippocampus, the cornu ammonis 1 (CA1) region is the most susceptible to transient cerebral ischemia, and neuronal death in the pyramidal cell layer of the hippocampal CA1 region has been described as "delayed neuronal death", whereas the hippocampal dentate gyrus (DG) is known to tolerate ischemic insult relatively [1, 2]. Our previous study showed that neuronal degeneration in the DG occurred earlier than the delayed neuronal death in the CA1 region following transient cerebral ischemia [3]. However, few studies are interested in the ischemia-induced changes in the DG, compared with the CA1 region, following transient cerebral ischemia.
Peroxisome proliferator-activated receptor gamma (PPARγ) has multiple actions, such as the regulation of adipocyte differentiation, insulin sensitivity, lipid metabolism and glucose homeostasis [4, 5, 6]. PPARγ is detected mainly in adipocytes, smooth muscles and macrophages, and it is expressed in neurons and glial cells throughout the brain and spinal cord [6, 7, 8, 9, 10]. In addition, many studies have reported the neuroprotective effect of PPARγ agonist in various models of neurodegenerative disorders and brain ischemia [11, 12, 13, 14, 15, 16, 17, 18]. Especially, neuroprotective effect of PPARγ agonists in brain ischemia is known to be closely associated with their anti-inflammatory activity [14, 16].
Recently, we reported the changes in PPARγ expression and the neuroprotective effect of PPARγ agonist, rosiglitazone, against ischemic damage in the gerbil hippocampal CA1 region following 5 minutes of transient cerebral ischemia [14]. However, there is no studies regarding changes in PPARγ expression in the ischemic DG, even though the ischemia-induced neuronal degeneration is represented differently in the hippocampal CA1 region and DG [3]. In the present study, therefore, we examined the ischemia-induced changes of PPARγ immunoreactivity and protein levels in the gerbil DG after 5 minutes of transient global cerebral ischemia.
Male Mongolian gerbils (Meriones unguiculatus) were obtained from the Experimental Animal Center, Kangwon National University, Chuncheon, South Korea. Gerbils were used at 6 months (B.W., 65-75 g) of age. The animals were housed in a conventional state under adequate temperature (23℃) and humidity (60%) control, with a 12-hours light/dark cycle, and free access to water and food. The procedures for animal handling and care adhered to guidelines that are in compliance with the current international laws and policies (Guide for the Care and Use of Laboratory Animals, The National Academies Press, 8th edition, 2011), and they were approved by the Institutional Animal Care and Use Committee (IACUC) at Kangwon University. All experiments were conducted to minimize the number of animals used and the suffering caused by the procedures used in the present study.
Mongolian gerbils underwent transient cerebral ischemia by the method of our previous study [3, 14]. Briefly, the animals were anesthetized with a mixture of 2.5% isoflurane in 33% oxygen and 67% nitrous oxide. Bilateral common carotid arteries were occluded for 5 min using non-traumatic aneurysm clips. The body (rectal) temperature under free-regulating or normothermic (37±0.5℃) conditions was monitored with a rectal temperature probe (TR-100, Fine Science Tools, Foster City, CA, USA) and maintained using a thermometric blanket before, during and after the surgery until the animals completely recovered from anesthesia. Sham-operated animals were subjected to the same surgical procedures except that the common carotid arteries were not occluded.
For the histological analysis, the sham- and ischemia-operated-groups (n=7 at each time point) were used at 6 hours, 12 hours, 1 day, 2 days, 4 days, 7 days, and 10 days after ischemia-reperfusion. The animals were anesthetized with sodium pentobarbital and perfused transcardially with 0.1 M phosphate-buffered saline (PBS, pH 7.4) followed by 4% paraformaldehyde in 0.1 M phosphate-buffer (pH 7.4). The brain tissues were removed, cryoprotected and serially sectioned on a cryostat (Leica, Wetzlar, Germany) into 30 µm coronal sections, and they were then collected into six-well plates containing PBS.
According to the method of our previous study [3, 14], immunohistochemical staining for PPARγ was performed using mouse anti-PPARγ (1:100, Santa Cruz Biotechnology, Santa Cruz, CA, USA), biotinylated goat anti-mouse IgG (1:200, Vector, Burlingame, CA, USA) and streptavidin peroxidase complex (1:200, Vector). A negative control test was carried out using pre-immune serum instead of primary antibody in order to establish the specificity of the immunostaining. The negative control resulted in the absence of immunoreactivity in all structures.
Eight sections per animal were selected to quantitatively analyze PPARγ immunoreactivity in the DG. Digital images were captured with an AxioM1 light microscope (Carl Zeiss, Göttingen, Germany) equipped with a digital camera (Axiocam, Carl Zeiss) connected to a PC monitor. According to the method of the previous study [14], semi-quantification of the immunostaining intensities was evaluated with digital image analysis software (MetaMorph 4.01, Universal Imaging Corp., Downingtown, PA, USA). The level of immunoreactivity was scaled as -, ±, + or ++, representing no staining (gray scale value, ≥200), weakly positive (gray scale value, 150-199), moderate (gray scale value, 100-149), or strong (gray scale value, ≤99), respectively.
To examine the cell type expressing PPARγ immunoreactivity, the sections after the ischemic surgery were processed by double immunofluorescence staining. Double immunofluorescence staining was performed using mouse anti-PPARγ (1:50, Santa Cruz Biotechnology)/rabbit anti-Iba-1 (1:200, Wako, Nuss, Germany) for microglia or rabbit anti-glial fibrillary acidic protein (GFAP; 1:200, Chemicon International, Temecula, CA, USA) for astrocytes. The sections were incubated in the mixture of antisera overnight at room temperature, and then were incubated in a mixture of both Cy3-conjugated goat anti-mouse IgG (1:200, Jackson ImmunoResearch, West Grove, PA, USA) and FITC-conjugated goat anti-rabbit IgG (1:200, Jackson ImmunoResearch) for 2 hours at room temperature. The immunoreactions were observed under the confocal microscope (LSM510 META NLO, Carl Zeiss).
To examine changes in PPARγ protein levels in the DG after transient cerebral ischemia, sham- and ischemia-operated animals (n=5 at each time point) were used for western blot analysis according to the method of our previous study [14]. In brief, after removing the brains, they were serially and transversely cut into a thickness of 400 µm on a vibratome (Leica), and the DG was then dissected with a surgical blade. After the tissues were homogenized and centrifuged, the supernatants were subjected to western blot analysis. Mouse anti-PPARγ (1:500, Santa Cruz Biotechnology) or mouse anti-β-actin (1:5,000, Sigma, St. Louis, MO, USA) was used as primary antibody. Western blot analysis was performed triplicate experiments and representative gel is shown. The result of the western blot analysis was scanned, and densitometric analysis for the quantification of the bands was done using Scion Image software (Scion Corp., Frederick, MD, USA), which was used to count relative optical density (ROD). A ratio of the ROD was calibrated as %, with sham-operated group designated as 100%.
The data shown here represent the means±SEM. Differences of the means among the groups were statistically analyzed by analysis of variance (ANOVA) with a post hoc Bonferroni's multiple comparison test in order to elucidate ischemia-related differences among experimental groups (SigmaStat, Systat Software, San Jose, CA, USA). Statistical significance was considered at P<0.05.
In the sham-operated group, PPARγ immunoreactive cells were not detected in any layers of the DG; weak immunoreactivity was found in the neuropil of the molecular and polymorphic layers, not the granule cell layer (Table 1, Fig. 1A). The pattern of PPARγ immunoreactivity was not changed until 12 hours after ischemia-reperfusion (Table 1). PPARγ immunoreactivity began to be shown in the DG from 1 day after ischemia-reperfusion, and PPARγ immunoreactivity was highest at 2 days after ischemia/reperfusion, especially in the polymorphic layer (Table 1, Fig. 2B, C). Thereafter, PPARγ immunoreactivity was decreased with time, and the pattern of PPARγ immunoreactivity in the ischemic DG at 10 days after ischemia-reperfusion was similar to that in the sham-operated group (Table 1, Fig. 2D-F).
Two days after ischemia-reperfusion, many PPARγ immunoreactive cells were well detected in the DG (Fig. 1C). Based on this result, we performed double immunofluorescence staining for PPARγ/Iba-1 or PPARγ/GFAP in the DG at 2 days after ischemia-reperfusion to identify the cell type. Most of all PPARγ immunoreactive cells were colocalized with Iba-1-immunoreactive microglia (Fig. 2). However, PPARγ immunoreactive cells were not colocalized with GFAP-immunoreactive astrocytes (data not shown).
From western blot analysis, we observed that the pattern of changes in PPARγ protein levels in the DG after ischemia-reperfusion was similar to that observed in the immunohistochemical data. PPARγ protein level was very low in the sham-operated gerbils. However, PPARγ protein level was significantly increased at 2 days after ischemia-reperfusion and was distinctively decreased at 4 days after ischemia-reperfusion (Fig. 3).
In the present study, we examined changes in PPARγ immunoreactivity and protein level in the gerbil DG after ischemia-reperfusion. In the sham-operated group, we did not find any PPARγ immunoreactive cells; very weak PPARγ protein level was found in the DG. This result was very similar with the finding that showed that PPARγ immunoreactive cells were not found in the hippocampal CA1 region of the sham-operated group [14]. In addition, it was reported that PPARγ immunoreactivity was found in the rat DG [9]. We also observed that PPARγ immunoreactivity was increased gradually from 1 day after ischemia-reperfusion and that PPARγ immunoreactivity and protein level were highest at 2 days after ischemia-reperfusion and they were decreased with time in the ischemic DG. Recently, it was reported that PPARγ immunoreactivity and protein level were significantly increased in the gerbil hippocampal CA1 region at 4 days after ischemia-reperfusion, which was the time that ischemia-induced delayed neuronal death occurred [14]. It was also reported that PPARγ immunoreactivity was significantly increased in the peri-infarct areas of the cerebral cortex 12 hours after middle cerebral artery occlusion in the rat and the immunoreactivity returned to basal level and remained unchanged [18]. In addition, a recent study showed that both PPARγ protein and mRNA expression were apparently increased 24 hours after ischemia-reperfusion and decreased with time in the rat hippocampus induced by global cerebral ischemia-reperfusion [19].
Ischemia-induced microglial activation is related to neuronal damage/death through the secretion of neurotoxic molecules [20, 21]. There are many reports regarding the relationship between PPARγ and microglia/macrophage. PPARγ has been well known as a negative regulator of macrophage activation through the inhibition of proinflammatory genes [6]. In addition, it was reported that PPARγ expression in microglia was dependent on microglial functional state and that PPARγ in microglia promoted phagocytosis and modulated neuroinflammation [7, 12, 22]. In the present study, we observed that PPARγ immunoreactive cells showed the highest immunoreactivity and they were colocalized with microglia, not astrocytes, in the ischemic DG at 2 days after ischemia-reperfusion. This finding is supported by our previous report that showed that PPARγ immunoreactivity was highest in activated microglia in the gerbil hippocampal CA1 region at 4 days after transient cerebral ischemia [14]. Therefore, it can be postulated that PPARγ in microglia may be related to ischemia-induced microglial activation as well as neuronal degeneration, and this reflects a change in microenvironment in the ischemic DG.
In conclusion, PPARγ immunoreactivity were expressed in microglia in the DG after transient global cerebral ischemia and the immunoreactivity was highest at 2 days post-ischemia. These results indicate that PPARγ may be related to ischemia-induced microglial activation and neuronal degeneration in the ischemic DG.
Figures and Tables
Fig. 1
Immunohistochemical staining for peroxisome proliferator-activated receptor gamma (PPARγ) in the dentate gyrus (DG) of the sham- (A) and ischemia-operated- (B-F) groups. Weak PPARγ immunoreactivity is detected in the neuropil of the molecular (ML) and polymorphic (PL) layers of the sham-operated group; the granule cell layer (GCL, asterisks) does not show any PPARγ immunoreactivity. In the ischemia-operated groups, many cells in the PL show strong PPARγ immunoreactivity (arrows) at 2 days after ischemia-reperfusion. Thereafter, PPARγ immunoreactivity is decreased with time. Scale bar=100 µm.
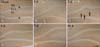
Fig. 2
Double immunofluorescence staining for peroxisome proliferator-activated receptor gamma (PPARγ) (red, A, D), Iba-1 (green, B)/glial fibrillary acidic protein (GFAP) (E) and merged images (C, F) in the dentate gyrus 2 days after ischemia-reperfusion. PPARγ immunoreactive cells are colocalized with Iba-1 immunoreactive microglia (arrows). ML, molecular layer; GCL, granule cell layer; PL, polymorphic layer. Scale bar=100 µm.
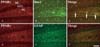
Fig. 3
Western blot analysis of peroxisome proliferator-activated receptor gamma (PPARγ) (57 kDa) in the dentate gyrus (DG) derived from the sham- and ischemia-operated groups. The relative optical density of the immunoblot band is represented as % values (*P<0.05, significantly different from the sham-operated group). The bars indicate the means±SEM. Isch, ischemia.
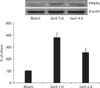
Acknowledgements
This research was supported by Basic Science Research Program through the National Research Foundation of Korea (NRF) funded by the Ministry of Science, ICT & Future Planning (NRF-2012R1A1A1007298).
References
1. Kirino T. Delayed neuronal death in the gerbil hippocampus following ischemia. Brain Res. 1982; 239:57–69.
2. Kirino T, Sano K. Selective vulnerability in the gerbil hippocampus following transient ischemia. Acta Neuropathol. 1984; 62:201–208.
3. Moon JB, Lee CH, Park CW, Cho JH, Hwang IK, Yoo KY, Choi JH, Shin HC, Won MH. Neuronal degeneration and microglial activation in the ischemic dentate gyrus of the gerbil. J Vet Med Sci. 2009; 71:1381–1386.
4. Berger JP, Akiyama TE, Meinke PT. PPARs: therapeutic targets for metabolic disease. Trends Pharmacol Sci. 2005; 26:244–251.
5. Chinetti G, Fruchart JC, Staels B. Peroxisome proliferator-activated receptors (PPARs): nuclear receptors at the crossroads between lipid metabolism and inflammation. Inflamm Res. 2000; 49:497–505.
6. Ricote M, Li AC, Willson TM, Kelly CJ, Glass CK. The peroxisome proliferator-activated receptor-gamma is a negative regulator of macrophage activation. Nature. 1998; 391:79–82.
7. Bernardo A, Levi G, Minghetti L. Role of the peroxisome proliferator-activated receptor-gamma (PPAR-gamma) and its natural ligand 15-deoxy-Delta12, 14-prostaglandin J2 in the regulation of microglial functions. Eur J Neurosci. 2000; 12:2215–2223.
8. Braissant O, Foufelle F, Scotto C, Dauça M, Wahli W. Differential expression of peroxisome proliferator-activated receptors (PPARs): tissue distribution of PPAR-alpha, -beta, and -gamma in the adult rat. Endocrinology. 1996; 137:354–366.
9. Moreno S, Farioli-Vecchioli S, Cerù MP. Immunolocalization of peroxisome proliferator-activated receptors and retinoid X receptors in the adult rat CNS. Neuroscience. 2004; 123:131–145.
10. Zhu Y, Alvares K, Huang Q, Rao MS, Reddy JK. Cloning of a new member of the peroxisome proliferator-activated receptor gene family from mouse liver. J Biol Chem. 1993; 268:26817–26820.
11. Breidert T, Callebert J, Heneka MT, Landreth G, Launay JM, Hirsch EC. Protective action of the peroxisome proliferator-activated receptor-gamma agonist pioglitazone in a mouse model of Parkinson's disease. J Neurochem. 2002; 82:615–624.
12. Combs CK, Johnson DE, Karlo JC, Cannady SB, Landreth GE. Inflammatory mechanisms in Alzheimer's disease: inhibition of beta-amyloid-stimulated proinflammatory responses and neurotoxicity by PPARgamma agonists. J Neurosci. 2000; 20:558–567.
13. Inestrosa NC, Godoy JA, Quintanilla RA, Koenig CS, Bronfman M. Peroxisome proliferator-activated receptor gamma is expressed in hippocampal neurons and its activation prevents beta-amyloid neurodegeneration: role of Wnt signaling. Exp Cell Res. 2005; 304:91–104.
14. Lee CH, Park OK, Yoo KY, Byun K, Lee B, Choi JH, Hwang IK, Kim YM, Won MH. The role of peroxisome proliferator-activated receptor gamma, and effects of its agonist, rosiglitazone, on transient cerebral ischemic damage. J Neurol Sci. 2011; 300:120–129.
15. Luo Y, Yin W, Signore AP, Zhang F, Hong Z, Wang S, Graham SH, Chen J. Neuroprotection against focal ischemic brain injury by the peroxisome proliferator-activated receptor-gamma agonist rosiglitazone. J Neurochem. 2006; 97:435–448.
16. Sundararajan S, Gamboa JL, Victor NA, Wanderi EW, Lust WD, Landreth GE. Peroxisome proliferator-activated receptor-gamma ligands reduce inflammation and infarction size in transient focal ischemia. Neuroscience. 2005; 130:685–696.
17. Xu F, Li J, Ni W, Shen YW, Zhang XP. Peroxisome proliferator-activated receptor-gamma agonist 15d-prostaglandin J2 mediates neuronal autophagy after cerebral ischemia-reperfusion injury. PLoS One. 2013; 8:e55080.
18. Zhao Y, Patzer A, Herdegen T, Gohlke P, Culman J. Activation of cerebral peroxisome proliferator-activated receptors gamma promotes neuroprotection by attenuation of neuronal cyclooxygenase-2 overexpression after focal cerebral ischemia in rats. FASEB J. 2006; 20:1162–1175.
19. Wang H, Jiang R, He Q, Zhang Y, Zhang Y, Li Y, Zhuang R, Luo Y, Li Y, Wan J, Tang Y, Yu H, Jiang Q, Yang J. Expression pattern of peroxisome proliferator-activated receptors in rat hippocampus following cerebral ischemia and reperfusion injury. PPAR Res. 2012; 2012:596394.
20. Lee JM, Grabb MC, Zipfel GJ, Choi DW. Brain tissue responses to ischemia. J Clin Invest. 2000; 106:723–731.
21. Lees GJ. The possible contribution of microglia and macrophages to delayed neuronal death after ischemia. J Neurol Sci. 1993; 114:119–122.
22. Zhao X, Sun G, Zhang J, Strong R, Song W, Gonzales N, Grotta JC, Aronowski J. Hematoma resolution as a target for intracerebral hemorrhage treatment: role for peroxisome proliferator-activated receptor gamma in microglia/macrophages. Ann Neurol. 2007; 61:352–362.