Abstract
Using immunohistochemical staining for alpha-smooth muscle actin (α-SMA), glial fibrillary acidic protein (GFAP), S100 protein (S100), p63, cytokeratin 14 (CK14), and cytokeratin 19 (CK19), we studied acinar and myoepithelial cells of major and minor salivary glands obtained from 14 donated cadavers (78-92 years old) and 5 donated fetuses (aborted at 15-16 weeks of gestation). CK and p63 expression was investigated only in the adult specimens. SMA was detected in all adult glands as well as in fetal sublingual and pharyngeal glands. GFAP expression was seen in a limited number of cells in adult glands, but was highly expressed in fetal pharyngeal glands. S100-positive myoepithelial-like cells were present in adult minor glands as well as in fetal sublingual and pharyngeal glands. Expression of p63 was evident in the ducts of adult glands. CK14 immunoreactivity was observed in a limited number of glandular cells in adults, in contrast to consistent expression of CK19. In both adults and fetuses, a mosaic expression pattern was usually evident for each of the examined proteins. A difference in immunoreactivity for the nerve markers GFAP and S100 was observed between the major and minor glands. Thus, in the present histologic study, we distinguished between the specific gland types on the basis of their immunohistochemical staining. A mosaic expression pattern suggested that the immunoreactivity against nerve protein markers in myoepithelial cells could not be due to the persistence of neural crest remnants or the physiological status of the gland, such as age-related degeneration.
Under normal conditions, in the absence of neoplasia, major and minor salivary glands express nerve markers such as glial fibrillary acidic protein (GFAP, one of the intermediate filament proteins), S100 protein (S100, a well-known marker for glial and Schwann cells), and neuron-specific enolase (NSE) [1-6]. These neural markers, as well as protein markers for smooth muscle (e.g., alpha-smooth muscle actin [α-SMA]) are usually used for classification of salivary gland tumors [7-9]. Another marker for myoepithelial cells, p63 (a homologue of the tumor suppressor p53), which is also a well-known marker for Müllerian duct derivatives in the urogenital organs [10-13], has recently been used for classification of salivary gland tumors [7, 14]. Thus, immunoreactivity for p63 does not indicate origin in a specific cell. In addition, cytokeratins, a group of intermediate filament proteins, are used as markers for salivary gland epithelium. Cytokeratin19 (CK19) is commonly used as a marker for the neuroendocrine and gastrointestinal tumors [15]. Cytokeratin14 (CK14) is also a marker for oral epithelial cells [16, 17] and is considered a marker for maturation of the epithelial lining [17, 18].
Although these markers have demonstrated differential expression patterns in myoepithelial cells of various salivary glands, to the best our knowledge, criteria to distinguish between specific glands on the basis of immunohistochemistry are yet to be established. Moreover, previously reported data on expression of neuronal markers in fetal salivary glands are inconsistent; for example, Gustafsson et al. [1] and Lee et al. [2] stated that fetal parotid glands expressed GFAP, whereas Chi [19] and Ianez et al. [14] found no evidence for GFAP immunostaining in these glands at a similar gestational age. Therefore, in this study, we aimed to comprehensively examine the immunohistochemical reactivity toward the described markers not only in the major parotid and submandibular glands, but also in the minor glands such as pharyngeal gland near the palatine tonsil, the lingual gland at the dorsum of the tongue, and the palatal gland by using specimens from cadavers of elderly persons. For comparison, we also examined fetal parotid and submandibular glands (obtained from fetuses at 15-16 weeks of gestation) because according to Chi [19] and Ianez et al. [14], at this stage, myoepithelial cells of major salivary glands stain positive for α-SMA, but negative for S100.
The study was performed in accordance with the provisions of the Declaration of Helsinki 1995 (as revised in Edinburgh 2000). We examined 14 donated cadavers (8 men and 6 women; age, 78-92 years; mean age, 88 years). Causes of death were ischemic heart failure or intracranial bleeding. These cadavers were donated to the Tokyo Dental College for research on human anatomy, and their use in this study was approved by the university ethics committee. The donated cadavers had been fixed by arterial perfusion of 10% v/v formalin solution and stored in 50% v/v ethanol solution for more than 3 months. From 1 cadaveric head, we prepared 4 tissue blocks, each of which included 1) the tonsillar fossa and the dorsal part of the tongue, 2) the soft palate and opening of the pharyngotympanic tube, 3) the superficial part of the parotid gland, or 4) the superficial part of the submandibular gland. After performing routine procedures for preparing paraffin-embedded histological specimens, semiserial sections were cut at 0.2-mm intervals. One of every 10 sections was stained with hematoxylin and eosin (H&E) to screen for sections suitable for gland immunohistochemistry (see below).
We also examined the histology of 5 paraffin-embedded fetuses at an estimated gestational age of 15-16 weeks (crown-rump length, or CRL, 105-125 mm). By approbation of the families concerned, these specimens were donated to the Department of Anatomy, Chonbuk National University, Korea, and their use in this study was approved by the university ethics committee. According to the regulations, authors other than those affiliated to the Chonbuk University were not required to inform the corresponding committee in Japan about this research project. The fetuses were obtained after induced abortions, and each of the mothers were personally informed by an obstetrician about the possibility of donating the fetus for research without any attempt to encourage donation. Because of the randomized numbering of specimens, tracing the families concerned was not possible. The donated fetuses were fixed in 10% w/w neutral formalin solution for more than 3 months. After division into the head and neck, thorax, abdomen, pelvis, and the 4 extremities, all parts were decalcified by incubating in 0.5 mol/l ethylenediaminetetraacetic acid solution (pH 7.5; Decalcifying Solution B, Wako, Tokyo, Japan) at 4℃ for 1-3 days, depending on the size of the specimen. Using a routine procedure, we obtained paraffin-embedded histological sections 5 µm thick. All head specimens were processed into sagittal (3 specimens) or horizontal (2 specimens) sections at intervals of 50 µm. Most of the sections were stained with H&E, whereas some were used for immunohistochemistry (see below).
The primary antibodies used for immunohistochemistry were 1) rabbit polyclonal anti-human GFAP (1:100, #Z0334, Dako Cytomation, Kyoto, Japan), 2) mouse monoclonal anti-human α-SMA (1:100, #M0760, Dako, Glostrup, Denmark), 3) mouse monoclonal anti-human S100 (1:100, #Z0311, Dako), 3) mouse monoclonal [BC4A4] anti-human p63 (1:100, #ab735, Abcam, Cambridge, UK), 4) mouse monoclonal anti-human CK14 (1:50, #LL002, Novo, Newcastle upon Tyne, UK), and 5) mouse monoclonal anti-human CK19 (1:100, #sc-6278, Santa Cruz Biotechnology, Santa Cruz, CA, USA). With the exception of the samples used for detection of S100 and SMA, antigen retrieval was performed using microwave treatment (500 W, 15 minutes, pH 6). Sections were incubated with a secondary horseradish peroxidase (HRP)-labeled antibody (1:1,000, Histofine Simple Stain Max-PO, Nichirei, Tokyo, Japan) for 30 minutes, and immunoreactivity was detected using an HRP-catalyzed reaction with diaminobenzidine (Histofine Simple Stain DAB, Nichirei) for 3-5 minutes. All samples were counterstained with hematoxylin. Negative controls consisted of samples processed without incubation with primary antibodies. Adult specimens were examined using all 6 markers, but only 3 of these markers (α-SMA, GFAP, and S100) were tested in the fetal specimens because difference in reactivity for these markers was evident between the major and minor adult glands. In addition, the Dako anti-α-SMA antibody has been shown to react strongly with the endothelium of blood vessels [20].
The major parotid gland displayed a mosaic expression pattern for α-SMA, with some of the myoepithelial-like cells staining positive, whereas others stained negative (Fig. 1). The α-SMA-positive and α-SMA-negative cells did not form large clusters but rather appeared randomly intermingled. The expression of both GFAP and p63 was restricted to the ducts. Unexpectedly, reactivity for S100 was found not only in the thin nerves around the acinus but also in fatty tissues embedded in the gland because of age-related degeneration. CK19 expression was seen in all ducts of the parotid gland, but CK14 expression was detected only in some cells in the ducts. In contrast, in the submandibular gland (Fig. 2), most of the myoepithelial-like cells were strongly positive for α-SMA. However, no acinar or myoepithelial-like cells appeared to express GFAP or S100. The patterns of p63 and CK19 expression were similar to those in the parotid gland, and CK14 was not detected in the submandibular gland. These expression patterns observed in the major glands were similar in all specimens.
Among the minor glands, the small lingual gland in the dorsal surface of the tongue (Fig. 3) was characterized by strong expression of S100 in most of the myoepithelial-like cells; however, α-SMA and GFAP both showed a mosaic pattern of expression. In contrast, the small pharyngeal gland near the tonsillar fossa (Fig. 4) displayed a mosaic pattern of S100 immunoexpression, whereas α-SMA was strongly expressed in all myoepithelial-like cells. Unlike the lingual and pharyngeal glands, the small palatal gland in the soft palate showed individual differences in the numbers of GFAP- and α-SMA-positive myoepithelial-like cells (Figs. 5, 6), constituting less than 50% of the cells in the acinus of 10 specimens (Fig. 5B, C) in contrast to almost 100% in the acinus of the 4 remaining specimens (Fig. 6A, B). In the palatal gland, S100 expression was observed in some acinus-like and most of the myoepithelial-like cells. Expression patterns for CK19, CK14, and p63 were similar in the minor and major salivary glands. The results of the immunohistochemical analysis of the adult specimens are summarized in Table 1.
Overall, each of the 5 examined salivary glands demonstrated specific immunohistochemical characteristics: 1) the parotid gland showed S100-positive fatty tissue degeneration with aging; 2) the submandibular gland had no GFAP-, S100-, or CK14-positive cells; 3) the myoepithelial cells in the lingual gland displayed strong and diffuse immunoreactivity for S100, but the ducts did not contain p63-positive cells; 4) among the examined markers, negative immunoreactivity was observed only for GFAP in the pharyngeal gland; and 5) the palatal gland showed reactivity for all the immunohistochemical markers in this study. Thus, in the present histological study, we demonstrated the possibility to distinguish the individual glands on the basis of their immunohistochemical staining patterns.
Because the sublingual gland was coincidentally included in the same sections, we studied it after complete preparation of the adult specimens. The parotid, submandibular and sublingual glands appeared to be at the pseudoglandular stage as per the classification by Tucker [21]. In contrast, the pharyngeal glands near the putative palatine tonsil were near the terminal bud stage. Glandular cells of the parotid and submandibular glands did not show reactivity for SMA, GFAP, or S100 (Fig. 7). In the submandibular gland (but not in other glands), abundant S100-positive thin nerves encircled each acinus. Thus, when glandular cells were cut tangentially, discrimination of the S100 immunoreactivity in the nerves from that in the glandular cells became difficult. In contrast, the sublingual gland contained both SMA-positive acinar cells and S100-positive myoepithelial-like cells (Fig. 8A-C). The positive cells were distributed among the negative cells in a mosaic pattern. It should be noted that despite the early stage of development, pharyngeal gland cells expressed SMA, GFAP, and/or S100 (Fig. 8D, E, G); these glandular cells were different in shape from both SMA-positive vessels and S100-positive nerves. Fetal brains from the same specimens contained GFAP-positive glial cells (Figs. 7F, 8F). The results for the fetal specimens are summarized in Table 2.
In conclusion, we demonstrated differences in immunoreactivity toward the nerve markers GFAP and S100 between the major (parotid and submandibular) and minor (pharyngeal, lingual, and palatal) salivary glands. In contrast to the major glands, the minor glands, except the adult pharyngeal gland, tended to express both S100 and GFAP.
In this study, we investigated 2 major and 3 minor adult salivary glands; therefore, our results appeared to be more comprehensive than those of previous studies. However, we were unable to include observations of the adult sublingual gland. We observed the specific morphology of the fetal sublingual gland after complete preparation of adult specimens. Possibly because of age-related degeneration, adult tissues obtained from cadavers of elderly individuals have not been studied extensively. However, the present results seemed to be consistent with those of Gustafsson et al. [1], who reported co-expression of GFAP and CK in some myoepithelial cells in parotid and palatal glands, but not in submandibular glands. In regard to S100 and GFAP expression in the major glands, our results were mostly consistent with those of Nakazato et al. [4], Murakami et al. [3], and Okura et al. [5], who observed uniform negative staining for these markers in clinical materials obtained from patients of different age groups. We demonstrated the differences in immunoreactivity among various types of salivary glands; had adult sublingual glands also been examined, the difference would have been even more distinct. Heterogeneity of salivary glands is poorly understood. Lee et al. [2] described the details of stage-dependent changes in immunoreactivity toward S100, GFAP, and NSE by using 100 human fetal salivary glands; however, they did not discriminate among the gland types, and therefore, their results probably reflect type- and not stage-specific differences.
Many previous studies addressed an important question of whether salivary gland cells, especially myoepithelial cells, express S100. In his excellent immunohistochemical study of the major salivary glands, Chi [19] observed that S100 reactivity was limited to fetuses at 19-40 weeks of gestation and was absent in adults. Likewise, Mori et al. [22] and Dardick et al. [23] reported that myoepithelial cells of adult major salivary glands were negative for S100. The occasional positive reaction for S100 in myoepithelium was attributed to staining of unmyelinated nerve endings, and unmyelinated nerve endings were suggested to make direct contact with myoepithelial and acinar cells [24]. In fact, in the present study, we did not observe S100-positive glandular cells in the major glands, although these were specifically evident in fetal submandibular glands abundant the thin nerves that encircled each acinus. In the present study, we demonstrated the presence of S100-positive glandular cells in the minor salivary glands in both adults and fetuses. Accordingly, we believe that some myoepithelial cells may express S100 in salivary glands, which is consistent with the statement that S100 is expressed in the intercalated duct and myoepithelial cells of the major salivary glands [25].
The most striking finding of the present study is a distinct difference between the major (parotid and submandibular) and the minor (pharyngeal, lingual, and palatal) salivary glands in their immunoreactivity toward the nerve markers GFAP and S100. Observations in fetuses suggested that adult sublingual glands were likely to show immunoreactivity similar to that of the minor glands, unlike the nearby submandibular glands. In fetuses, the mesenchymal tissue around the sublingual gland was continuous with that around the submandibular gland. Thus, the epithelial-mesenchymal interaction did not seem critical for the expression of nerve markers. However, given the rich nerve supply in fetuses, nerves were likely to induce specific morphology in the submandibular gland. Were there neural crest remnants persisting in myoepithelial cells of the adult minor glands? It is necessary to bear in mind that expression of GFAP and S100 is not strictly nerve-specific and can also occur in the cartilage in both fetuses and adults [26, 27]. Thus, the S100 or GFAP expression does not always indicate a neural origin. Because fetal major glands were negative for α-SMA, the fetal myoepithelial cells did not seem to have reached sufficiently differentiated stage for contraction in contrast to the pharyngeal small gland. Therefore, expression of these nerve markers does not depend on the stage of differentiation, but rather on other factors. If immunoreactivity for nerve marker proteins depends on the physiological status of the gland, such as age-related degeneration, then the mosaic expression pattern we observed may be thought to reflect the functional differences among adjacent glandular cells. However, such differences are unlikely to occur in a single acinus. Finally, the most basic observation in this study-the small glands expressed nerve markers, whereas the large glands did not-should also be noted. Therefore, immunoexpression may be determined by the size of the gland or the degree of branching in the ductal system. Notably, immunohistochemistry of the branching morphogenesis has been investigated in the small salivary glands, but not in the major glands [28].
Figures and Tables
Fig. 1
Immunohistochemistry of the parotid gland (78-year-old woman). (A) p63, (B) alpha-smooth muscle actin (α-SMA), (C) glial fibrillary acidic protein (GFAP), (D) S100 protein (S100), (E) cytokeratin 19 (CK19), (F) cytokeratin 14 (CK14). (A) p63 is expressed in the thick ducts (arrows). (B) α-SMA is expressed in some of the myoepithelial-like cells. (C) GFAP is weakly expressed in ducts (arrows). (D) Fatty tissues as well as nerve terminals around the acinus express S100. (E) CK19 is expressed in all the ducts. (F) CK14 is expressed in cells included in thick ducts (arrows). All micrographs were taken at the same magnification. Scale bar in (A)=0.1 mm (A-F).
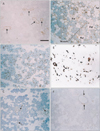
Fig. 2
Immunohistochemistry of the submandibular gland (78-year-old woman). The specimen was obtained from the same cadaver as in Fig. 1. (A) p63, (B) alpha-smooth muscle actin (α-SMA), (C) glial fibrillary acidic protein (GFAP), (D) S100 protein (S100), (E) cytokeratin 19 (CK19), (F) cytokeratin 14 (CK14). (A) p63 is positive in thick ducts (arrows). (B) SMA is strongly expressed in all or most of the myoepithelial-like cells. (C) Reactivity toward GFAP is evident in some nerve-like structures. (D) S100 expression appears to be limited to nerves. (E) CK19 is expressed in some acinar cells as well as in all the ducts. (F) CK14 expression was not observed. All micrographs were taken at the same magnification. Scale bar in (A)=0.1 mm (A-F).
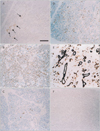
Fig. 3
Immunohistochemistry of a small lingual gland at the dorsal aspect of the tongue (81-year-old man). (A) p63, (B) alpha-smooth muscle actin (α-SMA), (C) glial fibrillary acidic protein (GFAP), (D) S100 protein (S100), (E) cytokeratin 19 (CK19), (F) cytokeratin 14 (CK14). (A) p63 is very weakly expressed in the thick ducts (arrows). (B) SMA is expressed in some myoepithelial-like cells (arrows). (C) Reactivity toward GFAP is evident in a limited number of myoepithelial-like cells (arrows). (D) All or most of the myoepithelial cells express S100 (arrows). (E) CK19 is expressed in some acinar cells as well as in all ducts. (F) CK14 expression was not observed. All micrographs were taken at the same magnification. Scale bar in (A)=0.1 mm (A-F).
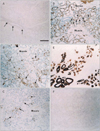
Fig. 4
Immunohistochemistry of a small pharyngeal gland near the tonsillar fossa (85-year-old man). (A) p63, (B) alpha-smooth muscle actin (α-SMA), (C) glial fibrillary acidic protein (GFAP), (D) S100 protein (S100), (E) cytokeratin 19 (CK19), (F) cytokeratin 14 (CK14). (A) p63 is weakly expressed in some thick ducts (arrows). (B) SMA is strongly expressed in all myoepithelial-like cells. (C) GFAP is negative. (D) S100 expression is seen in some acinus-like and myoepithelial-like cells (arrows) as well as in nerves. (E) CK19 is expressed in acinar cells as well as in all ducts. (F) CK14-positive cells are included in some thick ducts (arrows). All micrographs were taken at the same magnification. Scale bar in (A)=0.1 mm (A-F).
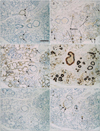
Fig. 5
Immunohistochemistry of a small palatal gland at the soft palate (88-year-old man). (A) p63, (B) alpha-smooth muscle actin (α-SMA), (C) glial fibrillary acidic protein (GFAP), (D) S100 protein (S100), (E) cytokeratin 19 (CK19), (F) cytokeratin 14 (CK14). (A) p63 is weakly expressed in some thick ducts (arrows). (B) SMA is expressed in a limited number of myoepithelial-like cells (arrows). (C) GFAP is weakly expressed in some ducts (arrows). (D) S100 expression is seen in myoepithelial-like cells (arrows) as well as in nerves. (E) CK19 is expressed in all the ducts. (F) CK14 reactivity is seen in the debris in the thick ducts (stars) as well as in some myoepithelial-like cells (arrows). All micrographs were taken at the same magnification. Scale bar in (A)=0.1 mm (A-F).
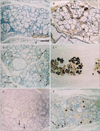
Fig. 6
Immunohistochemistry of a small gland in the soft palate (78-year-old woman). The specimen was obtained from the same cadaver as that shown in Figs. 1 and 2. (A) Alpha-smooth muscle actin (α-SMA), (B) glial fibrillary acidic protein (GFAP), (C) S100 protein (S100). (A) α-SMA is expressed in most of the myoepithelial-like cells. In contrast to Fig. 5, GFAP is expressed in some myoepithelial-like cells (arrows, B). (C) S100 is expressed in some acinus-like cells and most of the myoepithelial-like cells (arrows). All micrographs were taken at the same magnification. Scale bar in (A)=0.1 mm (A-C).
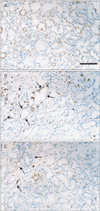
Fig. 7
Immunohistochemistry of the fetal parotid and submandibular glands (15 weeks). (A-C) Parotid gland, (D, E, G) submandibular gland. (F) Glial fibrillary acidic protein (GFAP) immunostaining of the brain (same specimen) as a positive control for GFAP. (A, D) Alpha-smooth muscle actin (α-SMA), (B, E, F) GFAP, (C, G) S100 protein (S100). α-SMA reactivity is restricted to vessels. GFAP is expressed in the brain (F) but not in these glands. (G) S100 is expressed in developing nerves, which encircle most of the acinus of the submandibular gland (arrows). All micrographs were taken at the same magnification Scale bar in (A)=0.1 mm (A-G).
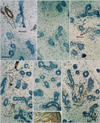
Fig. 8
Immunohistochemistry of fetal sublingual and pharyngeal glands (15 weeks). A specimen obtained from the same fetus as that shown in Fig. 7. Upper panels (A-C) displays the sublingual gland and the lower panels (D, E, G) shows the pharyngeal gland. The lower panels at a magnification higher than that of the upper panels (scale bar in A and D). Panel (F) shows glial fibrillary acidic protein (GFAP) immunostaining of the brain (same specimen) as a positive control for GFAP. (A, D) Alpha-smooth muscle actin (α-SMA), (B, E, F) GFAP, (C, G) S100 protein (S100). α-SMA reactivity is seen not only in vessels, but also in some myoepithelial-like cells (arrowheads in A and D). (E) GFAP is expressed in some myoepithelial-like cells of the pharyngeal gland (arrowheads) and in the brain (F). (C, G) S100-positive nerves encircle most of the acinus of the submandibular and pharyngeal glands (arrows). In addition, some acinus-like cells in both glands also express S100 (arrowheads, C and G). Panels (A-C) (panels D-G) were prepared at the same magnification. Scale bar in (A)=0.1 mm (A-C), in (D)=(D-G).
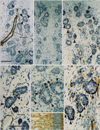
References
1. Gustafsson H, Virtanen I, Thornell LE. Glial fibrillary acidic protein and desmin in salivary neoplasms: expression of four different types of intermediate filament proteins within the same cell type. Virchows Arch B Cell Pathol Incl Mol Pathol. 1989. 57:303–313.
2. Lee SK, Kim EC, Chi JG, Hashimura K, Mori M. Immunohistochemical detection of S-100, S-100 alpha, S-100 beta proteins, glial fibrillary acidic protein, and neuron specific enolase in the prenatal and adult human salivary glands. Pathol Res Pract. 1993. 189:1036–1043.
3. Murakami M, Makino I, Nin F, Nishiyama Y, Saitou Y, Murakami Y. Immunohistological investigation of the histological origin and differentiation of pleomorphic adenoma of the parotid gland. Nihon Jibiinkoka Gakkai Kaiho. 1993. 96:1235–1245.
4. Nakazato Y, Ishida Y, Takahashi K, Suzuki K. Immunohistochemical distribution of S-100 protein and glial fibrillary acidic protein in normal and neoplastic salivary glands. Virchows Arch A Pathol Anat Histopathol. 1985. 405:299–310.
5. Okura M, Hiranuma T, Tominaga G, Yoshioka H, Aikawa T, Shirasuna K, Matsuya T. Expression of S-100 protein and glial fibrillary acidic protein in cultured submandibular gland epithelial cells and salivary gland tissues: histogenetic implication for salivary gland tumors. Am J Pathol. 1996. 148:1709–1716.
6. Sugiura R, Kuyama K, Utsunomiya T, Morikawa M, Fukumoto M, Yamamoto H. Myoepithelioma arising from the buccal gland: histopathological and immunohistochemical studies. J Oral Sci. 2000. 42:39–42.
7. Angiero F, Sozzi D, Seramondi R, Valente MG. Epithelial-myoepithelial carcinoma of the minor salivary glands: immunohistochemical and morphological features. Anticancer Res. 2009. 29:4703–4709.
8. Ogawa I, Nishida T, Miyauchi M, Sato S, Takata T. Dedifferentiated malignant myoepithelioma of the parotid gland. Pathol Int. 2003. 53:704–709.
9. Santos EP, Cavalcante DR, Melo AU, Pereira JC, Gomes MZ, Albuquerque RL Jr. Plasmacytoid myoepithelioma of minor salivary glands: report of case with emphasis in the immunohistochemical findings. Head Face Med. 2011. 7:24.
10. Kurita T, Cunha GR. Roles of p63 in differentiation of Mullerian duct epithelial cells. Ann N Y Acad Sci. 2001. 948:9–12.
11. Kurita T, Cunha GR, Robboy SJ, Mills AA, Medina RT. Differential expression of p63 isoforms in female reproductive organs. Mech Dev. 2005. 122:1043–1055.
12. Martens JE, Smedts F, van Muyden RC, Schoots C, Helmerhorst TJ, Hopman A, Ramaekers FC, Arends JW. Reserve cells in human uterine cervical epithelium are derived from mullerian epithelium at midgestational age. Int J Gynecol Pathol. 2007. 26:463–468.
13. Shapiro E, Huang H, McFadden DE, Masch RJ, Ng E, Lepor H, Wu XR. The prostatic utricle is not a Mullerian duct remnant: immunohistochemical evidence for a distinct urogenital sinus origin. J Urol. 2004. 172(4 Pt 2):1753–1756.
14. Ianez RF, Buim ME, Coutinho-Camillo CM, Schultz R, Soares FA, Lourenco SV. Human salivary gland morphogenesis: myoepithelial cell maturation assessed by immunohistochemical markers. Histopathology. 2010. 57:410–417.
15. Jain R, Fischer S, Serra S, Chetty R. The use of cytokeratin 19 (CK19) immunohistochemistry in lesions of the pancreas, gastrointestinal tract, and liver. Appl Immunohistochem Mol Morphol. 2010. 18:9–15.
16. Iwasaki S, Aoyagi H, Yoshizawa H. Localization of keratins 13 and 14 in the lingual mucosa of rats during the morphogenesis of circumvallate papillae. Acta Histochem. 2011. 113:395–401.
17. Lourenço SV, Lima DM, Uyekita SH, Schultz R, de Brito T. Expression of beta-1 integrin in human developing salivary glands and its parallel relation with maturation markers: in situ hybridisation and immunofluorescence study. Arch Oral Biol. 2007. 52:1064–1071.
18. Martins MD, Cavalcanti de Araujo V, Raitz R, Soares de Araújo N. Expression of cytoskeletal proteins in developing human minor salivary glands. Eur J Oral Sci. 2002. 110:316–321.
19. Chi JG. Prenatal development of human major salivary glands. Histological and immunohistochemical characteristics with reference to adult and neoplastic salivary glands. J Korean Med Sci. 1996. 11:203–216.
20. Hayashi S, Murakami G, Ohtsuka A, Itoh M, Nakano T, Fukuzawa Y. Connective tissue configuration in the human liver hilar region with special reference to the liver capsule and vascular sheath. J Hepatobiliary Pancreat Surg. 2008. 15:640–647.
21. Tucker AS. Salivary gland development. Semin Cell Dev Biol. 2007. 18:237–244.
22. Mori M, Murase N, Hosaka M, Orito T. Immunohistochemical expression of S-100 protein in reactive and neoplastic myoepithelial cells of variant salivary pleomorphic adenomas. Acta Histochem Cytochem. 1986. 19:231–240.
23. Dardick I, Stratis M, Parks WR, DeNardi FG, Kahn HJ. S-100 protein antibodies do not label normal salivary gland myoepithelium. Histogenetic implications for salivary gland tumors. Am J Pathol. 1991. 138:619–628.
24. Huang JW, Hashimura K, Sakamoto F, Yuba R, Mori M, Yoneda K, Yanagihara M, Mori S. Heterogeneity and multiple expression of intermediate filament proteins, S-100 protein and neuron specific enolase in skin mixed tumor. Anticancer Res. 1992. 12:1107–1114.
25. Martínez-Madrigal F, Bosq J, Casiraghi O. Sternberg SS, editor. Major salivary glands. Histology for Pathologists. 1997. 2nd ed. Philadelphia: Lippincott-Raven;405–429.
26. Jin ZW, Song KJ, Lee NH, Nakamura T, Fujimiya M, Murakami G, Cho BH. Contribution of the anterior longitudinal ligament to ossification and growth of the vertebral body: an immunohistochemical study using the human fetal lumbar vertebrae. Surg Radiol Anat. 2011. 33:11–18.
27. Miyako H, Suzuki A, Nozawa-Inoue K, Magara J, Kawano Y, Ono K, Maeda T. Phenotypes of articular disc cells in the rat temporomandibular joint as demonstrated by immunohistochemistry for nestin and GFAP. J Anat. 2011. 219:472–480.
28. Lourenço SV, Coutinho-Camillo CM, Buim ME, Uyekita SH, Soares FA. Human salivary gland branching morphogenesis: morphological localization of claudins and its parallel relation with developmental stages revealed by expression of cytoskeleton and secretion markers. Histochem Cell Biol. 2007. 128:361–369.