Abstract
The supinator muscle originates from the annular ligament of the radius, and the muscle fibers and ligament take a similar winding course. Likewise, the coccygeus muscle and the sacrospinous ligament are attached together, and show a similar fiber orientation. During dissection of adult cadavers for our educational curriculum, we had the impression that these ligaments grow in combination with degeneration of parts of the muscles. In histological sections of 25 human fetuses at 10-32 weeks of gestation, we found that the proximal parts of the supinator muscle were embedded in collagenous tissue when the developing annular ligament of the radius joined the thick intermuscular connecting band extending between the extensor carpi radialis and anconeus muscles at 18-22 weeks of gestation, and the anterior parts of the coccygeus muscle were surrounded by collagenous tissue when the intramuscular tendon became the sacrospinous ligament at 28-32 weeks. Parts of these two muscles each seemed to provide a mold for the ligament, and finally became involved with it. This may be the first report to indicate that a growing ligament has potential to injure parts of the "mother muscle," and that this process may be involved in the initial development of the ligament.
Skeletal muscles are each connected to a tendon, which in turn is often joined to a ligament. Thus, discrimination between the tendon and the ligament is sometimes difficult, as in the case of the patellar tendon and ligament. A skeletal muscle sometimes originates from or inserts into a ligament: typical examples are seen around the elbow and knee joints. Thus, parts of the supinator muscle originate from the annular ligament of the radius (ANL), and both muscle and ligament take a winding course. The sacrospinous ligament (SSL) has been illustrated as a triangular structure with a medial base and a lateral apex [1, 2]. Hammer et al. [3] defined the SSL as a contorted frustum comprising a single cone with elliptic planes. Henle [4] and Fick [1] described the SSL as being interspersed by the coccygeus muscle fibers, and Hammer et al. [3] confirmed this fact by using the plastination technique. Both the "supinator and ANL" and the "coccygeus and SSL" combinations share a common feature in that the directions of their fibers are very similar in adults.
Mechanical loading by skeletal muscles is required for tendon regeneration and repair [5, 6]. Likewise, dynamic loading by skeletal muscles is critically important for the morphogenesis of tendon and bone. During fetal development, early muscle contraction confers a specific morphology on a tendon [5, 7], and in turn, the tendon confers a specific shape on the bone [8, 9]. Does the inductive relationship between a muscle and a tendon also apply to a muscle and a ligament? In the present study, by focusing on two muscle and ligament pairs, we investigated the possibility that the two structures might show coordinated development and growth, i.e., that the muscle confers a suitable morphology on the ligament. Nevertheless, during dissection of adult cadavers for our educational curriculum, we had an impression that these ligaments grow in combination with degeneration of parts of the muscles because the fiber directions were almost the same and because the muscles sometimes developed poorly.
Development of the ANL during the fetal stage has been described by many research groups [10-13], but its close relationship to the supinator has been referred to in only a single sentence: "The initial ANL is strongest posteriorly at the origin of the supinator muscle" [14]. Does the supinator develop prior to the ANL around the head of the radius? Fetal development of the coccygeus muscle has been described by several groups [15-17]. Niikura et al. [16] stated that the initial SSL is formed by a thick aponeurosis between the sacrococcygeus posterior muscle and the dorsolateral surface of the coccygeus muscle. Does the SSL develop from an intermuscular fascial structure? Consequently, to consider the possibility of coordinated development and growth between muscle and ligament, the aim of this study was to clarify the development of these two muscle and ligament pairs in human fetuses.
The study was performed in accordance with the provisions of the Declaration of Helsinki 1995 (as revised in Edinburgh, 2000). We examined the histology of paraffin-embedded specimens of 1) 15 mid-term human fetuses (crown-rump length [CRL], 55-165 mm; approximately 10-22 weeks of gestation) for observations of the supinator muscle and ANL and 2) 10 larger human fetuses (CRL, 150-260 mm; approximately 20-32 weeks of gestation) for observations of the coccygeus muscle and SSL. All the fetuses were part of the large collection kept at the Embryology Institute of the Universidad Complutense, Madrid, being the products of urgent abortion, miscarriages, or ectopic pregnancies managed at the Department of Obstetrics of the University. Approval for the study was granted by the ethics committee of the university.
The donated fetuses were fixed with 10% v/v formalin solution for more than 3 months. After division into the head and neck, thorax, upper extremities, abdomen, and pelvis with part of the thigh, and the other parts of lower extremities, the 15 specimens for the study of the elbow were decalcified by incubating them at 4℃ in a 0.5 mol/l EDTA solution (pH 7.5; Wako, Tokyo, Japan), while the other 10 larger specimens were decalcified with 5% v/v nitric acid at room temperature. Routine procedures for paraffin-embedded histology were performed. Five- (or 10-) µm-thick sections of the elbow (or pelvis) were prepared at intervals of 10-20 (100-200) µm. All elbows were cut transversely across the long axis of the radius, while for the pelvis, horizontal (3 specimens) or sagittal (7 specimens) sections were prepared. Most of the sections were stained with hematoxylin and eosin, Masson trichrome, or silver staining, while some sections of the smaller fetuses were studied via immunohistochemisty with mouse monoclonal anti-human desmin (1:50, M0760, Dako, Glostrup, Denmark) to show the striated muscle fibers. Autoclave pretreatment was not performed because of the loose nature of the fetal tissues. The secondary antibody (Dako Chem Mate Envision Kit, Dako) was labeled with horseradish peroxidase (HRP), and antigen-antibody reactions were detected via an HRP-catalyzed reaction with diaminobenzidine, followed by counterstaining with hematoxylin.
At 10 weeks, although it was still composed of loose fibrous tissue, the ANL was already evident in the deep side of the anconeus (Fig. 1). The supinator muscle originated from a ridge of the cartilagenous ulna via a long distinct tendon (Fig. 1A, B). Notably, at a more proximal level, the supinator tendon became thicker and was continuous with the ANL. In the superficial side of the tendon and ligament, an intermuscular fascia extended between the extensor carpi radialis and anconeus muscles: this fascia was much thinner than the ANL. However, at 12-15 weeks, the intermuscular fascia became thick, and at 18 weeks, it was much thicker than the ANL (Fig. 2). Thus, near the origin from the ulna, the supinator muscle fibers were sandwiched by the ANL and the intermuscular fascia or band (Fig. 2B, D). At 18 weeks, the supinator tendon was tightly attached to the ANL and the tendon was difficult to discriminate from the ligament. Parts of the supinator muscle were still attached to the head of the radius. We observed several thick muscle fibers with multiple nuclei in the supinator along the head of the radius (Fig. 2F). At 22 weeks, both the ANL and supinator muscle were well developed. The thick and tight ANL contained several long muscle fibers originating from the supinator muscle (data not shown).
At 18 weeks, the SSL was identified as a loose fibrous band that appeared to be an insertion tendon of the coccygeus muscle (Fig. 3). However, some muscle fibers of the coccygeus were already embedded in the loose ligament (Fig. 3C, D). The primitive ligament was not yet connected with the sacrum. The sacrococcygeus posterior muscle [16] appeared to have already united with the coccygeus. At 28 weeks, the obturator internus muscle was very thick and extended more posteriorly than the tendon curving along the ischium. The tendinous arch of the levator ani muscle was long and loose. An intramuscular tendon was evident in the coccygeus muscle, and this extended outside the muscle along the inferior aspect (Fig. 4). Near the tendon, some muscle fibers of the coccygeus were much thicker than others and had lost their myotube morphology (Fig. 4E). The sacrotuberous ligament was well developed near the ischial tuberosity at 28 weeks, but appeared to connect between the gluteus maximus muscle and the tuberosity (Fig. 4D). At 31 weeks, the intramuscular tendon of the coccygeus muscle was connected with the growing sacrotuberous ligament (Fig. 5). In the posteroinferior margin of the coccygeus muscle, we found a thick bundle of collagenous fibers, which corresponded to the final SSL (Fig. 5D) in view of its topographical relationship with the sacrotuberous ligament. The SSL contained irregularly arrayed muscle fibers (Fig. 5E).
Many ligaments in the human body do not apparently develop in association with muscle development. A typical example of such independent development and growth is the alar and transverse atlantis ligaments at the craniovertebral junction [18]. However, the ANL and SSL appeared to be different. The present observations demonstrated that, in human fetuses, 1) proximal parts of the supinator muscle were embedded in the collagenous tissue when the developing ANL joined the thick intermuscular connecting band and 2) the anterior parts of the coccygeus muscle were surrounded by this collagenous tissue when the intramuscular tendon became the SSL. Moreover, in the ANL and SSL, muscle fibers embedded in the ligaments suggested degeneration, or at least impairment of function. In fact, macrophage accumulation has been reported in the fetal ANL at a similar stage [19]. Thus, at these two sites, ligament growth appears not to be "favorable" for the developing muscles, in contrast to the coordinated development and growth that has been hypothesized (see Introduction). The fetal supinator was very thick relative to the size of the elbow. Thus, even if the ANL remained thin during development, the supinator muscle appeared to stabilize the growing head of the radius in utero. Likewise, even if the SSL was absent, development of the fetal sacroiliac joint appeared to be not impaired. Therefore, fetal growth of the ANL or SSL may occur independently from, or even beyond, functional demand at the corresponding joint. Although muscle contraction is likely to induce initial development of the ANL and SSL, the two appear to paradoxically injure parts of the "mother" muscles.
Reidenbach and Schmidt [13] stated that the ANL develops in stages in fetuses with a CRL between 105 and 270 mm, but considered that the term "growth" was preferable to "development." In the present study, we paid close attention to the early stage of development. According to Mérida-Velasco et al. [12], the first signs of the ANL are evident at 7 weeks, and the joint capsule becomes anchored to the ligament at 9 weeks. The thick intermuscular connecting band we observed at the elbow most likely corresponded to the lateral ulnar collateral ligament in adults described by O'Driscoll et al. [20], Hannouche and Bégué [21] and Imatani et al. [22]. Thus, at 15-18 weeks, the so-called lateral collateral ligament complex of the elbow [23] seemed to develop, with subsequent thickening of the ANL. In a study involving dissection from the superficial to the deep side of the elbow including the anconeus and extensor carpi ulnaris muscles, Kim et al. [24] published many elegant images of the lateral collateral ligament complex. In spite of its rather late development, the ANL in adults became much stronger than the ligament complex. Hast and Perkins [25] reported a cadaveric elbow showing rare anomalies: the accessory supinator and tensor muscles. Because these anomalous muscles were similar to the present supinator fibers embedded in the ANL, these muscles may result from failure of the muscle fibers to become involved in the ANL.
Development of the SSL chronologically followed the formation of the initial sacrotuberous ligament. The sacrotuberous ligament appeared to begin its development as a tendon of the gluteus maximus temporarily inserted to the ischial tuberosity. In adults, the gluteus maximus is, in fact, tightly attached to the tuberosity. In contrast, the SSL is much weaker than the sacrotuberous ligament, although both are considered critical for stabilizing the sacroiliac joint [26-28]. Because of the long and loose tendinous arch of the levator ani muscle in fetuses, the growth and contraction of the fetal rectum appears to not create mechanical stress on the coccygeus and its intramuscular tendon (i.e., the primitive SSL). In any event, development of both the SSL and the sacrotuberous ligament appears to not stabilize the fetal sacroiliac joint, but occurs as a result of mechanical influence from the nearby muscles. In the present study, the intramuscular tendon of the coccygeus appeared to provide a primitive form of the SSL. The union of the fetal coccygeus muscle with the sacrococcygeus posterior muscle [16] may induce formation of the intramuscular tendon.
At the beginning of this study, we had an impression that the ANL and SSL grow in combination with degeneration of parts of the corresponding muscles on the basis of our experience in dissection of adult cadavers (see Introduction). This study did not provide evidence of muscle cell death at the interface with the growing ligament, but we demonstrated that the developing ligament involved parts of the muscle fibers. Although we had no idea whether another example is present in the human body, we believe that a negative influence of developing ligaments on the muscles in contact with them is possible.
Figures and Tables
Fig. 1
Early-stage development of the annular ligament of the radius. A 10-week fetus (crown-rump length, 55 mm). Cross-sections of the elbow. Hematoxylin and eosin staining (A and C) and silver staining (B). Panel (A) (panel C) is the most distal (proximal) side of the figure. Intervals between panels are 0.1 mm (A-B, B-C). The supinator muscle originates from a ridge (open star) of the ulna via a long tendon (arrowheads in A and B). At levels between (B) and (C), this tendon is continuous with the primitive annular ligament of the radius (arrows in C). Outside the tendon and ligament, there is an intermuscular fascia (black stars) connecting the extensor carpi radialis (ECR) and anconeus muscles: this fascia is much thinner than the primitive annular ligament. BR, brachioradialis muscle. Scale bar in (A)=1 mm (A-C).
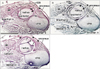
Fig. 2
Later development of the annular ligament of the radius. An 18-week fetus (crown-rump length, 155 mm). Cross-sections of the elbow. Panel (A) (panel E) is the most distal (proximal) side of the figure. Panels (B), (D), and (F) are higher-magnification views of the squares in panels (A), (C), and (E), respectively. Hematoxylin and eosin staining (A, C, E, F), Masson trichrome staining (D; adjacent section to panel C), and immunohistochemistry for desmin (B; adjacent section to panel A). Intervals between panels are 0.1 mm (A-C) and 0.5 mm (C-E). In contrast to Fig. 1, the intermuscular fascia (black stars in B and D) connecting the extensor carpi radialis (ECR) and anconeus muscles is much thicker than the annular ligament facing the head of the radius (arrows in B and D). At the side near the origin from the ulna, the supinator muscle fibers are sandwiched by the annular ligament and the intermuscular fascia (B, D). The tendon of the supinator has already become incorporated into the ligament. In the supinator facing the head of the radius, a thick muscle fiber with multiple nuclei is seen (arrows in F). BR, brachioradialis muscle. Scale bars=1 mm (A, C, E), 0.5 mm (B, D), 0.1 mm (F).
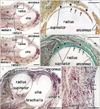
Fig. 3
Initial development of the sacrospinous ligament. An 18-week fetus (crown-rump length, 155 mm). Horizontal sections of the pelvis. Upper side of each panel corresponds to the anterior side of the pelvis. Panel (A) is 0.1 mm superior to panel (B) (A and B, hematoxylin and eosin [H&E] staining). Panel (C, desmin immunohistochemistry) displays an adjacent section to panel (B). Panel (D, H&E staining) is a higher-magnification view of a square in panel (B). The primitive sacrospinous ligament (stars in A and B) is a loose fibrous bundle that appears to be an insertion tendon of the coccygeus muscle. However, some muscle fibers are embedded in the ligament (arrows in C; higher magnification, D). The ligament has not yet connected with the sacrum. LA, levator ani muscle; OI, obturator internus muscle; PN, pudendal nerve. Scale bars in (A)=1 mm (A-C), in (D)=0.1 mm (D).
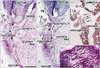
Fig. 4
Intramuscular tendon of the coccygeus connecting with the sacrotuberous ligament. A 28-week fetus (crown-rump length, 230 mm). Sagittal sections of the pelvis. hematoxylin and eosin staining. Panel (A) (panel D) is the most medial (lateral) side of the figure. The left-hand side of each panel corresponds to the anterior side of the pelvis. Intervals between panels are 1.0 mm (A-B), 0.8 mm (B-C), and 0.6 mm (C-D), respectively. Panel (E) is a higher-magnification view of a square in panel (D). The primitive tendinous arch (arrowheads in B) of the levator ani (LA) muscle extends anteriorly to reach the obturator internus muscle (OI). An intramuscular tendon is evident in the coccygeus muscle and along the inferior aspect of the muscle (arrows in B-D). Near the tendon, some muscle fibers of the coccygeus are thick and have lost their tube-like appearance (arrows in E). The developing sacrotuberous ligament (STL) has been partly damaged during histological preparation (asterisk in C). PN, pudendal nerve. Scale bars in (A)=2 mm (A-D), in (E)=0.05 mm (E).
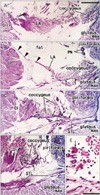
Fig. 5
Sacrospinous ligament appearing along the inferior margin of the coccygeus muscle. A 31-week fetus (crown-rump length, 255 mm). Sagittal sections of the pelvis. hematoxylin and eosain staining. Panel (A) (panel D) is the most medial (lateral) side of the figure. Intervals between panels are 1.2 mm (A-B), 1.6 mm (B-C), and 0.4 mm (C-D). Panel (E) is a higher-magnification view of the square in panel (D). The left-hand side of (A-C) corresponds to the anterior side of the pelvis, while that of panel (D) corresponds to the inferior side. The intramuscular tendon of the coccygeus muscle (arrows in C) is connected with the developing sacrotuberous ligament (STL). The sacrospinous ligament appears along the inferior margin of the coccygeus muscle (filled stars in D) and contains irregularly arrayed muscle fibers (open stars in E). LA, levator ani muscle; OI, obturator internus muscle. Scale bars=2 mm (A-D), 0.1 mm (E).
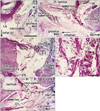
Acknowledgements
This study was supported by a grant (0620220-1) from the National R & D Program for Cancer Control, Ministry of Health & Welfare, Republic of Korea.
References
1. Fick R. von Bardeleben K, editor. Anatomie der Gelenke. Kreuz-Darmbein-gelenk., Handbuch der Anatomie und Mechanik der Gelenke unter Berűcksichtigung der bewegeden Muskeln. 1904. Vol. 2. Jena: Gustav Fischer;289–303.
2. Harrison DE, Harrison DD, Troyanovich SJ. The sacroiliac joint: a review of anatomy and biomechanics with clinical implications. J Manipulative Physiol Ther. 1997. 20:607–617.
3. Hammer N, Steinke H, Slowik V, Josten C, Stadler J, Böhme J, Spanel-Borowski K. The sacrotuberous and the sacrospinous ligament: a virtual reconstruction. Ann Anat. 2009. 191:417–425.
4. Henle J. Merkel F, editor. Bānder der unteren Extemitāt. Grundriss der Anatomie des Menschen. 1900. 3rd ed. Braunschweig: Friedrich Vieweg und Sohn;71.
5. Kjaer M, Langberg H, Heinemeier K, Bayer ML, Hansen M, Holm L, Doessing S, Kongsgaard M, Krogsgaard MR, Magnusson SP. From mechanical loading to collagen synthesis, structural changes and function in human tendon. Scand J Med Sci Sports. 2009. 19:500–510.
6. Thomopoulos S, Genin GM, Galatz LM. The development and morphogenesis of the tendon-to-bone insertion: what development can teach us about healing. J Musculoskelet Neuronal Interact. 2010. 10:35–45.
7. Mackey AL, Heinemeier KM, Koskinen SO, Kjaer M. Dynamic adaptation of tendon and muscle connective tissue to mechanical loading. Connect Tissue Res. 2008. 49:165–168.
8. Nowlan NC, Bourdon C, Dumas G, Tajbakhsh S, Prendergast PJ, Murphy P. Developing bones are differentially affected by compromised skeletal muscle formation. Bone. 2010. 46:1275–1285.
9. Rot-Nikcevic I, Reddy T, Downing KJ, Belliveau AC, Hallgrímsson B, Hall BK, Kablar B. Myf5-/- :MyoD-/- amyogenic fetuses reveal the importance of early contraction and static loading by striated muscle in mouse skeletogenesis. Dev Genes Evol. 2006. 216:1–9.
10. Mahasen LM, Sadek SA. Developmental morphological and histological studies on structures of the human fetal elbow joint. Cells Tissues Organs. 2000. 166:359–372.
11. Gray DJ, Gardner E. Prenatal development of the human elbow joint. Am J Anat. 1951. 88:429–469.
12. Mérida-Velasco JA, Sánchez-Montesinos I, Espín-Ferra J, Mérida-Velasco JR, Rodríguez-Vázquez JF, Jiménez-Collado J. Development of the human elbow joint. Anat Rec. 2000. 258:166–175.
13. Reidenbach MM, Schmidt HM. Prenatal development of the radial annular ligament. Ann Anat. 1993. 175:459–467.
14. Andersen H. Histochemical studies of the histogenesis of the human elbow joint. Acta Anat (Basel). 1962. 51:50–68.
15. Bardeen CR. Development and variation of the nerves and the musculature of the inferior extremity and of the neighboring regions of the trunk in man. Am J Anat. 1906. 6:259–390.
16. Niikura H, Jin ZW, Cho BH, Murakami G, Yaegashi N, Lee JK, Lee NH, Li CA. Human fetal anatomy of the coccygeal attachments of the levator ani muscle. Clin Anat. 2010. 23:566–574.
17. Power RM. Embryological development of the levator ani muscle. Am J Obstet Gynecol. 1948. 55:367–381.
18. Abe H, Ishizawa A, Cho KH, Suzuki R, Fujimiya M, Rodríguez-Vázquez JF, Murakami G. Fetal development of the transverse atlantis and alar ligaments at the craniovertebral junction. Clin Anat. 2012. 25:714–721.
19. Kim JH, Abe S, Shibata S, Asakawa S, Maki H, Murakami G, Cho BH. Dense distribution of macrophages in flexor aspects of the hand and foot of mid-term human fetuses. Anat Cell Biol. 2012. 45:259–267.
20. O'Driscoll SW, Horii E, Morrey BF, Carmichael SW. Anatomy of the ulnar part of the lateral collateral ligament of the elbow. Clin Anat. 1992. 5:296–303.
21. Hannouche D, Bégué T. Functional anatomy of the lateral collateral ligament complex of the elbow. Surg Radiol Anat. 1999. 21:187–191.
22. Imatani J, Ogura T, Morito Y, Hashizume H, Inoue H. Anatomic and histologic studies of lateral collateral ligament complex of the elbow joint. J Shoulder Elbow Surg. 1999. 8:625–627.
23. O'Driscoll SW, Bell DF, Morrey BF. Posterolateral rotatory instability of the elbow. J Bone Joint Surg Am. 1991. 73:440–446.
24. Kim PT, Isogai S, Murakami G, Wada T, Aoki M, Yamashita T, Ishii S. The lateral collateral ligament complex and related muscles act as a dynamic stabilizer as well as a static supporting structure at the elbow joint: an anatomical and experimental study. Okajimas Folia Anat Jpn. 2002. 79:55–61.
25. Hast MH, Perkins RE. Secondary tensor and supinator muscles of the human proximal radio-ulnar joint. J Anat. 1986. 146:45–51.
26. Dujardin FH, Roussignol X, Hossenbaccus M, Thomine JM. Experimental study of the sacroiliac joint micromotion in pelvic disruption. J Orthop Trauma. 2002. 16:99–103.
27. Seizeur R, Forlodou P, Person H, Morin JF, Sénécail B. The morphometric study of the sacrospinal and sacrotuberal ligaments correlated with the morphometry of the pelvis. Surg Radiol Anat. 2005. 27:517–523.
28. Simonian PT, Routt ML Jr, Harrington RM, Tencer AF. Anterior versus posterior provisional fixation in the unstable pelvis. A biomechanical comparison. Clin Orthop Relat Res. 1995. (310):245–251.