Abstract
Wnt/β-catenin signaling plays a critical role in bone formation and regeneration. Dentin and cementum share many similarities with bone in their biochemical compositions and biomechanical properties. Whether Wnt/β-catenin signaling is involved in the dento-alveolar complex formation is unknown. To understand the roles of Wnt/β-catenin signaling in the dento-alveolar complex formation, we generated conditional β-catenin activation mice through intercross of Catnb+/lox(ex3) mice with Col1a1-cre mice. In mutant mice, tooth formation and eruption was disturbed. Lower incisors and molars did not erupt. Bone formation was increased in the mandible but tooth formation was severely disturbed. Hypomineralized dentin was deposited in the crown but roots of molars were extremely short and distorted. In the odontoblasts of mutant molars, expression of dentin matrix proteins was obviously downregulated following the activation of β-catenin whereas that of mineralization inhibitor was increased. Cementum and periodontal ligament were hypoplastic but periodontal space was narrow due to increased alveolar bone formation. While cementum matrix proteins were decreased, bone matrix proteins were increased in the cementum and alveolar bone of mutant mice. These results indicate that local activation of β-catenin in the osteoblasts and odontoblasts leads to aberrant dento-alveolar complex formation. Therefore, appropriate inhibition of Wnt/β-catenin signaling is important for the dento-alveolar complex formation.
Wnt signaling plays essential roles in morphogenesis and cellular differentiation of many tissues during development [1]. Wnt proteins transduce multiple signaling cascades including the Wnt/β-catenin pathway, the Wnt/Ca2+ pathway, and the Wnt/polarity pathway [2]. Among those, the Wnt/β-catenin pathway has been intensely studied. Wnt proteins bind to the frizzled (Frz) transmembrane receptors and the low-density lipoprotein receptor related protein (LRP5/6) co-receptors located on the cell surface to form a receptor trimeric complex, resulting in the inhibition of glycogen synthase kinase-3β (GSK-3β). β-catenin, one of the targets of GSK-3β, acts as a transcriptional co-activator of the T-cell factor (Tcf) and lymphoid enhancer factor (Lef) protein. In the absence of Wnt signaling, β-catenin is a part of cytosolic protein complex that consists of axin, adenomatous polyposis coli, and GSK-3β. GSK phosphorylates β-catenin and leads to its ubiquitination and degradation. However, the binding of Wnt protein to its receptors results in inhibition of GSK and cytosolic accumulation of β-catenin, which is translocated to the nucleus where it interacts with members of the Tcf/Lef family of transcription factors, resulting in complexes that bind to specific response sequences on the promoters of Wnt downstream target genes.
Genetic studies have shown that Wnt/β-catenin signaling plays a critical role in skeletal development and homeostasis [3, 4]. Loss of function mutations of the LRP5 leads to low bone mass accompanied by fractures causing osteoporosis pseudoglioma syndrome in humans and in animal models [5, 6]. LRP5 gain-of-function mutations in humans result in a high bone mass syndrome [7, 8]. In the gene targeted animal models, deletion of β-catenin from differentiated osteoblasts leads to osteopenia, while its stabilization in differentiated osteoblasts leads to high bone mass and failure of tooth eruption [3]. Dento-alveolar complex shares many similarities with bone. However, role of Wnt/β-catenin signaling during its formation has not been reported.
During tooth development, Wnt/β-catenin signaling plays multiple roles in various stages of tooth morphogenesis [9]. Numerous reports have shown that Wnt/β-catenin signaling is required both in the epithelium and mesenchyme for the mediation of epithelial-mesenchymal interaction during tooth morphogenesis. Inactivation of β-catenin in the dental epithelium or mesenchyme leads to arrest of tooth germs at bud stage [10, 11]. In contrast, activation of β-catenin in the dental epithelium causes multiple tooth generation [10, 12]. Activation of β-catenin in the dental mesenchyme leads to premature differentiation of dental pulp cells and produces dentin-like matrix throughout the dental pulp compartment [11]. On the basis of these findings, it is thought that Wnt/β-catenin signaling may also participate in cellular differentiation and matrix formation during tooth formation. Furthermore, Kim et al. [13] recently has found that constitutive stabilization of β-catenin in the dental mesenchyme leads to excessive dentin and cementum formation. The results suggest that local modulation of Wnt/β-catenin signaling plays critical roles in the cellular differentiation and matrix production during dento-alveolar complex formation. However, it remains unclear whether Wnt/β-catenin signaling is involved in the dento-alveolar complex formation. To understand the roles of Wnt/β-catenin signaling in the dento-alveolar complex formation, we generated and analyzed the conditional β-catenin activation mice under the control of Col1a1 promoter. Our data suggest that appropriate inhibition of Wnt/β-catenin signaling is important for the formation of dento-alveolar complex during tooth development.
All experimental procedures were approved by the Animal Welfare Committee of the Chonbuk National University. For stabilization of β-catenin in the dento-alveolar complex, Catnb+/lox(ex3) mice [14] were crossed to Col1a1-cre [15] mice. Genotypes of the mice were determined as previously described [14, 15].
For histological analysis, jaws were dissected and fixed in 4% paraformaldehyde at 4℃ overnight. After rinsing with 0.01 M phosphate buffered saline (PBS), the specimens were decalcified in 10% EDTA/PBS for 2-4 weeks, then dehydrated, embedded in paraffin, and sectioned at a thickness of 7 µm. Slides were stained with hematoxylin and eosin (H&E).
Immunohistochemistry was performed using Histostain Plus Rabbit Primary (DAB) kit (Zymed Laboratories, San Francisco, CA, USA) and goat ImmunoCruz staining system (Santa Cruz Biotechnology Inc., Santa Cruz, CA, USA) following each manufacturer's instructions. The sagittal sections were treated with 3% hydrogen peroxide, and incubated with rabbit polyclonal antibodies against β-catenin (1 : 200, Thermo Scientific, Fremont, CA, USA), dentin sialophosphoprotein (Dspp; 1 : 400, kindly provided by Dr. Larry Fisher), biglycan (Bgn; 1 : 800, Dr. Larry Fisher), dentin matrix protein-1 (Dmp1; 1 : 500, Takara Bio Inc., Shiga, Japan), phosphate regulating endopeptidase homologue on the X chromosome (Phex; 1 : 50, Sigma-Aldrich, St. Louis, MO, USA), tissue-nonspecific alkaline phosphatase (Tnap; 1 : 50, Protein Tech Group, Inc., Chicago, IL, USA), bone sialoprotein (Bsp; 1 : 1,200, Abcam, Cambridge, MA, USA), osteopontin (Opn; 1 : 500, Abcam) and goat polyclonal fibroblast growth factor-23 (Fgf23; 1 : 200, Santa Cruz Biotechnology) antibody.
Dissected mandibles from 4-week-old Col1a1-cre:Catnb+/lox(ex3) (MT) and wild type (WT) mice were examined on a soft X-ray system (SOFTEX CSM-2, Softex Co. Ltd., Kanagawa, Japan). After then, the mandibles were scanned in a desktop scanner (1076 Skyscan Micro-CT, Skyscan, Kontich, Belgium) and analyzed with CTscan software (Skyscan).
Body weights of mice were measured at 3- and 4 weeks-old age. All statistical analyses were done using GraphPad Prism software (GraphPad Software, Inc., La Jolla, CA, USA). Statistical differences were determined by Student's t-test and null hypotheses of no difference were rejected if P-values were less than 0.05.
In the mandible of mouse at P8, β-catenin was localized in the ameloblasts, odontoblasts, and osteoblasts (Fig. 1A). β-galactosidase activities were also observed in the odontoblasts and osteoblasts of the Col1a1-cre:R26R double transgenic mouse at P8 (Fig. 1B). This confirmed that targeting of β-catenin with Col1a1-cre was effective in the formation of dento-alveolar complex. At 4 week-old, height of MT mice was shorter than that of WT mice (Fig. 1C). Body weight was also decreased in the MT mice compared to the WT littermates (Fig. 1D). The mean body weight was 7.77±0.61 g (n=9) and 3.58±1.44 g (n=9) in the WT and MT mice at 3 week-old, respectively. The decrease of body weight was more evident in the 4 week-old MT mice (Fig. 1D). The mean body weight was 13.55±1.42 g (n=9) and 3.73±0.72 g (n=9) in the WT and MT mice at 4 weeks-old, respectively. The decrease of body weight was statistically significant (P<0.05). In genotyping with tail DNA, bands of 720 bps and 600 bps were amplified for cre sequences and deleted allele for β-catenin exon 3 loci (Fig. 1E). In stereoscopic observation of incisors, both of upper and lower incisors were normally erupted in the WT mice. In the MT mice, upper incisors were erupted but lower incisors were not erupted (Fig. 1F-I). Mandibular molars were normally erupted into the oral cavity in WT mice but those of MT mice were not erupted as the same as lower incisors (Fig. 1J, K).
In microradiographic examination, general dimensions of craniofacial skeleton in MT mice were smaller than those in WT littermates (Fig. 2A, B). Mandible of MT mice was severely retarded in growth and mal-occluded. In microtomographic view of mid-sagittal sections of mandible, incisors were shorter and smaller than those of WT mice (Fig. 2C, D). In the molars of MT mice, root formation was severely impaired while those of WT mice were normally formed (Fig. 2E, F). In H&E stained sections, deposition of bone matrix was remarkably increased in the mandible, but was poorly mineralized in the MT mice (Fig. 2G, H). In the MT mice, molars with short roots were formed but were not erupted into the oral cavity. Overall dentin was poorly mineralized. In the consecutive sections of MT mice, β-catenin expression was increased in the odontoblasts and osteoblasts (Fig. 2I, J).
Immunohistochemistry revealed that β-catenin was localized in the differentiated odontoblasts and pulp cells of the mandibular first molar of WT mice, which is restrictedly increased in the odontoblasts of MT mice (Fig. 3A, B). Phex was localized in the differentiated odontoblasts of WT mice, but was clearly absent in the odontoblasts of MT mice except some cells included in dentin matrix (Fig. 3C, D). Dspp was extensively localized in the dentin matrix and odontoblasts of WT mice. But it was obviously decreased in the odontoblasts and predentin except thin mineralized dentin layer of MT mice (Fig. 3E, F). A mineralization inhibitor, Fgf23 was not localized in the odontoblasts as well as dentin matrix of WT mice. Interestingly, Fgf23 was extensively localized in the predentin layer of MT mice (Fig. 3G, H). Bgn was exclusively localized in the thin predentin layer in WT mice, but was significantly decreased in the MT mice (Fig. 3I, J). Dmp1 was localized in the junction area between dentin and predentin both of WT and MT mice. These results suggest that activation of β-catenin in the odontoblasts may disturb the differentiation of odontoblasts and mineralization of dentin matrix.
As described previously, molar roots were short and periodontium was defectively formed in the MT mice. In contrast to WT mice, root dentin was thin and hypomineralized in the MT mice. In addition, periodontal space was narrower due to excessively formed alveolar bone in the MT mice (Fig. 4A, B). In the root dentin of MT mice, Dspp was decreased in the dentin (Fig. 4C, D). In the WT mice, Bsp, Dmp1 and Fgf23 are specifically localized in the matrix of acellular cementum and alveolar bone, which was decreased in the MT mice (Fig. 4E-J). Opn was localized in the cementum and periodontal ligaments of WT mice, but it was also decreased in the cementum and periodontal ligaments of MT mice. From these results, it is suggested that activation of β-catenin in the osteoblasts and cells of periodontium may cause to increase bone formation and root deformities.
In the mandible of MT mice, trabecular spaces were reduced following increase of bone formation (Fig. 5A, B). Bsp immunoreactivity was increased whereas Tnap was decreased in the MT mice (Fig. 5C-F). Phex was strongly expressed in the bone matrix of WT mice but it was nearly absent in the MT mice (Fig. 5G, H). In the mandible of WT mice, Bgn was weakly expressed around osteoblasts. Increased Bgn immunoreactivities were observed around the osteoblasts and osteocytes in the MT mice (Fig. 5I, J). In addition, Dmp1 immunoreactivity was increased in the MT mice (Fig. 5K, L).
It is well known that Wnt/β-catenin signaling plays critical roles in the skeletal development. Although having biochemical similarities with bone, the roles of Wnt/β-catenin signaling in dento-alveolar complex formation are unclear. Here we provide in vivo evidence that Col1a1-cre mediated activation of β-catenin leads to disturbance in tooth eruption and aberrant dento-alveolar complex formation.
Wnt/β-catenin signaling plays important roles in bone formation and maintenance. Gain- or loss-of function mutation of β-catenin in osteoblasts resulted in osteopetrosis and osteoporosis, respectively [3]. The complementary regulation of bone formation is principally controlled by the function of osteoclasts. In present data, Col1a1-cre:Catnb+/lox(ex3) mice showed disturbances in tooth eruption together with increase of alveolar bone mass. These findings indicate that persistent activation of β-catenin in the osteoblasts may cause dysfunction in osteoclasts and result in disturbance of tooth eruption. In fact, several animal models having dysfunction of osteoclasts showed tooth eruption failure together with osteopetrotic bone formation [16-20]. Therefore, it is suggested that osteoclast mediated bone resorption may be essential for potential eruption pathway of developing tooth.
In contrast to well-known function in bone formation, it remains unknown whether Wnt/β-catenin signaling is involved in the dento-alveolar complex formation. Dentin, a major component of tooth, is formed by odontoblasts differentiated from dental mesenchymal cells. It has been well known that dentin is similar with bone in its biochemical composition. However, little is known of the roles of Wnt/β-catenin signaling in dentin formation. In our data, Col1a1-cre:Catnb+/lox(ex3) mice showed aberrant dentin formation in tooth. In mutant molars, odontoblasts were not fully differentiated but prematurely differentiated following continuous activation of β-catenin. This finding is consistent with previous report that Osr2Ires-cre mediated activation of β-catenin in the dental mesenchyme leads to premature differentiation of dental pulp cells [11]. In mutant mice, Phex positive odontoblasts were scarcely found and Dspp was significantly down-regulated in the dentin matrix. In addition, Bgn, a proteoglycan in unmineralized dentin matrix, and an Fgf23, an inhibitor of mineralization, was dramatically upregulated in the predentin. These findings indicate that prematurely differentiated odontoblasts secrete organic dentin matrix but with severely impaired mineralization. From these results, it is suggested that activation of β-catenin in the odontoblasts leads to disturb the differentiation of odontoblasts and mineralization of dentin matrix. Therefore, Wnt/β-catenin signaling may positively regulate matrix production but negatively regulate mineralization during dentinogenesis.
We also demonstrate that Col1a1-cre:Catnb+/lox(ex3) mice exhibit abnormalities in dento-alveolar complex including root deformities, cementum hypoplasia and narrowing of periodontal spaces. Numerous growth factors and transcription factors such as Shh, BMP and Msx, are extensively expressed in the dental epithelium and mesenchyme during root formation [21, 22]. However, little is known about the molecular mechanisms underlying root formation. To date, several studies reported that molecular regulation is necessary for the root elongation in the dental mesenchyme. Nfic, a CTF binding transcription factor, was well known to regulate odontoblast differentiation during root formation. The Nfic knockout mice showed short molar roots [23]. In addition, it has been reported that Smad4 and Ptc1 is also associated with the root elongation [22, 24]. Ablation of these genes leads to short roots in molars together with bone defects. This implies that a common molecular mechanism may be present in the regulation of bone and tooth root formation. There are other possibilities in the disturbances of root formation in Col1a1-cre:Catnb+/lox(ex3) mice. Increased bone mass may disturb the root elongation of molars into alveolar bone during root formation. It is interesting that several animal models related to dysfunction of osteoclasts also showed short roots in molars [16-20].
Cementum also shares many similarities with bone in its biochemical compositions. The accumulating in vitro reports suggest that activation of Wnt signaling is required for the regeneration of periodontal tissue [25]. Recently, it has been shown that constitutive stabilization of β-catenin in the dental mesenchyme leads to excessive cementum formation [13]. Activation of Wnt/β-catenin signaling may stimulate cementoblast differentiation and cementum formation. In contrast, cementum formation was severely disturbed in the Col1a1-cre:Catnb+/lox(ex3) mice. This phenotypic discrepancy between two different β-catenin activation mutant lines may be related with root formation. In the Col1a1-cre:Catnb+/lox(ex3) mice, molar root formation was severely disturbed. Root defects may affect differentiation of cementoblast and cementum formation.
Our results demonstrate that local activation of β-catenin in the dental mesenchyme leads to aberrant dento-alveolar complex formation in vivo. Thus our results indicate that temporospatial regulation of Wnt/β-catenin signaling plays critical roles in cell differentiation, matrix formation and mineralization during dento-alveolar complex formation.
Figures and Tables
Fig. 1
Targeted activation of β-catenin in the dentoalveolar complex. (A) In immunohistochemistry, β-catenin is localized in the Am, Od, and Ob of developing mouse dentoalveolar complex at P8. (B) β-galactosidase activities are also found in the Od and Ob of Col1a1-cre:R26R double transgenic mouse dentoalveolar complex at P8. (C) Col1a1-cre:Catnb+/lox(ex3) mutant mice exhibit severe growth retardation with short height and small body weights. (D) Differences in body weights between WT and MT mice are appeared in 3 weeks after birth and are clearer in the mice at 4 weeks-old. (E) Genotype analysis of mutant mice after intercross with Col1a1-cre and Catnb+/lox(ex3) mice. (F-I) Gross appearance of incisors in WT and MT mice at 4 weeks-old. Both of upper and lower incisors are normally erupted in WT mice, whereas upper incisors are erupted but lower incisors (white arrow) are not erupted in MT mice. (J, K) Molars of lower jaw (white arrowheads) are not erupted into oral cavity in MT mice in contrast to normally erupted in WT mice. Am, ameloblasts; Od, odontoblasts; Ob, osteoblasts; WT, wild type; MT, mutant. *P<0.05. Scale bar=200 µm (A, B).

Fig. 2
Disturbances in tooth formation and eruption failure of molars in Col1a1-cre:Catnb+/lox(ex3) mice. (A, B) In microradiography, general dimensions of craniofacial skeleton in MT mice are smaller than those in WT littermates. Particularly, mandible of MT is severely retarded and resulted in malocclusion. (C, D) In the mid-sagittal view, mandibular incisor is shorter and smaller than that of WT mice. (E, F) Molars of MT mice are impacted within mandible and root formation is impaired while those of WT mice are normally formed and erupted into the oral cavity. (G, H) In H&E-stained sagittal sections of the mandibles, bone deposition is remarkably increased and molars are not erupted in the MT mice. (I, J) In MT mice, β-catenin expression is upregulated in the odontoblasts and osteoblasts. MT, mutant; WT, wild type; H&E, hematoxylin and eosin. Scale bar=100 µm (G-J).
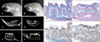
Fig. 3
Mineralization defects in dentin of Col1a1-cre:Catnb+/lox(ex3) mice. (A, B) Immunohistochemistry reveal that β-catenin is localized in the Od and pulp cells of mandibular first molar of WT mice, which is restrictedly increased in the odontoblasts of MT mice. (C, D) Phex is localized in the odontoblasts of WT mice, but which is clearly reduced in the odontoblasts of MT mice. (E, F) In WT mice, Dspp is localized in the dentin as well as odontoblasts, but it is obviously decreased in the predentin and odontoblasts of MT mice. Dspp remains only in thin mineralized dentin of MT mice. (G, H) Localization of Fgf23 is not found in the dentin and odontoblasts of WT mice but which is clearly observed in the predentin of MT mice. (I-L) Bgn and Dmp1 are mainly localized in the predentin and a part of dentin in WT mice, which also decreased in the predentin of MT mice. Od, odontoblasts; WT, wild type; MT, mutant; Phex, phosphate regulating endopeptidase homologue on the X chromosome; Dspp, dentin sialophosphoprotein; Fgf23, fibroblast growth factor-23; Bgn, biglycan; Dmp1, dentin matrix protein-1. Scale bar=100 µm (A-L).
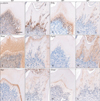
Fig. 4
Deformities in the roots and periodontium of Col1a1-cre:Catnb+/lox(ex3) mice. (A, B) In contrast to WT mice, RD is thin and hypomineralized in MT mice. In addition, PS is narrower due to excessive formation of alveolar bone in MT mice. (C, D) In the RD of MT mice, Dspp is decreased in the dentin. (E-J) In WT mice, Bsp, Dmp1 and Fgf23 are specifically localized in the matrix of acellular cementum (arrows) and alveolar bone, which is decreased in the MT mice. (K, L) Osteopontin is localized in the cementum and periodontal ligaments of WT mice. It is also decreased in the cementum and periodontal ligaments of MT mice. WT, wild type; RD, root dentin; H&E, hematoxylin and eosin; MT, mutant; PS, periodontal space; Dspp, dentin sialophosphoprotein; Bsp, bone sialoprotein; Dmp1, dentin matrix protein-1; Fgf23, fibroblast growth factor-23; Opn, osteopontin. Scale bar=50 µm (A-L).
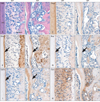
Fig. 5
Molecular changes related with increased bone mass in the mandibles of Col1a1-cre:Catnb+/lox(ex3) mice. (A, B) In the mandible of MT mice, bone mass is increased but number of osteoblasts are decreased to compare with those of WT mice. (C-F) Bsp expression is slightly increased with increase of bone mass, but Tnap is clearly decreased in the MT. (G-J) Bgn is significantly increased in the mandible of MT mice, while Phex is almost disappeared in MT mice. (K, L) Increased expression of Dmp1 is also observed in MT mice. WT, wild type; H&E, hamatoxylin and eosin; MT, mutant; Bsp, bone sialoprotein; Tnap, tissue-nonspecific alkaline phosphatase; Bgn, biglycan; Phex, phosphate regulating endopeptidase homologue on the X chromosome; Dmp1, dentin matrix protein-1. Scale bar=50 µm (A-L).
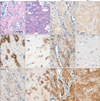
Acknowledgements
We thank Dr. L. Fisher for providing antibodies for Bgn and Dspp. This work was supported by the National Research Foundation of Korea (NRF) grant funded by the Korea government (MEST) (No. 2009-0085733).
References
1. Logan CY, Nusse R. The Wnt signaling pathway in development and disease. Annu Rev Cell Dev Biol. 2004. 20:781–810.
2. Reya T, Clevers H. Wnt signaling in stem cells and cancer. Nature. 2005. 434:843–850.
3. Glass DA 2nd, Bialek P, Ahn JD, Starbuck M, Patel MS, Clevers H, Taketo MM, Long F, McMahon AP, Lang RA, Karsenty G. Canonical Wnt signaling in differentiated osteoblasts controls osteoclast differentiation. Dev Cell. 2005. 8:751–764.
4. Holmen SL, Zylstra CR, Mukherjee A, Sigler RE, Faugere MC, Bouxsein ML, Deng L, Clemens TL, Williams BO. Essential role of beta-catenin in postnatal bone acquisition. J Biol Chem. 2005. 280:21162–21168.
5. Gong Y, Slee RB, Fukai N, Rawadi G, Roman-Roman S, Reginato AM, Wang H, Cundy T, Glorieux FH, Lev D, Zacharin M, Oexle K, Marcelino J, Suwairi W, Heeger S, Sabatakos G, Apte S, Adkins WN, Allgrove J, Arslan-Kirchner M, Batch JA, Beighton P, Black GC, Boles RG, Boon LM, Borrone C, Brunner HG, Carle GF, Dallapiccola B, De Paepe A, Floege B, Halfhide ML, Hall B, Hennekam RC, Hirose T, Jans A, Jüppner H, Kim CA, Keppler-Noreuil K, Kohlschuetter A, LaCombe D, Lambert M, Lemyre E, Letteboer T, Peltonen L, Ramesar RS, Romanengo M, Somer H, Steichen-Gersdorf E, Steinmann B, Sullivan B, Superti-Furga A, Swoboda W, van den Boogaard MJ, Van Hul W, Vikkula M, Votruba M, Zabel B, Garcia T, Baron R, Olsen BR, Warman ML. Osteoporosis-Pseudoglioma Syndrome Collaborative Group. LDL receptor-related protein 5 (LRP5) affects bone accrual and eye development. Cell. 2001. 107:513–523.
6. Kato M, Patel MS, Levasseur R, Lobov I, Chang BH, Glass DA 2nd, Hartmann C, Li L, Hwang TH, Brayton CF, Lang RA, Karsenty G, Chan L. Cbfa1-independent decrease in osteoblast proliferation, osteopenia, and persistent embryonic eye vascularization in mice deficient in Lrp5, a Wnt coreceptor. J Cell Biol. 2002. 157:303–314.
7. Boyden LM, Mao J, Belsky J, Mitzner L, Farhi A, Mitnick MA, Wu D, Insogna K, Lifton RP. High bone density due to a mutation in LDL-receptor-related protein 5. N Engl J Med. 2002. 346:1513–1521.
8. Little RD, Carulli JP, Del Mastro RG, Dupuis J, Osborne M, Folz C, Manning SP, Swain PM, Zhao SC, Eustace B, Lappe MM, Spitzer L, Zweier S, Braunschweiger K, Benchekroun Y, Hu X, Adair R, Chee L, FitzGerald MG, Tulig C, Caruso A, Tzellas N, Bawa A, Franklin B, McGuire S, Nogues X, Gong G, Allen KM, Anisowicz A, Morales AJ, Lomedico PT, Recker SM, Van Eerdewegh P, Recker RR, Johnson ML. A mutation in the LDL receptor-related protein 5 gene results in the autosomal dominant high-bone-mass trait. Am J Hum Genet. 2002. 70:11–19.
9. Liu F, Millar SE. Wnt/beta-catenin signaling in oral tissue development and disease. J Dent Res. 2010. 89:318–330.
10. Liu F, Chu EY, Watt B, Zhang Y, Gallant NM, Andl T, Yang SH, Lu MM, Piccolo S, Schmidt-Ullrich R, Taketo MM, Morrisey EE, Atit R, Dlugosz AA, Millar SE. Wnt/beta-catenin signaling directs multiple stages of tooth morphogenesis. Dev Biol. 2008. 313:210–224.
11. Chen J, Lan Y, Baek JA, Gao Y, Jiang R. Wnt/beta-catenin signaling plays an essential role in activation of odontogenic mesenchyme during early tooth development. Dev Biol. 2009. 334:174–185.
12. Järvinen E, Salazar-Ciudad I, Birchmeier W, Taketo MM, Jernvall J, Thesleff I. Continuous tooth generation in mouse is induced by activated epithelial Wnt/beta-catenin signaling. Proc Natl Acad Sci U S A. 2006. 103:18627–18632.
13. Kim TH, Lee JY, Baek JA, Lee JC, Yang X, Taketo MM, Jiang R, Cho ES. Constitutive stabilization of β-catenin in the dental mesenchyme leads to excessive dentin and cementum formation. Biochem Biophys Res Commun. 2011. 412:549–555.
14. Harada N, Tamai Y, Ishikawa T, Sauer B, Takaku K, Oshima M, Taketo MM. Intestinal polyposis in mice with a dominant stable mutation of the beta-catenin gene. EMBO J. 1999. 18:5931–5942.
15. Dacquin R, Starbuck M, Schinke T, Karsenty G. Mouse alpha1 (I)-collagen promoter is the best known promoter to drive efficient Cre recombinase expression in osteoblast. Dev Dyn. 2002. 224:245–251.
16. Tiffee JC, Xing L, Nilsson S, Boyce BF. Dental abnormalities associated with failure of tooth eruption in src knockout and op/op mice. Calcif Tissue Int. 1999. 65:53–58.
17. Kong YY, Yoshida H, Sarosi I, Tan HL, Timms E, Capparelli C, Morony S, Oliveira-dos-Santos AJ, Van G, Itie A, Khoo W, Wakeham A, Dunstan CR, Lacey DL, Mak TW, Boyle WJ, Penninger JM. OPGL is a key regulator of osteoclastogenesis, lymphocyte development and lymph-node organogenesis. Nature. 1999. 397:315–323.
18. Li J, Sarosi I, Yan XQ, Morony S, Capparelli C, Tan HL, McCabe S, Elliott R, Scully S, Van G, Kaufman S, Juan SC, Sun Y, Tarpley J, Martin L, Christensen K, McCabe J, Kostenuik P, Hsu H, Fletcher F, Dunstan CR, Lacey DL, Boyle WJ. RANK is the intrinsic hematopoietic cell surface receptor that controls osteoclastogenesis and regulation of bone mass and calcium metabolism. Proc Natl Acad Sci U S A. 2000. 97:1566–1571.
19. Kim N, Odgren PR, Kim DK, Marks SC Jr, Choi Y. Diverse roles of the tumor necrosis factor family member TRANCE in skeletal physiology revealed by TRANCE deficiency and partial rescue by a lymphocyte-expressed TRANCE transgene. Proc Natl Acad Sci U S A. 2000. 97:10905–10910.
20. Lu X, Rios HF, Jiang B, Xing L, Kadlcek R, Greenfield EM, Luo G, Feng JQ. A new osteopetrosis mutant mouse strain (ntl) with odontoma-like proliferations and lack of tooth roots. Eur J Oral Sci. 2009. 117:625–635.
21. Yamashiro T, Tummers M, Thesleff I. Expression of bone morphogenetic proteins and Msx genes during root formation. J Dent Res. 2003. 82:172–176.
22. Nakatomi M, Morita I, Eto K, Ota MS. Sonic hedgehog signaling is important in tooth root development. J Dent Res. 2006. 85:427–431.
23. Steele-Perkins G, Butz KG, Lyons GE, Zeichner-David M, Kim HJ, Cho MI, Gronostajski RM. Essential role for NFI-C/CTF transcription-replication factor in tooth root development. Mol Cell Biol. 2003. 23:1075–1084.
24. Gao Y, Yang G, Weng T, Du J, Wang X, Zhou J, Wang S, Yang X. Disruption of Smad4 in odontoblasts causes multiple keratocystic odontogenic tumors and tooth malformation in mice. Mol Cell Biol. 2009. 29:5941–5951.
25. Nemoto E, Koshikawa Y, Kanaya S, Tsuchiya M, Tamura M, Somerman MJ, Shimauchi H. Wnt signaling inhibits cementoblast differentiation and promotes proliferation. Bone. 2009. 44:805–812.