Abstract
Human epithelial cell lines were utilized to examine the effects of anoxia on cellular growth and metabolism. Three normal human epithelial cells lines (A549, NHBE, and BEAS-2B) as well as a cystic fibrosis cell line (IB3-1) and its mutation corrected cell line (C38) were grown in the presence and absence of oxygen for varying periods of time. Interleukin-8 (IL-8) levels were measured by enzyme-linked immunosorbent assay technique. Cellular metabolism and proliferation were assayed by determining mitochondrial oxidative burst activity by tetrazolium compound reduction. The viability of cells was indirectly measured by lactate dehydrogenase release. A549, NHBE, and BEAS-2B cells cultured in the absence of oxygen showed a progressive decrease in metabolic activity and cell proliferation after one to three days. There was a concomitant increase in IL-8 production. Cell lines from cystic fibrosis (CF) patients did not show a similar detrimental effect of anoxia. However, the IL-8 level was significantly increased only in IB3-1 cells exposed to anoxia after two days. Anoxia appears to affect certain airway epithelial cell lines uniquely with decreased cellular proliferation and a concomitant increased production of a cytokine with neutrophilic chemotactic activity. The increased ability of the CF cell line to respond to anoxia with increased secretion of inflammatory cytokines may contribute to the inflammatory damage seen in CF bronchial airway. This study indicates the need to use different cell lines in in vitro studies investigating the role of epithelial cells in airway inflammation and the effects of environmental influences.
One of the cardinal functions of the lung is to provide sufficient oxygen to meet cellular metabolic demands. However, there may be certain conditions such as chronic obstructive pulmonary diseases, asthma, cystic fibrosis (CF), pulmonary hypertension, and pneumonia in which mucus plugging of the bronchial airways results in impaired ventilation/perfusion relationships and subsequently localized hypoxia and even anoxia. Understanding the implications of anoxia in peripheral airways has not been well studied and is impeded by the lack of adequate animal models.
Because of the difficult access to small airways (<2 mm diameter), there are few in vivo studies of the effects of low oxygen tension. In a murine model of acute respiratory distress syndrome, lipopolysaccharide-treated animals exposed to hypoxia had a significantly increased influx of neutrophils in their bronchoalveolar lavage (BAL) fluid [1], as would be expected with increased neutrophilic chemokine production. Unfortunately, the functional murine interleukin-8 (IL-8) homologues keratinocyte-derived cytokine and macrophage inflammatory protein (MIP) 2 were not measured. Evidence for other cytokines had been found in animals exposed to hypoxia. Madjdpour et al. [2] found increased levels of mRNA for tumor necrosis factor-α, MIP-1 beta, and monocyte chemoattractant protein-1 in the BAL fluid. These cytokines appeared to be macrophage dependent. There is abundant in vitro evidence that hypoxia may aggravate airway inflammation through an effect on immune cells such as macrophages [3] or monocytes [4]. However, detailed studies on hypoxic or anoxic effects on lung epithelial cells are lacking and their effects remain to be elucidated.
Since anoxic effects on airway epithelial cells have not been extensively studied, we examined the inflammatory response, specifically, the production of IL-8, and metabolic activity of alveolar and bronchial epithelial cell lines exposed to anoxia. IL-8 was chosen because of its chemotactic effect on neutrophils, whose presence in the airways may reflect an inflammatory response in many chronic lung conditions such as asthma and cystic fibrosis. These conditions also result in mucus plugging with subsequent distal atelectasis and anoxic conditions.
Epithelial cell lines were originally obtained from the American Type Culture Collection (ATCC, Manassas, VA, USA), grown and passed as suggested by the manufacturer. They were: A549 cells (ATTC CCL-185), which are human alveolar basal epithelial cells from an explanted culture of lung carcinomatous tissue; BEAS-2B cells (ATTC CRL-9609), which is an adenovirus-transformed bronchial epithelial cell line obtained from autopsy of non-cancerous individual; IB3-1 cells (ATCC CRL-2777), which is an immortalized bronchial epithelial CF cell line with the mutation F508/W1282x in the cystic fibrosis transmembrane regulator (CFTR) protein, and C38 cells (ATCC CRL-2779), which is a stable cell line derived from the IB3-1 cells in which the defective CFTR protein has been corrected by transfection with a wild-type adeno-associated viral cystic CFTR gene. An additional normal human bronchial epithelial cell (NHBE) line had been obtained from Lonza Walkersville Inc. (No. CC-2541, Walkersville, MD, USA). NHBE, A549, and BEAS-2B cells were grown in Dulbecco's modified Eagle's medium basal medium supplemented with 10% fetal bovine serum (FBS), penicillin-streptomycin 100 U/ml, glutamine 100 mg/ml, and amphotericin B 2.5 µg/ml at 37℃ in a 5% CO2 incubator. The CF cells were grown in LHC-8 basal medium (12679-015, Invitrogen, Carlsbad, CA, USA), supplemented with 5% FBS in 75 cm2 tissue culture flasks precoated with a mixture of 0.01 mg/ml fibronectin, 0.03 mg/ml bovine collagen type I, and 0.01 mg/ml bovine serum albumin dissolved in LHC-8 medium at 37℃, in a 5% CO2 incubator.
In experiments with A549, BEAS-2B, and NHBE cells, FBS were substituted with an artificial serum supplement CSPC (Sigma, St. Louis, MO, USA) at 10% or no serum supplement. When all cells reached 80% confluence they were passed or transferred to microtiter plates at a concentration of 100,000 cells/well (or 106 cells/ml) and allowed to adhere overnight. The plates were then placed in varying duration in either the regular incubator with regular air consisting of 74% N2, 21% O2, and 5% CO2, or an anoxic environment in which the air was substituted with 95% N2 and 5% CO2. Measurement of the oxygen tension in the incubator at six hours confirms zero oxygen tension.
Both cellular proliferation and metabolic activity were determined by the level of ATP activity in mitochondria by measuring the change in the tetrazolium compound 3-(4,5-dimethylthiazol-2-yl)-5-(3-carboxymethoxyphenyl)-2-(4-sulfophenyl)-2H-tetrazolium (MTS) (CellTiter 96 Aqueous, Promega, Madison, WI, USA) using colorimetric measurements in enzyme-linked immunosorbent assay (ELISA) reader. With the addition of an electron coupling reagent (phenzaine methosulfate or PMS) MTS is bioreduced by cells into a formazan product that is soluble in tissue culture medium and can be quantified by its absorbance at 490 nm. The actual metabolic activity of the cells can be examined by looking at the changes in OD490/OD690 at multiple time points within the first 90 minutes after the addition of the MTS. The slopes of the increase in OD490/OD690 were obtained by the best fit linear curve of at least ten samples and the means were compared among the different cell lines. Cell proliferation can be indirectly determined by measuring the OD490/OD690 four hours after the addition of the MTS. This is the plateau measurement for all the cell lines although some cell lines such as A549 reached it earlier. Confirmation that this measurement represented cell number was indicated by its correlation with DNA synthesis by the cell proliferation BudU assay (Roche Molecular Biochemicals, Mannheim, Germany), performed according to the manufacturer's protocol in one set of experiments. In addition, there was a linear correlation with the number of cells plated (10,000, 25,000, 50,000, and 100,000 cells per well) and their MTS reduction four hours later. Results with the latter cellular concentration are reported. Finally, lactate dehydrogenase (LDH) levels in supernatants were obtained after the incubation period, indicating cell membrane integrity or viability (Tox-7, Sigma-Aldrich).
Supernatants were also withdrawn from the wells and measured for various cytokines, including IL-8 (Biosource, KHC0084, Invitrogen) and transforming growth factor-β (TGF-β; Biosource, Invitrogen), by the ELISA technique.
The response by the different cell lines to anoxia was compared by the Student's paired t-test. Determination of any significant changes over varying time periods were performed using ANOVA.
Mitochondrial activity in the cell lines was examined by measuring the rate of ATPase activity over 90 minutes after one to four days of anoxia. There were varying responses of several epithelial cells (Table 1). For A549 cells, there was an immediate and significant decrease in cellular metabolism after just one day of exposure (P<0.01 by ANOVA). However, BEAS-2B and NHBE cells required two days of anoxic incubation before a significant decrease in mitochondrial activity was observed (P<0.001 by ANOVA). For both CF cell lines, anoxia had no effect and in fact there is some indication that cells thrived in the absence of oxygen.
The detrimental effect of anoxia was most apparent with those cells with the highest level of metabolic activity (that is, the A549 cells). Since atelectasis may also decrease the blood supply of various nutrients to the cells, different media supplements were added to determine if there was any protection to the effects of anoxia. There did not appear to be any lessening with the addition of either 10% fetal calf serum or an artificial media, CSPC, in the media (data not shown).
Although we did not look directly at cell number or DNA concentration, the total amount of living cells can be examined indirectly by comparing the metabolic activity four hours after the addition of MTS since there is a linear relationship between activity and cell number. The greatest effect of anoxia occurred after 3 days of exposure for A549, NHBE, and BEAS-2B cells, with all three showing significant decreases in activity (P<0.001 by ANOVA) (Fig. 1A-C). A549 cells rapidly grew in the first 24 hours and then there was a plateau. However, all cells exposed to anoxia began to decrease their activity after 1 day and then reached a plateau after 3 days. There was no effect when fetal calf serum was added to the media.
In contrast, there did not appear to be any significant effect of anoxia on the proliferation of the two CF cell lines (corrected and uncorrected) (Fig. 1D). This is similar to a lack of effect of their mitochondrial oxidative activity. There also was no increase in cellular permeability as measured by release of LDH in the supernatant. However, the IB3-1 cells under both conditions (anoxia and normoxia) did demonstrate an increase in cell membrane permeability when compared with the C38 cells suggesting a CFTR dependent mechanism. The cell membrane also became more permeable the longer the cells remained in the anoxic environment (Fig. 2).
The production of cytokines was also affected by the presence of anoxia. There were significantly higher levels noted under all circumstances. Interestingly, the highest levels of IL-8 were seen with A549 cells under anoxic conditions and type of growth media did not make any difference. For the NHBE and BEAS-2B cells, the condition which resulted in least metabolic activity resulted in highest IL-8 production (i.e., cells supplemented with CSPC under anoxia) (Fig. 3). Decreased production of IL-8 occurred only in NHBE and BEAS-2B cells grown in anoxia with supplementation of the media with fetal calf serum (FCS). In contrast, there no effect of FCS with anoxic A549 cells. Finally, the levels of TGF-β were uniformly decreased in the presence of anoxia after three days of incubation (data not shown).
There was a progressive increase in IL-8 levels produced by both CF cell lines under anoxic as well as in normal conditions. However, the cells with the mutation in CFTR (i.e., IB3-1) produced significantly much more IL-8 when exposed to anoxia, which were even significantly different after one hour exposure (P<0.01). The difference became more pronounced the longer the cells were exposed. Fig. 4 shows the results of a typical experiment. Anoxic CFTR-corrected (C38) cells also showed an increase in IL-8 production, but not as pronounced as the IB3 cells. IL-8 levels in CF cell lines with corrected CFTR (C38) were statistically higher under anoxia condition compared to normoxic environment (P<0.05).
Hypoxia and certainly anoxic conditions result in different cellular reactions depending on the epithelial cell lines studied. In some cells a marked decrease in metabolic activity was immediately observed whereas more prolonged anoxic exposure was needed for other cell lines. Therefore, the use of A549 cells by Signorelli et al. [5] and Jia et al. [6] may limit their conclusions on the effects of hypoxia to alveolar but not bronchial epithelial cells. These results suggest the need to use several epithelial cell lines in in vitro studies.
However, all cells show significantly higher levels of IL-8 in the absence of oxygen after 2-3 days of culture. Therefore, anoxia appears to affect epithelial cells in two ways; increased production of IL-8 and decreased cell proliferation or metabolism. Such plausible mechanisms may explain the contribution of airway inflammation and of damage of epithelial cells exposed to anoxic conditions such as atelectasis due to mucus plugs or severe peripheral airway inflammation.
CF bronchial epithelial cell lines with CFTR dysfunction (IB3-1) or corrected CFTR (C38) also showed an increased secretion of IL-8 when exposed to anoxia. Other studies of bronchial epithelial cells derived from CF patients also showed low levels of baseline IL-8 production which did not differ between CFTR-corrected and mutated cells [7]. When exposed to various stimulants, such as toll-like receptor agonists, there was a stimulation of IL-8 production [8]. The presence of anoxia was also an effective induction of IL-8 but not TGF-β. In addition, our CF cell lines were unique because unlike the other three cell lines studied there was no apparent effect seen in either metabolic activity or cellular proliferation in anoxia.
Our study indicates that an increased inflammatory reaction by airway epithelial cells in an anoxic environment. Factors such as hypoxic gene expression may play a role in airway epithelial cells responses. In normal airway epithelial cell lines it has been established that hypoxia inducible factor 1 (HIF-1) is a primary driver of hypoxic gene expression in many cells and tissues playing a major role in lung adaptation to hypoxia and anoxia conditions [9-11]. Recent studies have suggested the role of other transcription factors for hypoxia induced lung adaptation, including the activating transcription factor/cAMP response element binding protein (ATF/CREB) family and genes that contain CREB family binding sites, such as ATF-3, endothelin-1 and vascular endothelial growth factor receptor-1. Transcription factors such as these have been found to be activated during hypoxia and have been shown to play a role in hypoxia related pulmonary diseases [12-14] although the exact pathway to airway inflammation has not been clearly elucidated.
The mechanism of hypoxia-induced IL-8 production is complex and dependent on the type of cell. In normoxia, the mitochondria consume approximately 90% of available oxygen through oxidative phosphorylation and ATP generation to satisfy the cell's metabolic needs [5]. The remaining 10% is utilized for other processes including degradation of hypoxia-responsive transcription factors. However, in hypoxia the high affinity of cytochrome c oxidase for oxygen in the mitochondria consumes any available oxygen molecule and allows the increase in certain transcription factors such as HIF. Levels of HIF-1 protein is regulated at the post-translational level by prolyl hydroxylase domain (PHD) proteins, through the hydroxylation of HIF-1 and subsequent proteasomal degradation. The activity of PHD enzymes is inhibited by low oxygen tension, permitting HIF-1 stabilization during hypoxia. Depending on the cell type, these transcriptional factors appear to regulate IL-8 transcription. HIF-1 did not result hypoxic induction of IL-8 in macrophages [15], but AP-1 and CREB were increased. There was no increase in nuclear factor κB (NF-κB) binding activity. On the other hand, hypoxia did increase IL-8, HIF-1, and NF-κB transcriptional activity in prostate cancer cells [16]. In pancreatic cancer cells, AP-1 and NF-κB factors promoted IL-8 gene transcription [17].
Cells with the defective CFTR showed a ten-fold increase in IL-8 release than the cells with the corrected CFTR. The ability of the CF cell lines to produce increased secretion of inflammatory cytokines (e.g., IL-8 with neutrophilic chemotactic activity) may contribute to the enhanced inflammatory response and subsequent damage seen in the airways of CF patients. Although the viability and metabolic activity of both types of CF epithelial cells were not altered in the presence of anoxia, increased cellular permeability as measured by LDH release was observed in the uncorrected CFTR cells.
It has been shown that hypoxia impairs the function of the alveolar epithelium and thus augments edema via reduced clearance of lung liquid. This phenomenon has been suggested to be due to impaired airway epithelial sodium transport related to the decrease in alveolar epithelial Na+-K+-ATPase hydrolytic activity and not secondary to the alteration of cellular energy source when exposed to hypoxia [18-21]. Since CF epithelial cells have an inherent defective transmembrane electrolyte transport system this tendency for increased edema due to impaired Na+ transport in hypoxia may be balanced by the effect of defective Cl- transport by CFTR mutation. Our observation of increased cellular permeability in the uncorrected CFTR cells suggest the impaired Na+ transport supersedes the Cl- movement since the corrected CFTR failed to correct the increased leak of LDH.
It should be noted that studies to investigating the oxygen level in airways distal to mucous plugging are limited. Additional studies should evaluate the level of fluid inflammatory markers (i.e., IL-8) in BAL fluid of selective obstructed airways distal to mucous plugging and compare the amount found in nonobstructed regions of the bronchial tree. In addition, the effects of hypoxia/anoxia on airway permeability and integrity of tight junctions between airway epithelial cells should be further examined. In any case, initiating aggressive airway clearance measures to alleviate areas of atelectasis should result in decreased inflammatory process and improved pulmonary function by decreasing the inflammatory response of airway epithelial cells.
Because of differing results observed depending on the origin of the cells studied, the need to use multiple cell lines is illustrated by these results. In general, respiratory epithelial cells show production of IL-8 upon exposure to anoxia. However, the time courses differ with some cells showing a relative resistance to the detrimental effects on metabolism and proliferation.
Figures and Tables
Fig. 1
Cell proliferation in respiratory epithelial cells after varying exposures to anoxia or normal conditions in fetal bovine serum (FCS)-supplemented or non-supplemented media. Panel (A) shows a typical result for A549 cells; (B) for NHBE cells; and (C) for BEAS-2B cells in various media as indicated. Panel (D) shows typical results for IB3-1 (triangle marks) and C38 (square marks) cells in plain media without FCS. Shown are ratios of optical density (OD) measured at 490 nm divided by the OD measured at 690 nm four hours after the addition of MTS after exposure to anoxia (dotted lines, open marks) or normal oxygen (solid lines, solid marks) environments for varying days (one to four) as indicated.
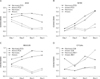
Fig. 2
Cellular cytotoxicity of cystic fibrosis cell lines. Cell viability was measured by the release of lactate dehydrogenase (LDH) by IB3-1 and C38 cells after incubation for varying times in either conditions of anoxia or normal air (normoxia). Shown are ratios of optical density (OD) measured at 490 nm divided by the OD measured at 690 nm after addition of LDH assay mixture within 30 minutes of triplicate wells of a typical experiment of three.
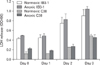
Fig. 3
Production of interleukin-8 (IL-8) in normal respiratory cell lines after three days of exposure to anoxia or normal conditions with different media. Panel (A) are mean levels of IL-8 (pg/ml) with standard error for triplicate wells and three experiments for A549 cells; (B) for NHBE cells; and (C) for BEAS-2B cells. The cells were grown with media supplemented with either no serum supplement (media-plain), 10% artificial nutrients (media-CPSC), or 10% fetal calf serum (media-FCS) as indicated below the bars. The P-values are shown comparing the results under anoxia (solid bars) and normal oxygen (open bars) conditions.
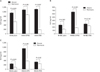
Fig. 4
Production of interleukin-8 (IL-8) in cystic fibrosis cell lines. The IB3-1 cells (with cystic fibrosis transmembrane regulator [CFTR] mutation) (triangle marks) and C38 cells (corrected CFTR) (square marks) were incubated for varying time in either conditions of anoxia (dotted lines and open marks) or normal air (normoxia) (solid lines and solid marks). The day 0 results are the responses of cells exposed to anoxia or normoxia for one hour. Shown are the mean levels of IL-8 (pg/ml) with standard error. The P-values are shown comparing the results of IB3-1 or C38 cells under anoxia to normoxia. The day 0 P-values are P<0.05 for IB3-1 and P=0.1 for C38 cells.
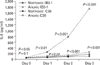
Acknowledgements
Portions of this study were approved by the IRB committees of Long Beach Memorial Medical Center (LBMCC) and Loma Linda University (LLU). The funding of portions of study was provided by the Long Beach Memorial Foundation and Department of Pediatrics at LLU.
References
1. Vuichard D, Ganter MT, Schimmer RC, Suter D, Booy C, Reyes L, Pasch T, Beck-Schimmer B. Hypoxia aggravates lipopolysaccharide-induced lung injury. Clin Exp Immunol. 2005. 141:248–260.
2. Madjdpour C, Jewell UR, Kneller S, Ziegler U, Schwendener R, Booy C, Kläusli L, Pasch T, Schimmer RC, Beck-Schimmer B. Decreased alveolar oxygen induces lung inflammation. Am J Physiol Lung Cell Mol Physiol. 2003. 284:L360–L367.
3. Imtiyaz HZ, Williams EP, Hickey MM, Patel SA, Durham AC, Yuan LJ, Hammond R, Gimotty PA, Keith B, Simon MC. Hypoxia-inducible factor 2alpha regulates macrophage function in mouse models of acute and tumor inflammation. J Clin Invest. 2010. 120:2699–2714.
4. Ghezzi P, Dinarello CA, Bianchi M, Rosandich ME, Repine JE, White CW. Hypoxia increases production of interleukin-1 and tumor necrosis factor by human mononuclear cells. Cytokine. 1991. 3:189–194.
5. Signorelli S, Jennings P, Leonard MO, Pfaller W. Differential effects of hypoxic stress in alveolar epithelial cells and microvascular endothelial cells. Cell Physiol Biochem. 2010. 25:135–144.
6. Jia L, Xu M, Zhen W, Shen X, Zhu Y, Wang W, Wang X. Novel anti-oxidative role of calreticulin in protecting A549 human type II alveolar epithelial cells against hypoxic injury. Am J Physiol Cell Physiol. 2008. 294:C47–C55.
7. Fulcher ML, Gabriel SE, Olsen JC, Tatreau JR, Gentzsch M, Livanos E, Saavedra MT, Salmon P, Randell SH. Novel human bronchial epithelial cell lines for cystic fibrosis research. Am J Physiol Lung Cell Mol Physiol. 2009. 296:L82–L91.
8. Greene CM, Ramsay H, Wells RJ, O'Neill SJ, McElvaney NG. Inhibition of Toll-like receptor 2-mediated interleukin-8 production in cystic fibrosis airway epithelial cells via the alpha7-nicotinic acetylcholine receptor. Mediators Inflamm. 2010. 2010:423241.
9. Cummins EP, Taylor CT. Hypoxia-responsive transcription factors. Pflugers Arch. 2005. 450:363–371.
10. Bracken CP, Whitelaw ML, Peet DJ. The hypoxia-inducible factors: key transcriptional regulators of hypoxic responses. Cell Mol Life Sci. 2003. 60:1376–1393.
11. Shimoda LA, Manalo DJ, Sham JS, Semenza GL, Sylvester JT. Partial HIF-1alpha deficiency impairs pulmonary arterial myocyte electrophysiological responses to hypoxia. Am J Physiol Lung Cell Mol Physiol. 2001. 281:L202–L208.
12. Leonard MO, Howell K, Madden SF, Costello CM, Higgins DG, Taylor CT, McLoughlin P. Hypoxia selectively activates the CREB family of transcription factors in the in vivo lung. Am J Respir Crit Care Med. 2008. 178:977–983.
13. Vaporidi K, Tsatsanis C, Georgopoulos D, Tsichlis PN. Effects of hypoxia and hypercapnia on surfactant protein expression proliferation and apoptosis in A549 alveolar epithelial cells. Life Sci. 2005. 78:284–293.
14. Voelkel NF, Vandivier RW, Tuder RM. Vascular endothelial growth factor in the lung. Am J Physiol Lung Cell Mol Physiol. 2006. 290:L209–L221.
15. Hirani N, Antonicelli F, Strieter RM, Wiesener MS, Ratcliffe PJ, Haslett C, Donnelly SC. The regulation of interleukin-8 by hypoxia in human macrohages: a potential role in the pathogenesis of the acute respiratory distress syndrome (ARDS). Molecular Medicine. 2001. 7:685–697.
16. Maxwell PJ, Gallagher R, Seaton A, Wilson C, Scullin P, Pettigrew J, Stratford IJ, Williams KJ, Johnston PG, Waugh DJ. HIF-1 and NF-kappaB-mediated upregulation of CXCR1 and CXCR2 expression promotes cell survival in hypoxic prostate cancer cells. Oncogene. 2007. 26:7333–7345.
17. Shi Q, Le X, Abbruzzese JL, Wang B, Mujaida N, Matsushima K, Huang S, Xiong Q, Xie K. Cooperation between transcription factor AP-1 and NF-kappaB in the induction of interleukin-8 in human pancreatic adenocarcinoma cells by hypoxia. J Interferon Cytokine Res. 1999. 19:1363–1371.
18. Tomlinson LA, Carpenter TC, Baker EH, Bridges JB, Weil JV. Hypoxia reduces airway epithelial sodium transport in rats. Am J Physiol. 1999. 277(5 Pt 1):L881–L886.
19. Planès C, Escoubet B, Blot-Chabaud M, Friedlander G, Farman N, Clerici C. Hypoxia downregulates expression and activity of epithelial sodium channels in rat alveolar epithelial cells. Am J Respir Cell Mol Biol. 1997. 17:508–518.
20. Suzuki S, Noda M, Sugita M, Ono S, Koike K, Fujimura S. Impairment of transalveolar fluid transport and lung Na(+)-K(+)-ATPase function by hypoxia in rats. J Appl Physiol. 1999. 87:962–968.
21. Mairbäurl H, Mayer K, Kim KJ, Borok Z, Bärtsch P, Crandall ED. Hypoxia decreases active Na transport across primary rat alveolar epithelial cell monolayers. Am J Physiol Lung Cell Mol Physiol. 2002. 282:L659–L665.