Abstract
Minor variations in the ossicles, foramina and ridges of the cranium have aroused the curiosity of anatomists for many decades. These non-metric variants help us to study the genetic relationships among ancient populations. Since these traits show considerable frequency differences in different populations, they can be used as anthropological characters in epidemiological studies. These variants indirectly reflect the part of underlying genotype of a given population thus implying their usefulness in biological comparisons of related groups. They can be used for the assessment of the existence of the parental structures within a community or as taxonomic indicators. For anthropological studies, the traits should be genetically determined, vary in frequency between different populations and should not show age, sex, and side dependency. The present study was conducted on hundred dry adult human skulls from Northern India. They were sexed and studied for the presence of hyperostotic traits (double hypoglossal canal, jugular foramen bridging, and paracondylar process). Sexual and side dimorphism was observed. None of the traits had shown statistically significant side and sexual dimorphism. Since the dimorphism is exhibited by none of them, it can be postulated that these traits are predominantly under genetic control and can be effectively used for population studies.
Anthropological and paleoanthropological studies of epigenetic cranial traits or non-metrical cranial traits have increased in the last decade. Assessment of discontinuous traits is popular in anthropological studies due to their apparent ease of scoring and quick analysis. Such traits have been used for the investigation of population history and the measurement of biological distances between different populations [1-4]. For usefulness in such studies, the traits should be genetically determined, vary in frequency between different populations and should not show age, sex, and side dependency. The biological considerations of these traits, including ontogeny, asymmetry, sex differences, and intertrait association, have been addressed to assess possible genetic origins [5-12].
The non-metric traits of the skull include hypostatic variants, hyperostotic variants, supernumerary ossicles and variants related to the foramina of nerves and vessels. This work is concerned with hyperostotic variants-double hypoglossal canal, jugular foramen bridging and paracondylar process-their side and sexual dimorphism and their use in anthropological studies. These traits did not show any sexual or side dimorphism (Tables 1-4), reinforcing their independence of side and sex and suggesting their genetic causes and that they can be effectively used in population and anthropological studies.
The study was conducted in the Department of Anatomy, Lady Hardinge Medical College, Delhi on 100 dry adult human skulls (57 males, 33 females, and 10 uncertain) with no deformities or fractures. They were sexed and studied for the presence or absence of hyperostotic traits: double hypoglossal canal (Fig. 1), jugular foramen bridging (Fig. 2), and paracondylar process (Fig. 3). The skulls were sexed by assessing the contour of the supraorbital margin using plasticine casts. The casts' shapes were assessed in cross section on a seven grade scale [13]:
Seventy percent accuracy of sex identification using this method was achieved.
Each trait was noted for its presence or absence in the skulls and chi-square testing assessed side and sex dimorphism. After sexing, the skulls were studied for hyperostotic variants: double hypoglossal canal, jugular foramen bridging, and paracondylar process. The variants were scored and if they occurred only on one side the dimorphism was also noted. The traits' occurrences were coupled with the sex information to show side and sexual dimorphisms, which were assessed by chi-square testing.
Double hypoglossal canal occurred in 3.5% of the right and 7.0% if the left sides of the male skulls; it was bilaterally present in 5.3%. In the female skulls, the incidence was 6.1% on the right, 6.1% on the left, and 3% bilaterally. In the skulls of uncertain sex, its occurrence was 10% on the right side and 10% bilaterally (Table 1, Fig. 4). Jugular foramen bridging occurred in 5.2% on of the males' right sides and 7% of their left sides; its bilateral occurrence was 1.7%. In females, its incidence was 6.1% on the right, 12.1% on the left, and 3% bilaterally. It was recorded in 10% of the uncertainly sexed skulls' right sides but never on their left sides or bilaterally (Table 1, Fig. 4). Paracondylar process was observed in 3.5% of the males' right sides and 1.7% of their left sides; it occurred bilaterally in 7% of the skulls. In females, its incidence was 6.1% on right side and 6.1% bilaterally. In the unsexed skulls, its incidence was 10% on both the right and the left sides individually and also bilaterally (Table 1, Fig. 4).
The incidence of double hypoglossal canal was 10.5% in males and 9.1% in females (Table 2, Fig. 5). Jugular foramen bridging occurred in 7.1% of the male skulls, 12.1% of the female skulls, and 5% of the uncertain skulls (Table 2, Fig. 5). Paramastoid process was found in 9.5% of the male, 9.1% of the female, and 20% unsexed skulls (Table 2, Fig. 5).
Three hyperostotic variants-double hypoglossal canal, jugular foramen bridging and paracondylar process-were assessed for side and sexual dimorphism.
Anterior to the occipital condyle is a hypoglossal canal medial to and below the lower border of the jugular foramen at the junction of the basilar and the lateral parts of the occipital bone. The canal is directed laterally and forwards from the posterior cranial fossa. It may be divided partly or wholly by a spicule of bone and transmits the hypoglossal nerve and meningeal branch of the ascending pharyngeal artery, an emissary vessel connecting the intracranial basilar plexus with the extracranial internal jugular vein [14]. The rootlets of the hypoglossal nerve attached to the medulla run laterally behind the vertebral artery in two bundles perforating the duramater separately opposite the hypoglossal canal in the occipital bone. They unite after traversing it. The canal pierces the anterior part of the occipital condyle and transmits the hypoglossal nerve. Embryologically, the nerve originates from several segments and this may result in the canal being divided into two (Fig. 1).
The bridging termed ponticuli foraminis jugularis is established by the contact of the intrajugular process of the temporal bone situated posterior to the triangular depression with the bony process of the occipital bone projecting either from just above the hypoglossal canal (type I, anterior type) or from a site posterior to the hypoglossal canal (type II, posterior type) [12, 15]. The foramen is divided into a larger, posterolateral compartment (pars venosa) and a smaller anteromedial compartment (pars nervosa). The two parts are separated by a fibrous bridge connecting the jugular spine of the petrous temporal bone to the jugular process of the occipital bone. This bridge may be ossified, dividing the foramen into two compartments. The anteromedial compartment, the pars nervosa, contains the glossopharyngeal nerve and the inferior petrosal sinus. The pars vascularis contains the jugular bulb, vagus, and spinal accessory nerves (Fig. 2).
A bony exostosis of the cranium base projects from the jugular process (lateral to the occipital condyle) towards the transverse process of the atlas. It may vary from a small tubercle to a large elongated process articulating with the transverse process of the first cervical vertebra. The paracondylar process can rarely be fused with the transverse process of the atlas, functionally limiting neck movement. This may present clinical symptoms due to altered posture and restricted motion. A tubercle in this position has been shown to be a constant feature of 21-82 mm embryos. Once established as a morphological character of the individual, a paracondylar process behaves like a hyperostotic trait (Fig. 3).
These traits are characterized by excess ossification over the anomalous conditions, i.e., ossification of cartilage, ligament, duramater or bone. They might be reminiscent of the bony spurs and osteophytes that form in the skeletons of older people and are related to degenerative changes in such as arthritis. This suggests that they achieve expression at different times during postnatal development [5]. However, other variants such as pterygospinous process and paracondylar process show similar frequencies in infants and elderly suggesting their origins are genetic. Jugular foramen bridging has shown similar incidence between Japanese fetal and adult cranial series [12, 15], indicating that almost all the cases of bridging are established by the end of fetal development and suggesting the existence of genetic factors in the expression of these traits. The influence of postnatal development on the manifestation of these traits has been postulated to be negligible and that they can be effectively used in population studies. The isoincidence lines can be constructed for a variant in the same way as isogens on blood group frequency maps, reinforcing the genetic predisposition of these variants [16].
The hyperostotic traits can be regarded as non-metric anthropological markers to measure genetic distinctiveness or divergence between populations. These variants are either clearly present or absent, easily defined and standardized. Hence their scoring and analysis is quick and easy. For population studies, the trait should be genetically determined and vary in frequency between populations; it should not show age, sex or side dependence. Variants' usefulness is markedly reduced if environmental factors significantly affect their expression. In humans, it is difficult to demonstrate the degree of genetic control. However, since the human variants are morphologically analogous to those in mice, the genetic background of non-metric traits in humans can be strongly supported based on the study of mice [17]. The presence or absence of a minor skeletal variant is the epigenetic consequence of interaction among developmental processes controlled by multiple genes with additive effects [17]. Variants' expression can be modified by non-genetic or environmental factors such as deficient maternal diet and other aspects of the prenatal and neonatal environment. Maternal physiology affecting the intrauterine environment and lactation can strongly influence the speed of embryonic and postnatal development, thereby affecting the frequencies of variants in inbred strains [18-20]. However maternal physiology has also been reported to have no overall significant influence when many variants are considered together, although the frequency of a few individual variants may be significantly affected [21].
There is no concencus regarding these traits' environmental and genetic predisposition. They have been reported not to show sexual or side dimorphism [1, 10]; other studies have found significant age and sex differences between traits [6]. A number of variations on the original statistic were later tested and concluded to show very little difference between them [22]. An investigation of only side dimorphism reported asymmetry for these traits [23]. It was concluded that if the traits are strictly under genetic control, both sides should be affected equally. Asymmetry suggests the importance of environmental factors such as nutrition, climate and biomechanical stress in controlling the appearance of such traits. However, others claim that since Trinkaus [23] only counted symmetrical positive scores as symmetry, and neglected bilateral symmetrical absence, his conclusion that asymmetry is common, can be discounted [24].
While several differences, both sexual and side, do occur in human crania, there is little consistency in the occurrence of these dimorphisms. The incidence of a variant may be observed more in females in one sample and more in males of another sample. Such lack of consistency suggests that the variants have genetic, epigenetic and also environmental origins and are well removed from the primary site of gene action [8]. This does not invalidate their usefulness as anthropological tools because if sufficient variants are used, the proportion of the genome represented by them is so much greater than when single gene characters alone are used. Considering population samples with nearly equal numbers of males and females could adequately correct for the frequency differences displaying sexual dimorphism [25]. Male and female samples can be summarized for all the character variants which do not show sexual dimporphism and only male and female samples for those traits that do display sexual dimorphism [26].
It can thus be concluded that hyperostotic variants are predominantly under genetic control and can be used effectively in population studies despite being influenced by environmental factors and exhibiting dimorphism. However, the dimorphism is not statistically significant (Tables 3, 4) and the traits can be inferred to be predominantly under genetic control. Moreover, the small frequency differences associated with age, sex, and side need not in general concern the anthropologist who wishes to maximize his sample for population comparisons by pooling data for all the individuals.
The hypoglossal canal passing as two roots into the occipital bone and emerging as one trunk may be of clinical importance. The nerve roots might get trapped in the occipital bone on one or both sides during ossification, resulting in slight alterations of tongue movement. While not sufficient to casue any major problems, these differences are sufficient to affect speech. There are no reported clinical studies of the entrapment of the hypoglossal nerve in the occipital bone. It is worth considering this variation in elderly patients with minor difficulties in movements of the tongue or speech.
Jugular foramen bridging can press upon the cranial nerves causing nerve palsies (Vernet syndrome) characterized by loss of taste at the posterior third of the tongue (cranial nerve ninth), vocal cord paralysis and dysphasia (cranial nerve X), weakness of sternocleidomastoid and trapezius (cranial nerve XI) [27].
A large paracondylar process may cause vascular insufficiency symptoms due to compression of the vertebral arteries. The third atlanto-occipital joint, if present, may disrupt the biomechanics between the occiput and the atlas resulting in mechanical pain and increased rigidity. The condition may also be associated with an osseous torticolis [28]. The head may adopt an attitude of flexion termed caput obstipum [29]. Paracondylar process may be fused to the transverse process of the atlas leading to restricted movement of the head and neck. Patients might present symptoms of headache in the occipital region radiating to the forehead, dizziness, neck pain, weakness or ataxia. They may suffer from vertigo and disturbances of balance.
Figures and Tables
Fig. 4
Side dimorphism of hyperostotic variants. M, male; F, female; U, unilateral; B, bilateral; RT, right; LT, left; DHC, double hypoglossal canal; JFB, jugular foramen bridging; PP, paracondylar process.
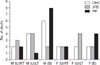
Fig. 5
Sexual dimorphism of hyperostotic variants. DHC, double hypoglossal canal; JFB, jugular foramen bridging; PP, paracondylar process.
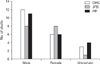
References
1. Carolineberry A, Berry RJ. Epigenetic variation in the human cranium. J Anat. 1967. 101(Pt 2):361–379.
2. De Villiers H. The skull of the South African negro: a biomedical and morphological study. 1968. Johannesburg: Witwatersrand University Press.
3. Dodo Y. Non-metrical cranial traits in the Hokkaido Ainu and the northern Japanese of recent times. J Anthropol Soc Nippon. 1974. 82:31–51.
4. Dodo Y, Ishida H. Population history of Japan as viewed from cranial nonmetric variation. J Anthropol Soc Nippon. 1990. 98:269–287.
5. Ossenberg NS. The influence of artificial cranial deformation on discontinuous morphological traits. Am J Phys Anthropol. 1970. 33:357–371.
6. Corruccini RS. An examination of the meaning of cranial discrete traits for human skeletal biological studies. Am J Phys Anthropol. 1974. 40:425–445.
7. Pucciarelli HM. The influence of experimental deformation on neurocranial wormian bones in rats. Am J Phys Anthropol. 1974. 41:29–37.
8. Berry AC. Factors affecting the incidence of non-metrical skeletal variants. J Anat. 1975. 120(Pt 3):519–535.
9. Gottlieb K. Artificial cranial deformation and the increased complexity of the lambdoid suture. Am J Phys Anthropol. 1978. 48:213–214.
10. Cosseddu GG, Floris G, Vona G. Sex and side differences in the minor non-metrical cranial variants. J Hum Evol. 1979. 8:685–692.
11. Dodo Y. Appearance of bony bridging of the hypoglossal canal during the fetal period. J Anthropol Soc Nippon. 1980. 88:229–238.
12. Dodo Y. Observations on the bony bridging of the jugular foramen in man. J Anat. 1986. 144:153–165.
13. Graw M, Czarnetzki A, Haffner HT. The form of the supraorbital margin as a criterion in identification of sex from the skull: investigations based on modern human skulls. Am J Phys Anthropol. 1999. 108:91–96.
14. Williams PL, Bannister LH, Berry MM, Collins P, Dyson M, Dussek JE, Fergusson MW. Gray's anatomy. 1995. New York: Churchill Livingstone;584.
15. Dodo Y. A population study of the jugular foramen bridging of the human cranium. Am J Phys Anthropol. 1986. 69:15–19.
16. Brothwell DR. The use of non-metrical characters of the skull in differentiating populations. Dtsch Ges Anthropol. 1958. 6:103–109.
17. Grüneberg H. The pathology of development: a study of inherited skeletal disorders in animals. 1963. New York: Wiley.
18. Searle AG. Genetical studies on the skeleton of the mouse. 9. Cases of skeletal variation within pure lines. J Genet. 1954. 52:68–102.
19. Searle AG. Genetical studies on the skeleton of the mouse. 11. The influence of diet variation within pure lines. J Genet. 1954. 52:413–424.
20. Deol MS, Truslove GM. Genetical studies on the skeleton of the mouse XX. Maternal physiology and variation in the skeleton of C57 BL mice. J Genet. 1957. 55:288–312.
21. Howe WL, Parsons PA. Genotype and environment in the determination of minor skeletal variants and body weight in mice. J Embryol Exp Morphol. 1967. 17:283–292.
22. Finnegan M, Cooprider K. Empirical comparison of distance equations using discrete traits. Am J Phys Anthropol. 1978. 49:39–46.
23. Trinkaus E. Bilateral asymmetry of human skeletal non-metric traits. Am J Phys Anthropol. 1978. 49:315–318.
24. Perizonius WR. Non-metric cranial traits: sex difference and age dependence. J Hum Evol. 1979. 8:679–684.
25. Finnegan MJ. Population definition on the northwest coast by analysis of discrete character variation. 1972. Boulder: University of Colorado;PhD Dissertation.
26. Gaherty G. Discrete traits, cranial measurements, and non-biological data in Africa. Am J Phys Anthropol. 1974. 40:136.
27. Hakuba A, Hashi K, Fujitani K, Ikuno H, Nakamura T, Inoue Y. Jugular foramen neurinomas. Surg Neurol. 1979. 11:83–94.
28. Prescher A, Brors D, Adam G. Anatomic and radiologic appearance of several variants of the craniocervical junction. Skull Base Surg. 1996. 6:83–94.
29. Lombardi G. The occipital vertebra. Am J Roentgenol Radium Ther Nucl Med. 1961. 86:260–269.