Abstract
The formation of neural synapses according to the development and growth of neurite were usually studied with various markers. Of these markers, synaptophysin is a kind of synaptic protein located in the synaptic vesicle of neuron or neuroendocrine cell known to be distributed consistently in all neural synapses. The purpose of this study was to investigate differential expression levels and patterns of synaptic marker (synaptophysin) in the mouse hippocampal region according to the developmental stages of embryonic, neonatal, and adulthood respectively. In the embryonic and neonatal groups, synaptophysin immunofluorescence was almost defined to cornu ammonis subfields (CA1 and CA3) of hippocampus and subiculum proper in the hippocampal region. However in dentate gyrus, synaptophysin immunoreactivities were insignificant or absent in all developmental stages. In embryonic and neonatal hippocampus, the intensities of immunofluorescence were significantly different between molecular and oriens layers. Furthermore, those intensities were decreased considerably in both layers of neonatal group compared to embryonic. The results from this study will contribute to characterizing synaptogenic activities in the central nervous system through developmental stages.
Differentiation and growth of central nervous system are accomplished through relatively early stages of development. The formation of a neural synapse indicates the beginning of electrical signaling between neurons, so that may be a critical step in the differentiation of neurons as well as the development and growth of central nervous system. The formation of neural synapses according to the development and growth of neurite were usually studied with various markers. With regard to neurite sprouting and outgrowth along with synaptogenesis, a variety of markers are used to detect core matter and core factors. Many researchers who study synapse formation select a few substances such as growth associated protein-43 (GAP-43), synaptophysin, synapsin [1, 2]. Synaptophysin is one of the first synaptic vesicular proteins characterized, [3] and is known to have four transmembrane domains that include synaptogyrin and synaptoporin [4]. Synaptophysin is located in the synaptic vesicle of neuron or neuroendocrine cell and is known to be distributed consistently in all neural synapses. It is suggested that synaptophysin promotes formation of highly curved membranes such as synaptic vesicles [5]. It was found in an ultra structural study that synaptophysin forms structure that is similar to connexons [6]. Recently, synaptophysin was reported to regulate kinetics of synaptic vesicular endocytosis in neurons [7].
In our previous study, we investigated different synaptogenic patterns among the various regions of develo ping cerebral cortex and reported differential expression levels of synaptic marker (synaptophysin) between superficial and deep layers of cerebral cortex according to the developmental stages [8]. However, the hippocampus is a major component of vertebrates playing important roles in the consolidation of information and spatial navigation [9-12]. The anatomy of the hippocampal region has been studied intensely in rodents and connections of the hippocampal region including cytoarchitectures also have been well elucidated. The hippocampal region occupies most of the ventroposterior and ventrolateral walls of the cerebral cortex. This region includes six distinct structures: entorhinal cortex, parasubiculum, presubiculum, subiculum proper, fields cornu ammonis (CA) 1-CA3 in Ammon's horn, and dentate gyrus [13]. Among the structures in the hippocampal region, the hippocampus (Ammon's horn and the dentate gyrus) extends anteriorly beneath the corpus callosum to the posterior level of the septal nuclei. Development of synaptic connectivities has been investigated using a variety of electrophysiological methods. In the rat hippocampus, pyramidal cell connections between CA3 and CA1 increase with development [14]. Characteristics of connectivity between GABAergic interneurons and pyramidal cells in the CA1 of hippocampus were reported in the developing hippocampal region [15]. In the developing hippocampus, GABAergic and glutamatergic synapses are formed and mature sequentially [16]. Furthermore, glutamatergic and GABAergic synaptic connectivity was reported to develop very differently in the CA1 region [17].
Nevertheless, investigations of synaptogenesis in the hippocampal region thorough the developmental stages using synaptic markers are few and our previous results are limited to the cortical areas. In this study, we investigate the differential expression levels and patterns of synaptic marker (synaptophysin) in the mouse hippocampal region according to the developmental stages. For this study, we introduced immunofluorescence staining of synaptophysin various structures of hippocampal region. Densitometric analyses for the morphological quantifications were also performed in the areas with positive synaptophysin immunoreactivities to assess statistical significance. The results from this study along with our previous report will lead to understanding different patterns of synaptogenesis among the regions of developing brain and also for estimating the timing of synapse elimination.
C57BL/6 mouse strain was used for immunofluorescence staining. The mice were classified into 3 groups of embryo (embryonic day 17), neonatal (postnatal day 1), and adult (10-12 weeks adult) according to developmental stages.
Brains of embryonic day 17 mice obtained from surgically sacrificed pregnant mouse and neonatal day 1 mice were separated under an operating microscope. The adult mice were anesthetized by ether and perfused transcardially with 0.05 M phosphate buffered saline (PBS, pH 7.4) for 1 minute followed by 4% paraformaldehyde (PFA) in 0.05 M PBS for 10 minutes using peristaltic pump. The enucleated brains were fixed in 4% PFA for 24 hours at 4℃. The brains were then transferred three times to 12%, 16% and 18% sucrose in 0.05 M PBS for 30 minutes at 4℃ respectively for cryoprotection. The fixed brains were immersed in OCT compound (Tissue-Tek, Torrance, CA, USA) as embedding medium and then quick-frozen in isopropanol cooled by liquid nitrogen for 30 seconds. The brains embedded in OCT compound were cut on the coronal plane at 10 µm thicknesses with a cryostat (Leica CM1850, Leica, Wetzlar, Germany) and attached onto gelatin-coated slide. Prior to immunofluorescence staining, the brain sections were stored in deep freezer at -70℃.
For the immunofluorescence staining, rabbit anti-synaptophysin polyclonal antibody (1 : 500, ab14692, Abcam, Cambridge, UK) was used as a synaptic marker. First, brain sections were washed three times in 0.05 M PBS for 5 minutes. Nonspecific reactivity was blocked by adding albumin (Sigma, St. Louis, MO, USA) and 1.5% goat serum (Vector Lab, Burlingame, CA, USA) into the primary antibody diluting solution. After the incubation of the primary antibody for 24 hours at 4℃, the brain sections were rinsed three times with 0.05 M PBS for 5 minutes and incubated in fluorescence tagged secondary antibody (1 : 200, Alexa Fluor 555 goat anti-rabbit IgG, A21424, Invitrogen, Carlsbad, CA, USA) for 90 minutes at room temperature. The brain sections were washed again three times for 5 minutes after the termination of secondary antibody incubation. Finally, nuclei of cultured neurons were counter-stained with DAPI (ABBOT Molecular, Des Plaines, IL, USA) and immediately inspected with the laser con-focal microscope system.
A confocal microscope (LSM-700, Carl Zeiss, Jena, Germany) equipped with associated software of ZEN2009 (ver. 5,5,0,375, Carl Zeiss) was used for the analysis of synaptophysin immunofluorescence staining. Images of the immunostained sections were captured and the densitometric intensity of synaptophysin immunoreactivities in each layer was determined using the ZEN2009 soft ware (Fig. 1C). The synaptophysin immunoreactivities were visualized with 555 nm lasers (red), and nuclear stain was detected with 488 nm lasers (blue) on the same section. We set up the standard master gain of Rodamin fixation at 750, master gain of DAPI fixation at 650. We applied this condition to all the samples to obtain accurate and reliable results on fluorescence intensities and to remove any bias from the manipulation of the confocal microscope. The selected anatomical regions included superficial molecular layer and deep oriens layer in hippocampal region (hippocampus and subiculum proper). To measure synaptophysin immunoreactivities in two layers, the entire areas of interests were selected respectively (Fig. 1B). For the statistical analysis, about 10 different tissue samples were recruited into morphological quantification according to each developmental stage. The average for each variable was calculated, and then the difference of averaged figures was compared between the two layers. All statistical analysis was conducted with the statistical package R-program. For multiple group comparisons, statistical differences were calculated by independent samples t-test. Values of P<0.05 or P<0.001 were considered significant.
In the embryonic and neonatal hippocampus, synaptophysin immunofluorescence was almost defined to cornu ammonis subfields (CA1 and CA3) of hippocampus and subiculum proper in the hippocampal region. However in dentate gyrus, synaptophysin immunoreactivities were insignificant or absent in all developmental stages. According to the immumofluorescence findings, synaptophysin expressions showed various patterns through the layers of developing hippocampus. These expression patterns changed according to the developmental stages of embryo, neonatal, and adult respectively and the detailed findings of synaptophysin expressions are as follows.
In the embryonic and neonatal group, synaptophysin immunoreactivities following neural processes extended perpendicular between pyramidal cell layer and lacunosum molecular layer through radiatum layer (Fig. 2D). Specific synaptophysin immunoreactivities were almost absent in the adult hippocampal region and only homogeneous background immunofluorescence could be found.
Synaptophysin immunoreactivities were different between the layers of the hippocampal region. In embryo, the mean intensity in molecular layer was 1,769.9 whereas the mean intensity in oriens layer was 1,317.1. In neonate, the mean intensity in molecular layer was 1,357.4 whereas the mean intensity in oriens layer was 1,190.5. In adult, the mean intensity in oriens layer was rather higher (1,336.7) than in the molecular layer (1,268.3). Thus the embryo, there was a remarkable difference between deep layer of both oriens and wide superficial molecular layer (Fig. 2A). Neonate hippocampal region also showed similar synaptophysin immunoreactivities. However the neonate, differences between the layers was much less compared to the embryo (Fig. 2B). In the adult group, any significant differences between the layers couldn't be found due to the lack of specific synaptophysin immunoreactivities.
In the statistical analyses based on morphological quantifications, there were significant differences between molecular and oriens layer in the embryo and neonate whereas there wasn't any significant differences in the adult (Fig. 2E).
Along with the difference between the layers of the hippocampal region, synaptophysin immunoreactivities were also various among the groups of developmental stages.
In molecular layer, the mean intensity in embryo was 1,769.9 whereas the mean intensity in neonate (adult) was 1,357.4 (1,268.3). In oriens layer, the mean intensity was lowest in neonate (1,190.5) compared to embryo (1,317.1) or adult (1,336.7). Thus in molecular layer, the difference between embryo and neonate or between embryo and adult was prominent whereas there were no significant differences among the groups in case of oriens layer.
The intensities of synaptophysin immunofluorescence in the layers of the hippocampal region along with the developmental stages were summarized in Table 1.
In the results of this study, we obtained morphological findings on the expression patterns of synaptic marker (synaptophysin) between the layers of the hippocampal region and also according to the developmental stages of embryonic, neonatal, and adult. As described in the introduction, this study was a continuation of previous morphological results in cerebral cortex. It is well known that the hippocampus is connected to the septum, mammillary body of hypothalamus, and the anterior nucleus of thalamus which are involved with emotional behavior. However, the hippocampus is an elaboration of the edge of the cerebral cortex (limbic system) anatomically. The entorhinal cortex which is located in the parahippocampal gyrus is also a part of the hippocampal region because of its anatomical connections and its reciprocal connections with many other parts of the cerebral cortex. Therefore based on the morphological findings in cerebral cortex and hippocampal region, we characterize morphological patterns of neural connections and synaptogenesis according to the developmental stages for the next step.
In the embryonic and neonatal hippocampal region, synaptophysin immunofluorescence was observed mainly in the molecular and oriens layers. Furthermore, the synaptophysin immunoreactivities following neural processes extended perpendicular between pyramidal cell layer and lacunosum molecular layer through radiatum layer. These results seem to be associated with a dendritic structure of pyramidal cells. In the rodent hippocampus, apical dendrites of pyramidal cells extend from the cell body in pyramidal cell layer through the radiatum layer and into the lacunosum molecular layer. The basal dendrites of pyramidal cells also produce another dense tuft in oriens layer. Therefore the differences of synaptophysin immunoreactivities between the layers could be explained by different synaptogenic activities between apical and basal dendrites of pyramidal cells according to the developmental stages.
Unlike CA in hippocampus, synaptophysin immunoreactivities were slight or negligible from the dentate gyrus even in the embryonic and neonatal group. The major input to the dentate gyrus is from entorhinal cortex and there's no direct input from other cortical structures. In the layers of dentate gyrus, granule cells in the middle layer are most prominent and known to project mostly to the interneurons within CA3 subfield. More than 80% of the granule cells are generated after birth in rodents and in humans, it is estimated that granule cells continue being generated not only after birth but also all the way into adulthood [18]. In the study of granule cell migration during rat brain development, the granule cells begin to migrate and settle into the developing dentate gyrus around embryonic day 17 or 18 [19]. Therefore considering numerous nuclei stained with DAPI within the dentate gyrus in all groups, synaptogenic activities from and to the dentate gyrus seem focused on the periods between neonatal and adulthood. Because we used only neonatal day 1 mice for neonatal group at this time, further experiments with the developing stages between neonatal and adulthood will reveal certain morphological synaptogenic activities in dentate gyrus. In the results showing changes of synaptophysin expressions according to the developmental stages, the adult group also possessed significant or even higher intensities than embryonic or neonatal. As shown in the picture of result, only homogeneous background immunofluorescence was detected with no specific synaptophysin immunoreactivities in the hippocampal region of adult group. Therefore it seems to be more reasonable to ignore the values obtained from the adult group although the background intensities in adult group were included for the morphological calculations and statistical analyses. Along with the morphological and many other electrophysiological findings of synaptogenic activities, various factors known to be involved in the neural network formation and plasticity should be also weighed for more detailed and precise interpretation of synaptogenic activities in the cortical and hippocampal region throughout the developmental stages. For example, progesterone, which is known to have a protective effect on damage to the central nervous system, also increased synaptophysin expression in the CA1 region of hippocampus [20], and the amount of synaptic protein expression was reported to differ between male and female through the postnatal periods [21].
In conclusion, we delineated differential synaptophysin expressions between molecular and oriens layers of embryonic and neonatal hippocampus. We also demonstrated decreased synaptophysin expressions from the neonatal group compared to embryonic in both layers. It is not enough to explain synaptogenic findings with only one synaptic marker. However, synaptophysin is known as the most abundant [22] and is expressed ubiquitously in almost all types of synapse forming neurons. Therefore the results from this study along with the previous work from the cortical region will contribute not only to analyzing regional characteristics of synaptogenic activities through developmental stages but also to understanding pathological physiologies of brain development in association with time and regional distinctions of synaptogenic activities.
Figures and Tables
Fig. 1
Confocal densitometric analysis methods for the morphological quantification of synaptophysin in the mice hippocampal region (hippocampus and subiculum proper). (A) Diagram of coronal thin section (10 µm) of the mouse brain. Hippocampal areas in red rectangular region were used for confocal microscopic analysis. (B) Confocal image of coronal sectioned hippocampal region. Synaptophysin volume data were obtained by analyzing this image using the system calculating penetration ratio of light intensity into optical density values. Optical density measurement was obtained by manually positioning the area (white dashed square, superficial molecular layer [Mol] and lacunosum molecular layer [LMol]; white straight square, deep oriens layer [Or]) of immunofluorescence-stained section. (C) ZEN 2009 (ver. 5,5,0,375, Carl Zeiss) software used for image acquisition and analysis. The synaptophysin densities were assayed in relation to the areas outlined, and results were appeared on the analysis software (C). Py, pyramidal cell layer; Rad, radiatum layer.
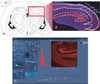
Fig. 2
Immunofluorescence of synaptophysin in the hippocampal region of mouse brain in each developmental stage of embryonic (A), neonatal (B), and adult (C). (D) Magnified picture of (A). Note synaptophysin immunoreactivities connecting pyramidal cell layer (Py) and lacunosum molecular layer (LMol) through radiatum layer (Rad) (arrow). (E) Differential synaptophysin immunoreactivites between molecular layer (Mol) and oriens layers (Or) of hippocampal region in each developmental stages. *P<0.05. n=6-10. Nuclei are counterstained with DAPI. Scale bars=100 µm.
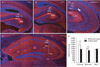
References
1. Eastwood SL, Harrison PJ. Synaptic pathology in the anterior cingulate cortex in schizophrenia and mood disorders. A review and a Western blot study of synaptophysin, GAP-43 and the complexins. Brain Res Bull. 2001. 55:569–578.
2. Jin Y. Synaptogenesis. 2005. Pasadena: WormBook;1–11.
3. Wiedenmann B, Franke WW. Identification and localization of synaptophysin, an integral membrane glycoprotein of Mr 38,000 characteristic of presynaptic vesicles. Cell. 1985. 41:1017–1028.
4. Sudhof TC, Lottspeich F, Greengard P, Mehl E, Jahn R. A synaptic vesicle protein with a novel cytoplasmic domain and four transmembrane regions. Science. 1987. 238:1142–1144.
5. Leube RE. The topogenic fate of the polytopic transmembrane proteins, synaptophysin and connexin, is determined by their membrane-spanning domains. J Cell Sci. 1995. 108(Pt 3):883–894.
6. Arthur CP, Stowell MH. Structure of synaptophysin: a hexameric MARVEL-domain channel protein. Structure. 2007. 15:707–714.
7. Kwon SE, Chapman ER. Synaptophysin regulates the kinetics of synaptic vesicle endocytosis in central neurons. Neuron. 2011. 70:847–854.
8. Pyeon HJ, Lee IL. Differential expression levels of synaptophysin through developmental stages in cerebral cortices of mouse brain. Korean J Phys Anthropol. 2012. 25:55–62.
9. Diana RA, Yonelinas AP, Ranganath C. Imaging recollection and familiarity in the medial temporal lobe: a three-component model. Trends Cogn Sci. 2007. 11:379–386.
10. VanElzakker M, Fevurly RD, Breindel T, Spencer RL. Environmental novelty is associated with a selective increase in Fos expression in the output elements of the hippocampal formation and the perirhinal cortex. Learn Mem. 2008. 15:899–908.
11. Di Gennaro G, Grammaldo LG, Quarato PP, Esposito V, Mascia A, Sparano A, Meldolesi GN, Picardi A. Severe amnesia following bilateral medial temporal lobe damage occurring on two distinct occasions. Neurol Sci. 2006. 27:129–133.
12. Rolls ET, Xiang JZ. Spatial view cells in the primate hippocampus and memory recall. Rev Neurosci. 2006. 17:175–200.
13. Paxinos G, Watson C. The rat brain in stereotaxic coordinates. 1982. Sydney: Academic Press.
14. Hsia AY, Malenka RC, Nicoll RA. Development of excitatory circuitry in the hippocampus. J Neurophysiol. 1998. 79:2013–2024.
15. Groc L, Gustafsson B, Hanse E. Early establishment of multiple release site connectivity between interneurons and pyramidal neurons in the developing hippocampus. Eur J Neurosci. 2003. 17:1873–1880.
16. Ben-Ari Y. Excitatory actions of gaba during development: the nature of the nurture. Nat Rev Neurosci. 2002. 3:728–739.
17. Riebe I, Hanse E. Development of synaptic connectivity onto interneurons in stratum radiatum in the CA1 region of the rat hippocampus. BMC Neurosci. 2012. 13:14.
18. Eriksson PS, Perfilieva E, Bjork-Eriksson T, Alborn AM, Nordborg C, Peterson DA, Gage FH. Neurogenesis in the adult human hippocampus. Nat Med. 1998. 4:1313–1317.
19. Altman J, Bayer SA. Migration and distribution of two populations of hippocampal granule cell precursors during the perinatal and postnatal periods. J Comp Neurol. 1990. 301:365–381.
20. Choi JM, Romeo RD, Brake WG, Bethea CL, Rosenwaks Z, McEwen BS. Estradiol increases pre- and post-synaptic proteins in the CA1 region of the hippocampus in female rhesus macaques (Macaca mulatta). Endocrinology. 2003. 144:4734–4738.
21. Bian C, Zhu K, Guo Q, Xiong Y, Cai W, Zhang J. Sex differences and synchronous development of steroid receptor coactivator-1 and synaptic proteins in the hippocampus of postnatal female and male C57BL/6 mice. Steroids. 2012. 77:149–156.
22. Takamori S, Holt M, Stenius K, Lemke EA, Gronborg M, Riedel D, Urlaub H, Schenck S, Brugger B, Ringler P, Muller SA, Rammner B, Grater F, Hub JS, De Groot BL, Mieskes G, Moriyama Y, Klingauf J, Grubmuller H, Heuser J, Wieland F, Jahn R. Molecular anatomy of a trafficking organelle. Cell. 2006. 127:831–846.