Abstract
We examined a series of changes that occur in the trabecular meshwork fibers of human eyes during fetal development at 12-30 weeks of gestation. At 12 and 15 weeks, the uveal meshwork was stained black with silver impregnation (indicating the predominance of collagen types III and IV) in the endomysium of the ciliary muscle. At 20 weeks, in combination with Schlemm's canal, a dense fibrous tissue mass corresponding to the trabecular meshwork anlage appeared and was colored black. The anlage was continuous with the corneal endothelium rather than with the ciliary muscle. Until 25 weeks, the trabecular meshwork was identifiable as fragmented fiber bundles that stained red-black, suggesting a mixture of collagen types I, III, and IV. At 30 weeks, half of the ciliary muscle fibers were inserted into the scleral spur and not into the meshwork. Therefore, any contribution of ciliary muscle contraction to the differentiation of the trabecular meshwork would appear to be limited. We hypothesize that an uneven distribution of mechanical stresses in the area of the cornea-sclera junction causes a tear thereby creating Schlemm's canal and is accompanied by a change in the collagen fiber types comprising the meshwork.
There have been numerous morphological studies on the human fetal trabecular meshwork (TM) at the iridocorneal angle. Using fetal eyes at 12-22 weeks of gestation, McMenamin [1-3] conducted a series of elegant studies using scanning electron microscopy (SEM) and obtained comprehensive data on the morphological changes that occur during TM development. Those studies demonstrated that the uvea-derived trabecular endothelial cells were distinguishable from the corneal endothelium at 12-13 weeks. McMenamin [1, 2] appeared to focus especially on the issue of whether the TM endothelium is continuous or interrupted, and this issue has since been pursued by many research groups. Very recently, VanderWyst et al. [4] demonstrated different types of collagen and other related substances such as integrin on and in the TM cell membrane in human adults. Another important contribution of McMenamin [1] was the methods that he employed to study the development of the iridocorneal angle: he demonstrated that tissue cleavage occurring as an artifact during histological procedures [5, 6] was easily identifiable as a rough surface in SEM observations.
Anderson [7] presented a clear diagram showing posterior "sliding" (a relative topographical change) of the iridocorneal angle or lateral expansion of the anterior chamber due to more rapid growth of the cornea and sclera relative to the uvea after 20 weeks of gestation; the TM acquires a position anterior to the ciliary muscle from this point onward. He hypothesized that the posterior mechanical traction from the iris root is fundamental to TM development. Anderson's scheme [7] is simple, but it appears to be a great improvement over the scenario proposed by Hansson and Jerndal [8], whose diagram is detailed but does not demonstrate the relative topographical changes that occur between the angle and the ciliary muscle. Using SEM observations of human fetal eyes (8-36 weeks of gestation), Ruano-Gil et al. [9] also hypothesized that mechanical forces induced TM differentiation with the aid of tensile stress generated by the ciliary muscle at 25 weeks of gestation. They confirmed the connection between the ciliary muscle and the TM, which had been ruled out by other researchers [10, 11]. According to Ruano-Gil et al. [9], the ciliary muscle insertions are divided into 3 layers: 1) an outer layer corresponding to the scleral spur, 2) a middle layer corresponding to Schlemm's canal, and 3) an inner layer corresponding to Descemet's membrane of the cornea.
Previous research does not provide overall views showing the series of changes in fetal morphology, i.e., lower-magnification views of the fetal TM and surrounding areas at various stages. However, a limited example was presented by Meghpara et al. [12], who conducted studies of cell death at the iridocorneal angle and provided lower-magnification views of the area at 7-27 weeks of gestation and at 5 years after birth. Although their sections were stained simply with hematoxylin and eosin (H&E), the overall views that included the angle, TM, and ciliary muscle together provided critical information about the structures that were dense or loose at the same point of fetal development. Such information is important to discuss the mechanical stresses that are hypothesized to occur in and around the fetal TM. Similarly, previous research has provided no information about the types of collagen fibers that are present in human fetuses. Collagen types would be expected to change according to mechanical stresses in the local environment (reviewed by Marshall et al. [13]), but immunostaining of collagen in human fetuses is difficult because of poor preservation and fixation in the period immediately prior to and during abortion. Instead, using silver staining (silver impregnation), we recently discriminated collagen types III- and IV-predominant fibrous tissue from the usual collagen type I-predominant connective tissue [14-16]. However, few studies seem to have applied silver staining to demonstrate the fiber configuration at the iridocorneal angle, with the apparent exception of Speakman [17], who studied the shape of TM endothelial cells using this approach.
Accordingly, in this study, we used silver staining with the aim of acquiring a thorough knowledge of changes in collagen fiber types during human fetal development in the tissues surrounding the TM, as well as the TM itself, to help identify the mechanical stresses that play a role in this process and, if possible, the extent of their contribution.
This study was performed in accordance with the provisions of the Declaration of Helsinki 1995 (as revised in Edinburgh 2000). We examined the paraffin-embedded histology of 20 mid-term fetuses with an estimated gestational age of 12-30 weeks (crown-rump length, 80-250 mm). For horizontal sections, 4 fetuses each were used at the ages of 12, 15, 20, 25, and 30 weeks. The thickness of the sections was 5 µm (fetuses earlier than 15 weeks) or 10 µm (fetuses later than 20 weeks), at intervals of 50 µm (early stage) or 100 µm (late stage), and stained with H&E or silver impregnation according to Lillie et al. [18]. This staining is usually called "Gitter (reticular network) staining" and differs from both the well-known Gomori method [19] and periodic acid methenamine silver staining [20]. Some of the sections were used for immunohistochemistry with monoclonal anti-human alpha-1 smooth muscle actin (1:100, Dako, Glostrup, Denmark) as the primary antibody. The secondary antibody (Dako Chem Mate Envison Kit, Dako) was labeled with horseradish peroxidase (HRP), and antigen-antibody reactions were detected via an HRP-catalyzed reaction with diaminobenzidine. Counterstaining with hematoxylin was performed on the same samples.
We chose specimens that did not show obvious deformation of the eyeball, such as shrinkage and irregularity of shape, resulting from histological procedures. All of the specimens were part of the large collection kept at the Embryology Institute of Universidad Complutense, Madrid. The specimens were obtained from women who suffered miscarriages and ectopic pregnancies at the Department of Obstetrics of the University. Although we were unable to rule out the presence of pathology because of the nature of the specimens, our aim was to describe the morphology that was commonly evident in fetuses at each stage. Approval for the study was granted by the Ethics Committee of the University (B-08/374 for the second author, J.F.R-V.).
At 12 and 15 weeks, the sclera was composed of thin fibers that were colored red with silver staining (i.e., type I collagen-predominant), whereas Descemet's membrane was stained black (i.e., collagen types III and IV) (Fig. 1). The ciliary muscle showed immunoreactivity for smooth muscle actin, while the muscle was identified as a bundle of black fibers upon silver staining because of the endomysium in combination with the basement membrane of smooth muscle fibers (i.e., types III and IV collagen-predominant) [21]. The immunoreactive smooth muscle fibers reached the sclera but did not approach the iris. In 4 fetuses at 12 weeks, the iridocorneal angle was 0.5-0.8 mm from the ciliary muscle (Fig. 1A, B). Likewise, in 4 fetuses at 15 weeks, the angle was 0.3-0.5 mm from the muscle (Fig. 1C, D). Loose mesenchymal tissue, or the so-called uveal meshwork [1, 2, 22], occupied an area between the muscle and the angle and was at the base of the iris. However, small spaces appeared in this space near the angle at 15 weeks, and veins developed along the outer margin of the cornea-sclera junction area.
In 4 fetuses at 20 weeks, the iridocorneal angle reached a site within 0.2 mm of the ciliary muscle (Fig. 2). Relatively dense fibrous tissue, possibly corresponding to a presumptive anlage of the TM, appeared in the corneal side of the loose mesenchymal tissue between the muscle and the angle (Fig. 2A shows a form more primitive or earlier than that shown in Fig. 2B). The maximum thickness of the TM anlage was 0.05-0.21 mm. The composite fibers were colored black or displayed a color similar to that of the ciliary muscle. The corneal endothelium was continuous with the suggested TM anlage (Fig. 2B), and Schlemm's canal was present immediately adjacent to the outer side of the latter (Fig. 2A, B). No connecting fiber was evident between the TM anlage and the ciliary muscle. Immunostaining for smooth muscle actin identified the ciliary muscle (Fig. 2C). The anterior chamber reached or faced the suggested anlage of the TM (Fig. 2B). The collagen fibers formed thick bundles in the cornea and sclera. No irregularity was evident along the global aspects of the cornea and sclera because of the absence of the scleral spur. In the area of the cornea-sclera junction near the ciliary muscle, the fiber bundle was fragmented, and, in one fetus (Fig. 2B), a vein ran through and interrupted the collagen fiber bundles.
In 8 fetuses at 25 and 30 weeks (Fig. 3), the scleral spur became evident, and half of the ciliary muscle fibers were inserted into the red-colored fibers of the spur (i.e., type I collagen-predominant). The other half of the muscle fibers were continuous with thin tendons that were dispersed in the loose uveal meshwork near the iridocorneal angle. The ciliary muscle contained numerous capillaries, but these were identified as membranous structures (Fig. 3A) or black dots (Fig. 3B) depending on whether the fixation of the materials was good or poor, respectively. Immediately on the corneal side of the spur, the TM was identified as fragmented fiber bundles that were colored black-red or black-brown (i.e., a mixture of collagen types I, III, and IV), and thus differed from the color of the ciliary muscle. The maximum thickness of the definite TM was 0.12-0.22 mm. The fragmented bundles corresponded to a lattice-like meshwork in a 3-dimensional view, and the color of each bundle appeared to be homogeneous. The posterior 2/3 or larger parts of the TM faced the anterior chamber. Schlemm's canal was adjacent to the outer aspect of the TM, and veins were evident in the sclera nearby. The connection between the ciliary muscle and the TM was weak, or even missing, because of loose interposing tissue (asterisk in Fig. 3). The corneal endothelium was continuous with the TM (Fig. 3B), but Schwalbe's line was difficult to identify in the sections. At these late stages, the iris root was occupied by loose mesenchymal tissue similar to the uveal meshwork in the early stage, although abundant capillaries or veins were evident.
Consequently, a major part of the ciliary muscle was not attached to the TM but to the scleral side of the scleral spur. At 20 weeks, an anlage of the TM appeared as a relatively dense area of fibrous tissue (rich in collagen types III and IV on the basis of its color), and this faced Schlemm's canal and continued to Descemet's membrane. The suggested TM anlage showed a change in collagen types up to 25 weeks (becoming a mixture of collagen types I, III, and IV), and was fasciculated, forming thick bundles that were woven into a lattice-like meshwork.
This study, using silver impregnation, demonstrated stepwise changes in the color and thickness of composite fibers of the TM and surrounding structures. However, without immunohistochemistry for smooth muscle actin, it was difficult to discriminate the uveal meshwork from the ciliary muscle in the early stage. In fact, Sevel and Isaacs [22] appeared to identify the muscle area as the primitive TM in the figure that they provided. The color of the TM fibers (or the anlage) changed from black to black-red or brown, which, according to the results of our previous studies [14-16], suggested a change in the collagen composition from types III and IV to a mixture of types I, III, and IV. This apparent mixture of collagen types in the fetal TM is consistent with a result obtained using materials from elderly men (aged 60 years or over) [23].
In general, as is also the case in human fetal eyes, collagen type IV, in combination with laminin, is a major component of basement membranes like Descemet's membrane [24]. Marshall et al. [13] reported that the basement membrane of TM endothelial cells exceptionally contained collagen type I. According to Ishii [25], silver staining demonstrated that very thin collagen type I fibers were black when an accompanying surface coat of the fibroblast basement membrane was present. Therefore, we cannot rule out the possibility that, in the present photos, some black-colored fibers, especially those in the suggested TM anlage, contained collagen type I. However, these very thin fibers would be unlikely to resist tensile stress. Silver staining may not identify elastin [26], collagen type VIII [27], and microfilaments [28], which have been reported in the human TM. However, the color changes that occurred during TM development were most likely a result of multiple changes in the components. Molecular biological studies using cultured TM cells have revealed that mechanical stress induces intracellular changes [29, 30].
Classically, mechanical stress has been hypothesized to account for TM differentiation, but the generator of the tension or traction proposed by various researchers has differed and has been attributed to 1) the long extending iris, 2) ciliary muscle contraction, and 3) elevated pressure within the fetal eye. Anderson [7] hypothesized that, after 20 weeks of gestation, more rapid growth of the cornea and sclera relative to the uvea resulted in a posterior shift of the iris root in accordance with the posterior traction of the TM. However, even in the late stages, the iris root was occupied by loose mesenchymal tissue similar to the uveal meshwork in the early stage, although abundant capillaries or veins were evident. These structures would be unlikely to conduct tensile stress from the extending iris. In contrast, Ruano-Gil et al. [9] hypothesized that mechanical forces induced TM differentiation in human fetuses with the aid of tensile stress that was generated by the ciliary muscle. They confirmed the connection between the ciliary muscle and the TM, although their figures did not include the topographical anatomy around the TM. In our study, this connection was not evident because of the presence of thin and few tendons at any stage, although the major part of the ciliary muscle was inserted into the scleral spur. In contrast, Smelser and Ozanics [31] provided beautiful photographs of fetal monkey eyes that clearly demonstrated the termination of all muscle fibers of the ciliary muscle at the developing trabecular meshwork. Thus, there appears to be a striking difference between human and monkey in the fetal development of the TM. Con versely, this difference seems to suggest that tensile stress resulting from ciliary muscle contraction is unlikely to play a major role in the induction of TM differentiation.
McMenamin [3] evaluated quantitative changes in the TM and iridocorneal angle space (showing a 100-200% increase between 12 and 22 weeks) and proposed that physiological demand accounted for TM differentiation. A recent molecular biology study also suggested that the remodeling of the extracellular matrix of the TM under mechanical stress resulted from elevated intraocular pressure, although the focus was more on adult pathology than on fetal development [30]. In this study, the anlage of the TM (i.e., a relatively dense fibrous tissue mass adjacent to Schlemm's canal) appeared at 20 weeks, in which the drainage vein was very thin or absent at the cornea-sclera junction. If elevated intraocular pressure is indeed the major cause, it seems to influence a structure that is much looser than the TM (e.g., the iris root). Thus, intraocular pressure does not appear to be the major initiator of TM differentiation. However, as reviewed by Johnstone [32], we cannot rule out the possibility that later growth of the TM depends on aqueous pressure.
Consequently, other than the iris, ciliary muscle, and intraocular pressure, we hypothesize that TM differentiation is induced by a combination of stresses at the cornea-sclera junction (Fig. 4). Among various candidate stress generators (Fig. 4A), we have already eliminated the iris root and the ciliary muscle. However, the contraction of the ciliary muscle is likely to contribute to the formation of the scleral spur. Fig. 4B shows the lamellar configuration of the corneasclera junction at the early fetal stage. At this junction, where different growth vectors impinge, remodeling of the vasculature has been well described [33, 34]. We hypothesize that this sharing stress first results in Schlemm's canal as a split by 20 weeks (Fig. 4C); subsequently (at and around 20 weeks), relatively dense fibrous tissue (i.e., the TM anlage) is induced to prevent tissue loss because of the aqueous pressure. This physiological response or repair seems to be consistent with another well-known phenomenon, the start of the endothelial lining of Schlemm's canal. Until 25 weeks, composite fibers of the TM anlage show a change in collagen types; the collagen becomes fasciculated, forming thick bundles that are woven into a lattice-like meshwork. These changes not only counteract the continuously generated stress at the corneasclera junction but also allow aqueous outflow through it. The TM endothelium may later extend along the meshwork fiber bundles after Schlemm's canal has become supplied with veins. With the aid of silver impregnation, overall views at relatively low magnification have thus enabled us to postulate a model of the changes that occur in and around the TM during fetal development.
The suggested late development of the TM would be responsible for one of the processes involved in the pathogenesis of congenital glaucoma: some external or maternal factor might influence the normal change of collagen types in the late stage of gestation. Before this study, we performed immunohistochemistry for collagens but obtained no convincing results, possibly because of the long preservation of the materials in formalin solution. If good human materials were to become available for immunohistochemistry, additional studies to reveal the detailed changes in collagen types at the fetal TM might become feasible.
Figures and Tables
Fig. 1
Early stage of iridocorneal angle development. Horizontal sections. (A, C) Silver impregnation and (B, D) smooth muscle actin immunohistochemistry. Panels (A) and (B) display sections from a 12-week fetus, while (C) and (D) show sections from a 15-week fetus. At these stages, the iridocorneal angle (star) is distant from the ciliary muscle. Smooth muscle is immunopositive (B, D) and is accompanied by types III and IV collagen fibers (black fibers in A and C). In the cornea and sclera, type I collagen fibers appear red with silver staining. At 15 weeks, small spaces appear in the loose mesenchymal tissue at the angle. The corneal endothelium (arrowheads in C) is well preserved in this specimen. Scale bar in (A)=0.2 mm (A-D).
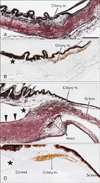
Fig. 2
Iridocorneal angle without a definite trabecular meshwork at 20 weeks. Horizontal sections. Silver impregnation (A, B) and smooth muscle actin immunohistochemistry (C). Panel (A) displays a specimen different from (B) and (C). Panel (C) shows a section taken from near (B). Schlemm's and collector canal (Schlemm) is evident in both specimens. On the corneal side of the ciliary muscle, a loose mesenchymal tissue, or the uveal meshwork, is evident (asterisk in A and B), but parts of the tissue form a relatively dense fibrous mass at the future trabecular meshwork (a perspective anlage; arrows in A and B). In (B), a thin vein passes through the margin of the sclera. The corneal endothelium is well preserved in these specimens (arrowheads in A and B). Scale bar in (A)=0.2 mm (A-C).
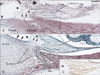
Fig. 3
Iridocorneal angle with a definite trabecular meshwork. Horizontal sections. Silver impregnation. Panel (A; panel B) displays a 25-week fetus (a 30-week fetus). In both specimens, the trabecular meshwork is evident (arrows) and is composed of red-black fiber bundles. Schlemm's canal (Schlemm) is adjacent to the meshwork. Insert at the lower right-hand angle is a higher magnification view of the meshwork. The brown fibers have collagen fiber types that differ from the red fibers in the cornea and sclera, as well as from the black fibers in the ciliary muscle. The sclera spur (spur) is evident in both specimens. The connection between the ciliary muscle and trabecular meshwork appears to be very weak because of loose interposing tissue (asterisk). A black-colored mass in the anterior chamber in (A) is blood, possibly due to injury during abortion. The corneal endothelium is well preserved in (B, arrowheads), and is continuous with the trabecular meshwork. Scale bars in (A)=0.2 mm (A, B).
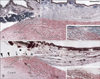
Fig. 4
Diagrams showing hypothetical induction of trabecular meshwork differentiation. Panel (A) displays four candidate generators of mechanical induction for early differentiation of the trabecular meshwork (TM): 1) growth of the iris, 2) contraction of the ciliary muscle, 3) thickening of the cornea, and 4) expansion of the sclera due to increased intraocular pressure. Panel (B) exhibits the lamellar configuration of the cornea and sclera in the early stage, while panel (C) shows lamellar distension and interlamellar dispersion due to sharing stress at the cornea-sclera junction. In (C), stars indicate the site at which the anlage of the TM appears. First, the sharing stress forms Schlemm's canal as a split, subsequently inducing the TM anlage to cover the tissue loss. Second, composite fibers of the TM anlage show a change in collagen types (becoming a mixture of types I, III, and IV), becoming fasciculated to form thick bundles and woven into a lattice-like meshwork.
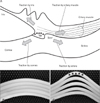
References
1. McMenamin PG. Human fetal iridocorneal angle: a light and scanning electron microscopic study. Br J Ophthalmol. 1989. 73:871–879.
2. McMenamin PG. A morphological study of the inner surface of the anterior chamber angle in pre and postnatal human eyes. Curr Eye Res. 1989. 8:727–739.
3. McMenamin PG. A quantitative study of the prenatal development of the aqueous outflow system in the human eye. Exp Eye Res. 1991. 53:507–517.
4. VanderWyst SS, Perkumas KM, Read AT, Overby DR, Stamer WD. Structural basement membrane components and corresponding integrins in Schlemm's canal endothelia. Mol Vis. 2011. 17:199–209.
5. Kupfer C. A note on the development of the anterior chamber angle. Invest Ophthalmol. 1969. 8:69–74.
6. Kupfer C, Ross K. The development of outflow facility in human eyes. Invest Ophthalmol. 1971. 10:513–517.
7. Anderson DR. The development of the trabecular meshwork and its abnormality in primary infantile glaucoma. Trans Am Ophthalmol Soc. 1981. 79:458–485.
8. Hansson HA, Jerndal T. Scanning electron microscopic studies on the development of the iridocorneal angle in human eyes. Invest Ophthalmol. 1971. 10:252–265.
9. Ruano-Gil D, Costa-Vila J, Barastegui C. Arrangement of the sclerocorneal trabecular system in human fetuses. Acta Anat (Basel). 1986. 127:233–236.
10. Tripathi RC. Davson H, Graham LT, editors. Comparative physiology and anatomy of the aqueous outflow pathway in the eye. The Eye. 1974. New York: Academic Press;163–336.
11. Hogan MJ, Alvarado JA, Weddell JE. Histology of the human eye: an atlas and textbook. 1971. Philadelphia: W. B. Saunders.
12. Meghpara B, Li X, Nakamura H, Khan A, Bejjani BA, Lin S, Edward DP. Human anterior chamber angle development without cell death or macrophage involvement. Mol Vis. 2008. 14:2492–2498.
13. Marshall GE, Konstas AG, Lee WR. Collagens in ocular tissues. Br J Ophthalmol. 1993. 77:515–524.
14. Abe S, Rhee SK, Osonoi M, Nakamura T, Cho BH, Murakami G, Ide Y. Expression of intermediate filaments at muscle insertions in human fetuses. J Anat. 2010. 217:167–173.
15. Osanai H, Abe S, Rodríguez-Vázquez J, Verdugo-López S, Murakami G, Ohguro H. Human orbital muscle: a new point of view from the fetal development of extraocular connective tissues. Invest Ophthalmol Vis Sci. 2011. 52:1501–1506.
16. Osanai H, Rodríguez-Vázquez JF, Abe H, Murakami G, Ohguro H, Fujimiya M. Fetal check ligament connected between the conjunctiva and the medial and lateral recti. Invest Ophthalmol Vis Sci. 2011. 52:7175–7179.
17. Speakman JS. Drainage channels in the trabecular wall of Schlemm's canal. Br J Ophthalmol. 1960. 44:513–523.
18. Lillie RD, Tracy RE, Pizzolato P, Donaldson PT, Reynolds C. Differential staining of collagen types in paraffin sections: a color change in degraded forms. Virchows Arch A Pathol Anat Histol. 1980. 386:153–159.
19. Satoh T, Takeda R, Oikawa H, Satodate R. Immunohistochemical and structural characteristics of the reticular framework of the white pulp and marginal zone in the human spleen. Anat Rec. 1997. 249:486–494.
20. Yamanouchi M. An electron microscopic study of the human iris vessels with special reference to the vascular changes on aging, using PAM-stain technique. Nihon Ganka Gakkai Zasshi. 1969. 73:767–784.
21. Duance VC, Stephens HR, Dunn M, Bailey AJ, Dubowitz V. A role for collagen in the pathogenesis of muscular dystrophy? Nature. 1980. 284:470–472.
22. Sevel D, Isaacs R. A re-evaluation of corneal development. Trans Am Ophthalmol Soc. 1988. 86:178–207.
23. Rehnberg M, Ammitzböll T, Tengroth B. Collagen distribution in the lamina cribrosa and the trabecular meshwork of the human eye. Br J Ophthalmol. 1987. 71:886–892.
24. Bystrom B, Virtanen I, Rousselle P, Gullberg D, Pedrosa-Domellof F. Distribution of laminins in the developing human eye. Invest Ophthalmol Vis Sci. 2006. 47:777–785.
25. Ishii T. On the light microscopic fine structure of reticular fibers in the lymph node (a contribution to the understanding of the nature of reticular fibers). Verh Anat Ges. 1967. 62:487–494.
26. Lutjen-Drecoll E, Futa R, Rohen JW. Ultrahistochemical studies on tangential sections of the trabecular meshwork in normal and glaucomatous eyes. Invest Ophthalmol Vis Sci. 1981. 21:563–573.
27. Tamura Y, Konomi H, Sawada H, Takashima S, Nakajima A. Tissue distribution of type VIII collagen in human adult and fetal eyes. Invest Ophthalmol Vis Sci. 1991. 32:2636–2644.
28. Grierson I, Rahi AH. Microfilaments in the cells of the human trabecular meshwork. Br J Ophthalmol. 1979. 63:3–8.
29. Tumminia SJ, Mitton KP, Arora J, Zelenka P, Epstein DL, Russell P. Mechanical stretch alters the actin cytoskeletal network and signal transduction in human trabecular meshwork cells. Invest Ophthalmol Vis Sci. 1998. 39:1361–1371.
30. Keller KE, Kelley MJ, Acott TS. Extracellular matrix gene alternative splicing by trabecular meshwork cells in response to mechanical stretching. Invest Ophthalmol Vis Sci. 2007. 48:1164–1172.
31. Smelser GK, Ozanics V. The development of the trabecular meshwork in primate eyes. Am J Ophthalmol. 1971. 71(1 Pt 2):366–385.
32. Johnstone MA. The aqueous outflow system as a mechanical pump: evidence from examination of tissue and aqueous movement in human and non-human primates. J Glaucoma. 2004. 13:421–438.
33. Hamanaka T, Bill A, Ichinohasama R, Ishida T. Aspects of the development of Schlemm's canal. Exp Eye Res. 1992. 55:479–488.
34. Smith RS, Zabaleta A, Savinova OV, John SW. The mouse anterior chamber angle and trabecular meshwork develop without cell death. BMC Dev Biol. 2001. 1:3.