Abstract
Ciliary neurotrophic factor (CNTF) is well known as a growth/survival factor of neuronal tissue. We investigated the expression of CNTF and its specific receptor alpha (CNTFRα) in a unilateral ureteral obstruction (UUO) model. Complete UUO was produced by left ureteral ligation in Sprague-Dawley rats. The animals were sacrificed on days 1, 3, 5, 7, 14, 21, and 28 after UUO. The kidneys were fixed, and processed for both immunohistochemistry and in situ hybridization. CNTF immunoreactivity in sham-operated kidneys was observed only in the descending thin limb (DTL) of the loop of Henle. In UUO kidneys, CNTF expression was induced in the S3 segment (S3s) of the proximal tubule from day 1, and progressively expanded into the entire S3s and a part of the convoluted proximal tubules, distal tubules (DT), and glomerular parietal epithelium up to day 7. Upregulated CNTF expression was maintained to day 28. From day 14, the inner medullary collecting duct showed weak CNTF immunoreactivity. The CNTFRα mRNA hybridization signal in sham-operated kidneys was weakly detected in the DTL, DT, medullary thick ascending limb, and in a few S3s cells. After UUO, CNTFRα mRNA expression increased progressively in both the renal cortex and the medulla up to day 7 and increased expression was maintained until day 28. The results suggest that the S3s may be the principal induction site for CNTF in response to renal injury, and that CNTF may play a role in chronic renal injury.
Ciliary neurotrophic factor (CNTF) was originally identified as a trophic molecule for the survival of embryonic chicken ciliary neurons in vitro [1]. Subsequent studies have shown that CNTF is a neuronal growth factor with a regulatory role in local neuronal healing and regeneration [2, 3]. Additionally, CNTF plays an important role regulating complex cellular processes such as gene activation, proliferation and differentiation, and regeneration, particularly in neuronal tissue [4].
CNTF is a member of the interleukin-6 (IL-6) family of cytokines that include leukemia inhibitory factor, IL-6, interleukin-11, and oncostatin M (OSM) [5]. Unlike other cytokines, IL-6-family cytokines use two kinds of common receptor subunits, gp130 and leukemia inhibitory factor receptor, as well as the CNTF-specific receptor alpha (CNTFRα) [6].
CNTF and CNTFRα are expressed predominantly in neuronal tissue, but CNTF mRNA and protein are also widely expressed in non-neuronal tissue such as the heart, lungs, liver, kidneys, and testes [7, 8]. Apart from neuronal tissue, CNTF is most abundant in the kidney, suggesting that it may play a particular role in the kidney, but its role and expression in the kidney are yet very limited.
The kidney consists of several types of renal tubules. These renal tubules produce several kinds of growth factors, cytokines, and vasoactive substances in response to renal injury [9]. These products act in a coordinated manner to restore renal structure and function after injury. Until now, only a small number of growth factors have been reported from the kidney, but additional growth factors are believed to be produced and involved in renal injury.
Our previous study [10] demonstrated that CNTF is induced mainly in the regenerating proximal tubules in the outer stripe of the outer medulla (OSOM) which are most vulnerable to ischemia-reperfusion injury. The results of that study suggested that CNTF may play a role as a growth factor in renal tissue repair. Based on these findings, we investigated CNTF and CNTFRα expression in a model of unilateral ureteral obstruction (UUO) that results in tubular injury throughout the entire kidney.
Male Sprague-Dawley rats (weight, 250-300 g; Orient Bio Co., Seoul, Korea) were used. Animals were anesthetized with an intraperitoneal injection of ketamine (75 mg/kg body wt). After the abdomen was opened with a midline incision, UUO was performed by ligating the left ureter with 4.0 silk. Sham-operated animals were used to obtain control kidneys, and rats were killed at days 1, 3, 5, 7, 14, 21, and 28 after the operation. In total, we used 84 animals; two sham and 10 UUO, five for a morphological study, and five for immunoblotting were included at each time point.
The kidneys were perfused with phosphate-buffered saline (PBS) briefly through the abdominal aorta and subsequently were fixed by in vivo perfusion with a periodate-lysine-paraformaldehyde (PLP) solution for 10 minutes. They were cut into sagittal slices and then immersed in PLP overnight at 4℃. After a rinse in PBS, the tissues were dehydrated in a graded ethanol series, embedded in wax (polyethylene glycol 400 disterate, Polysciences Inc., Warrington, PA, USA), and 5-µm thick sections were prepared for in situ hybridization. PLP-fixed tissues were cut on a vibratome (Lancer Vibratomes Series 1000, Technical Products International, St. Louis, MO, USA) to a thickness of 50 µm for immunohistochemistry.
Immunohistochemistry using a pre-embedded method was conducted, as described previously [11]. The 50-µm thick vibratome sections were washed with 50 mM NH4Cl in PBS. Before incubation with a primary antibody, the tissue sections were incubated for 3 hours with PBS containing 1% bovine serum albumin, 0.05% saponin, and 0.2% gelatin (solution A). Tissue sections were then incubated overnight at 4℃ with a polyclonal antibody against CNTF (1 : 1,500, Cedarlane Lab., Burlington, ON, Canada) in PBS containing 1% bovine serum albumin (solution B). After washes with solution A, the tissue sections were incubated for 2 hours with peroxidase-conjugated donkey anti-rabbit IgG Fab fragment (Jackson ImmunoResearch Laboratories, West Grove, PA, USA), diluted 1 : 100 in solution B. The tissues were then rinsed, first in solution A and subsequently in 0.05 M Tris buffer (pH 7.6). The sections were incubated in 0.1% 3,3'-diaminobenzidine in 0.05 M Tris buffer for 5 minutes to detect horseradish peroxidase, after which H2O2 was added to a final concentration of 0.01%, and the incubation was continued for 10 minutes. After the sections were washed with 0.05 M Tris buffer, they were dehydrated in a graded ethanol series and embedded in Epon-812. The embedded 50-µm thick sections were examined, and 1-µm semi-thin sections were cut and photographed with an Olympus photomicroscope (Tokyo, Japan) equipped with differential-interference contrast optics.
The cortical, outer medullary, and inner medullary renal tissues were separated from PBS-perfused kidneys and used for protein extraction. Tissues were homogenized in a buffer containing 20 mM Tris-HCl (pH 7.6), 150 mM NaCl, 0.5% (w/v) sodium deoxycholate, 1% (v/v) Triton X-100, 0.1% (v/v) sodium dodecyl sulfate (SDS), 0.02% (v/v) sodium azide, 1 mM ethylenediaminetetraacetic acid fresh leupeptin (1 µg/ml), and phenylmethylsulfonyl fluoride (1 mM). Homogenates were centrifuged at 16,000 ×g for 15 minutes at 4℃, and protein concentrations were determined in supernatants using the Bradford method protein microassay (Bio-Rad, Hercules, CA, USA). Homogenates were boiled for 5 minutes in Laemmli sample buffer. Proteins were separated by SDS-polyacrylamide gel electrophoresis on 15% polyacrylamide gels and were electroblotted onto Bio-Blot nitrocellulose membranes (Costar, Cambridge, MA, USA). Nonspecific binding was blocked by incubating the blots for 1 hour in 5% (w/v) nonfat milk. CNTF protein was detected with a polyclonal anti-CNTF antibody (1 : 15,000, Cedarlane Lab.) with an overnight incubation. The primary antibody incubation was followed by six washes with PBS containing 0.1% Tween 20. The blots were then incubated with peroxidase-conjugated donkey anti-rabbit CNTF IgG for 2 hours. Antibody-reactive protein was detected using an enhanced chemiluminescence kit (Amersham Life Science, Buckinghamshire, UK). Densitometric analysis was performed using Imagemaster VDS software (Pharmacia Biotech, Piscataway, NJ, USA).
Total RNA was extracted from the kidney using TRIZOL reagent (Life Technologies Inc., Carlsbad, CA, USA), and reverse transcribed using the oligo(dT) primer and Superscript II RNase H-reverse transcriptase (Life Technologies), followed by polymerase chain reaction (PCR)-amplification using specific primers (sense, GCTGTGACCTGGAGGGTAAA; antisense, GTGATAGCCGTGGTGTTGTG; probe length, 425 bp; Gene Bank S54212). The reverse trancscription-PCR product was cloned into the T-Easy Vector (Promega, Madison, WI, USA) and sequenced. The digoxygenin (DIG)-labeled sense and antisense CNTFRα riboprobes were prepared by in vitro transcription using a DIG RNA labeling kit (Boehringer, Mannheim, Germany). The specificity of the antisense CNTFRα riboprobe was confirmed by Northern blot (by Prof. JH Youn, Dept. Anatomy, Hanyang University, Medical College, Korea).
After dewaxing, the sections were treated in 0.2 N HCl for 10 minutes and incubated in 20 µg/ml pepsin (0.1 N HCl) for 10 minutes at room temperature, followed by three washes with PBS. Prehybridization and hybridization steps were carried out at 58℃ for 1 hour and 15 hours, respectively. The prehybridization buffer was composed of 50% formamide, 4× sodium chloride/sodium citrate, 10% dextran sulfate, 1× Denhardt's solution, and 1 µg/µl salmon sperm DNA. The hybridization buffer was identical to the prehybridization buffer except that salmon sperm DNA was substituted with 400 ng/µl CNTFRα riboprobe. After posthybridization washing, the sections were incubated with anti-DIG antiserum conjugated with alkaline phosphatase (Boehringer), and histochemical detection was performed using a 4-nitroblue tetrazolium chloride/5-bromo-4-chloro-3-indolyl-phosphate mixture (Boehringer).
From UUO day 21, the length of the outer and inner medulla including renal papilla decreased extremely (Figs. 1G, H, 5G, H). However, many cortical tubular cells and glomeruli maintained their normal structure until day 28 (Fig. 2F).
No CNTF immunoreactivity was detected in the cortex or OSOM in sham-operated rat kidneys (Figs. 1A, 2A). CNTF immunoreactivity was observed only in the tubular profiles in the inner stripe of the outer medulla (ISOM) and the proximal half of the inner medulla (IM) (Fig. 1A); that is, the descending thin limb (DTL) of the loop of Henle (Figs. 2B, 3A).
In the UUO rats, CNTF expression began to appear in the OSOM on day 1 (Figs. 1B, 2C). On day 3, CNTF expression extended to the cortex along the medullary ray and progressively expanded into the cortex and the OSOM up to day 7 (Fig. 1C-E). Subsequently, CNTF expression decreased slightly, but upregulated CNTF expression was maintained until day 28, particularly in the OSOM (Fig. 1H).
Strong CNTF expression was observed partly in convoluted proximal tubules (cPT) and distal tubules (DT) of the cortex in UUO rat kidneys from days 3 to 14 (Fig. 2D), but with weak intensity on days 21 and 28 (Fig. 3C). Furthermore, the parietal epithelial cells (PEC) of Bowman's capsule (Fig. 3C) were CNTF immunoreactive, but CNTF immunoreactivity was not observed in the glomerulus. Strong induction of the CNTF protein was observed in most of the S3 segments (S3s) of the PTs in the OSOM (Fig. 2C, E) after UUO. The CNTF-positive cells of the S3s (arrows in Fig. 3B) usually had poor or no brush borders, in contrast to CNTF-negative cells (arrowheads in Fig. 3B) with well-developed brush borders. In the ISOM, no CNTF-positive tubules were detected except in the DTL, which showed no change in CNTF immunoreactivity throughout the experiment (Fig. 1A-H). No CNTF-positive tubules were detected in the IM except in the DTL (Fig. 3D). However, from day 14, weak to moderate CNTF expression began to appear in the inner medullary collecting duct (IMCD) (Fig. 3E, F), and such CNTF expression increased until day 28.
CNTF protein expression in the cortex and OM began to increase on days 1 to 3 and was maximal on days 7 to 14 after UUO. It then decreased slightly but sustained high expression up to day 28 (Fig. 4A, B). CNTF protein expression in the IM increased slightly (Fig. 4C).
A very faint CNTF protein band was detected in sham-operated rat kidney cortex (Fig. 4A). CNTF protein began to increase on day 1 and was maximum on day 14 in UUO rats. The relative optical densities in each lane were as follows: day 1, 507%; day 3, 2,212%; day 5, 6,978%; day 7, 9,534%; day 14, 10,467%; day 21, 9,701%; and day 28, 8,553%. The CNTF expression pattern in the OM was similar to that of the cortex (Fig. 4B). The relative optical densities of the CNTF protein were as follows: day 1, 104%; day 3, 130%; day 5, 155%; day 7, 163%; day 14, 149%; day 21, 143%; and day 28, 128%. The relative optical densities of the CNTF protein in the IM (Fig. 4C) were as follows: day 1, 101%; day 3, 103%; day 5, 149%; day 7, 121%; day 14, 121%; day 21, 133%; and day 28, 114%.
Northern blot using P32-labeled antisense CNTFRα revealed a strong band between 18S and 28S (Fig. 8), which was identical with previous results [12]. After confirming the specificity of our riboprobe, we used a non-isotope DIG labeling method for in situ hybridization.
In sham-operated rat kidneys, the CNTFRα mRNA hybridization signal was detected weakly in the DTL, the thick ascending limb (TAL), the DT, and in some S3s cells (Fig. 6A, B) but was hardly expressed in the IM (Figs. 5A, 6C).
CNTFRα mRNA expression began to increase in the TAL (Fig. 5B) as early as day 1 in UUO rats, and CNTFRα mRNA expression increased in almost all parts of kidney, including the IM, up to day 7 (Fig. 5C-E). Thereafter, the CNTFRα mRNA signal decreased slightly but remained upregulated until day 28 (Fig. 5H).
The CNTFRα mRNA signal was partly observed in the cPT, the PEC with moderate intensity, and many DT with strong intensity in the cortex of UUO rats (Fig. 7A). However, the CNTFRα signal decreased markedly in the outer cortex on day 28 (Fig. 5H). The most prominent increase in the signal (Fig. 5B-E) occurred in the S3s and TAL of the ISOM and OSOM (Fig. 6D, F). This increased expression was maintained until day 28 (Fig. 7C, E). The OMCD showed moderately induced CNTFRα expression (Figs. 6F, 7C-E). The CNTFRα mRNA signal increased from day 1 in the IMCD, and such induction was observed in all experimental groups including the day-28 group (Figs. 6E, F, 7B, F).
In our previous study [10], we reported that the S3s were the earliest and principal induction site for CNTF after an ischemia-reperfusion injury. The S3s are known to be the most vulnerable to ischemia-reperfusion injury. Therefore, in the present study, we examined where CNTF was induced first in the kidney after a UUO injury, because in the early phase of a UUO injury the IM is more affected than any other renal structure [13, 14].
The patterns of CNTF induction during the early phase (up to day 7) of UUO injury were very similar to those of ischemia-reperfusion renal injury [10]. However, after day 7, CNTF expression between the UUO and acute ischemia-reperfusion model was different. Contrary to the acute renal injury model in which CNTF expression was restored to control levels by day 28, UUO animals retained strong CNTF immunoreactivity in the cPT cells of the cortex and OM until day 28. Such long-lasting CNTF expression in the UUO model may be attributed to chronic obstructive injury. In neuronal tissue, CNTF expression increases only in neuroglia, but not in neurons, following a variety of experimental injuries [15, 16]. Taken together with the data from the ischemia-reperfusion injury model, our data suggest that the S3s may be the principal induction site for CNTF in response to acute and chronic renal injury, although some tubular cells, the cPT, DT, and the IMCD also showed CNTF immunoreactivity.
Interestingly, the DTL expressed CNTF even in the normal state, and CNTF expression in the DTL was not affected by either ischemic-reperfusion or UUO injury. CNTF plays an important role in developing tissues; however, its function under normal physiological conditions in adult animals is still obscure. According to the classical vesicular mechanism, CNTF is not secreted without injury, because no signal sequence allows its secretion [17]. Moreover, several studies have failed to demonstrate the release of significant amounts of CNTF from cultured CNTF-expressing cells [18, 19]. CNTF may be released through other secretory pathways in damaged cells that do not require conventional signaling sequences. For example, cultured astrocytes can be stimulated by exogenous cytokines, such as interleukin-1 and tumor necrosis factor-α, to secrete CNTF [20]. Further investigations are required to examine the role of CNTF in the DTL.
We prepared a riboprobe for in situ hybridization of CNTFRα and confirmed its specificity by Northern blot. However, we observed only a slight difference to our previous in situ hybridization result [10] with a riboprobe provided by Dr. Hofmann [21]. Our present results showed increased sensitivity particularly in the TAL, and a moderate in situ signal was observed even in the medullary TAL from sham-operated animals. This difference may be attributed to the fenestration ability of the probe length between the present study (425 bp) and the previous study (>1.8 kb).
CNTFRα expression in the UUO model showed both similarities and differences compared to the ischemia-reperfusion model. CNTFRα expression in both models increased in the S3s of the PT, in the OSOM, and in the medullary TAL and progressively expanded to the cortex during the early phase (up to day 7). At the end of the early phase, almost all of the DT and TAL cells, and some of the cPT and PEC cells, showed strong CNTFRα expression. The difference between the two models was observed in the in situ signal from the collecting ducts. In ischemia-reperfusion animals, the cortical and medullary collecting ducts showed almost no CNTFRα in situ signal, whereas CNTFRα expression began to appear first in the IMCDs from as early as day 1 and increased progressively up to day 7 from the medulla to the cortex in the present study. Thereafter, increased CNTFRα expression in the collecting duct was maintained until day 28. The increased CNTFRα expression in the collecting duct in the UUO model may have been due to the fact that the medullary collecting ducts become markedly damaged in this model [13]. This could be evidence that CNTFRα is expressed in damaged renal tubular cells and plays an important role by combining with endogenous CNTF.
Many factors, including epidermal growth factor (EGF) and insulin-like growth factor (IGF-1) reduce the renal damage caused by UUO. However, EGF and IGF-1 expression decrease rapidly after UUO [22, 23]. Unlike EGF and IGF-1, CNTF and CNTFRα expression levels remained high until day 28, suggesting that CNTF may play an important role in UUO. Although many reports are available about factors related to UUO kidneys (for review article see Truong et al. [24]), very few studies are available on IL-6-family expression related to UUO kidneys, and there are no reports about CNTF in UUO. Elbjeirami et al. [25] reported that UUO increased OSM and expression of its specific receptor in a time-dependent manner within hours following UUO; therefore, OSM may play an important role initiating renal fibrogenesis. Akin et al. [26] reported increased renal IL-6 expression on day 30 after UUO using an enzyme-linked immunosorbent assay. Their results agree with the present results on the increase in CNTF and its receptor at both the early and late (day 28) stages of UUO. Further study will be needed to examine the roles of the IL-6 family, including CNTF.
Figures and Tables
Fig. 1
Light micrographs of a 50-µm thick vibratome section illustrating ciliary neurotrophic factor (CNTF) immunostaining in kidney of sham-operated rat (A), and in kidneys of rats who underwent unilateral ureteral obstruction (UUO) on days 1 (B), 3 (C), 5 (D), 7 (E), 14 (F), 21 (G), and 28 (H). (A) In sham-operated rats, CNTF-immunostaining was strong in the inner stripe (IS) of the outer medulla and in the inner medulla (IM). (B) From day 1 after UUO, induced CNTF immunostaining started to appear between the outer stripe (OS) of the outer medulla and the cortex (CO). CNTF immunostaining expanded into the cortex and the outer stripe of the outer medulla by day 7 (C-E), and then decreased slightly (F-H), but CNTF immunostaining in the OS and IS remained strong up to day 28 after UUO. Scale bar=1,000 µm.
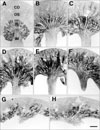
Fig. 2
High magnification of sham-operated (A, B) kidneys and those of rats who underwent unilateral ureteral obstruction on days 1 (C), 5 (D), 14 (E), and 28 (F) illustrating ciliary neurotrophic factor (CNTF) immunostaining. (A) No positive reaction was detected in the cortex. (B) Only the descending thin limb (arrows) showed CNTF immunoreactivity in the inner medulla . The collecting ducts (arrowheads) were negative. CNTF immunoreactivity was induced in several cells (arrows in C) of the S3 segment. Dotted lines indicate the boundary between the outer stripe (OS) and inner stripe (IS). Many CNTF-positive distal tubules (arrowheads) and proximal tubular cells (arrows) could be seen on day 5 (D), but only small numbers of tubular profiles (arrows) were immunoreactive on day 28 (F) in the cortex. (E) Note the numerous S3 segments with intense immunoreactivity (arrows). G, glomerulus; P, proximal tubule. Scale bar=100 µm.
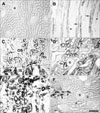
Fig. 3
One-µm thick sections from sham-operated (A) kidney and those of rats who underwent unilateral ureteral obstruction on days 7 (B), 21 (C, F), 3 (D), and 14 (E) illustrating ciliary neurotrophic factor (CNTF) immunostaining. (A) The descending thin limb (arrows) showed CNTF immunoreactivity in the outer medulla, whereas the thick ascending limb (asterisks) and collecting duct (CD) were negative. Note the CNTF-positive cells with no brush border (arrows in B) and CNTF-negative cells with a well-developed brush border (arrowheads in B) in the S3 segments. Cortical immunoreactivity on day 21 (C) was usually detected in the distal tubule (DT) and parietal epithelium (arrowhead) of Bowman's capsule. Proximal tubules (Ps in C) were immunonegative and appeared morphologically normal. The inner medullary collecting duct (CDs in D-F) showed no immunoreactivity up to day 7 (D), but weak immunostaining was detected from day 14 (E, F). Note strong immunoreactivity in the descending thin limb (arrows in E and F) and no immunoreactivity in the thick ascending limb (arrowheads in F). G, glomerulus. Scale bar=50 µm.
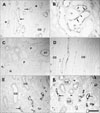
Fig. 4
Immunoblotting for ciliary neurotrophic factor (CNTF) protein in kidneys of rats after a sham operation or unilateral ureteral obstruction. A kidney from each rat was dissected into cortex, outer medulla, inner medulla, and protein (20 µg) was applied to each line. A 22-kDa band corresponded to the molecular weight of the CNTF protein. β-actin was re-probed to demonstrate equal protein loading and the 46-kDa band corresponded to the molecular weight. (A) Immunoblot for the CNTF protein in the cortex. (B) Immunoblot for the CNTF protein in the outer medulla. (C) Immunoblot for the CNTF protein in the inner medulla. *P<0.05 vs. sham. Optical densities are mean±standard deviation.
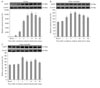
Fig. 5
Five µm thick waxed section illustrating ciliary neurotrophic factor-specific receptor alpha (CNTFRα) mRNA expression in kidney from sham-operated rat (A), and kidneys of rats who underwent unilateral ureteral obstruction on days 1 (B), 3 (C), 5 (D), 7 (E), 14 (F), 21 (G), and 28 (H). (A) A weak hybridization signal was observed in the cortex and outer medulla of sham-operated rats. In situ CNTFRα mRNA signals were induced in the outer medulla on day 1 (B), and the signals increased and expanded into the cortex on day 7 (C-E). Thereafter, the in situ CNTFRα mRNA signals decreased slightly (F-H). Note the persistently high levels of CNTFRα mRNA on day 28 (H). CO, cortex; OS, outer stripe; IS, inner stripe; IM, inner medulla. Scale bar=1,000 µm.
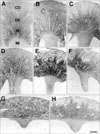
Fig. 6
High magnification of sham-operated (A-C) kidneys and those who underwent unilateral ureteral obstruction (D-F) illustrating ciliary neurotrophic factor-specific receptor alpha mRNA expression. A weak signal was shown only in some cells of the cortex (arrowheads in A) and of the distal tubule (DT). (B) Note the moderate to weak in situ signal in the thick ascending limb (asterisks), collecting duct (CD) in the outer stripe of outer medulla, and in the changing section from the descending thin limb to the S3 segment. The descending thin limb (arrow) was positive, whereas the S3 segment (arrowhead) was negative. (C) No detectable signal was observed in the inner medulla or the descending thin limb (arrowheads). On days 1 (D) and 3 (F), in the outer stripe of the outer medulla, moderately increased signals were observed in the thick ascending limb (asterisks), descending thin limb (arrows), and S3 segments (arrowheads). (E) The CDs of the inner medulla showed a moderate signal on day 1. G, glomerulus; P, proximal tubule. Scale bar=100 µm.
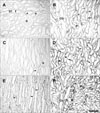
Fig. 7
High magnification of a rat kidney following unilateral ureteral obstruction illustrating ciliary neurotrophic factor-specific receptor alpha mRNA expression. (A) In the cortex, a strong signal was observed in the distal tubule (arrows), some proximal tubules (arrowheads), and in glomerular parietal epithelial cells (G) on day 5. In the outer stripe of the outer medulla on days 14 (C) and 28 (E), strong in situ signals were observed in the thick ascending limbs (asterisks) and S3 segments (arrowheads). (D) In the inner stripe of the outer medulla on day 21, signals were observed in the descending thin limb (arrowheads), thick ascending limb (asterisks), and collecting duct (CD). In the inner medulla, the CD showed a moderate signal on days 7 (B) and 28 (F). Scale bar=100 µm.
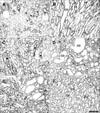
Acknowledgements
We would like to thank to Prof. Youg-Gyu Park (Dept. of Biostatistics, College of Medicine, The Catholic University of Korea) for helping us analyze the data.
References
1. Adler R, Landa KB, Manthorpe M, Varon S. Cholinergic neuronotrophic factors: intraocular distribution of trophic activity for ciliary neurons. Science. 1979. 204:1434–1436.
2. Ip NY, Nye SH, Boulton TG, Davis S, Taga T, Li Y, Birren SJ, Yasukawa K, Kishimoto T, Anderson DJ, Stahl N, Yancopoulos GD. CNTF and LIF act on neuronal cells via shared signaling pathways that involve the IL-6 signal transducing receptor component gp130. Cell. 1992. 69:1121–1132.
3. Ikeda K, Iwasaki Y, Shiojima T, Kinoshita M. Neuroprotective effect of various cytokines on developing spinal motoneurons following axotomy. J Neurol Sci. 1996. 135:109–113.
4. Humes HD, Lake EW, Liu S. Renal tubule cell repair following acute renal injury. Miner Electrolyte Metab. 1995. 21:353–365.
5. Heinrich PC, Behrmann I, Müller-Newen G, Schaper F, Graeve L. Interleukin-6-type cytokine signalling through the gp130/Jak/STAT pathway. Biochem J. 1998. 334(Pt 2):297–314.
6. Davis S, Aldrich TH, Stahl N, Pan L, Taga T, Kishimoto T, Ip NY, Yancopoulos GD. LIFR beta and gp130 as heterodimerizing signal transducers of the tripartite CNTF receptor. Science. 1993. 260:1805–1808.
7. Ohta K, Hara H, Hayashi K, Itoh N, Ohi T, Ohta M. Tissue expression of rat ciliary neurotrophic factor (CNTF) mRNA and production of the recombinant CNTF. Biochem Mol Biol Int. 1995. 35:283–290.
8. Ohta M, Ohi T, Nishimura M, Itoh N, Hayashi K, Ohta K. Distribution of and age-related changes in ciliary neurotrophic factor protein in rat tissues. Biochem Mol Biol Int. 1996. 40:671–678.
9. Ong AC, Fine LG. Tubular-derived growth factors and cytokines in the pathogenesis of tubulointerstitial fibrosis: implications for human renal disease progression. Am J Kidney Dis. 1994. 23:205–209.
10. Yang CW, Lim SW, Han KW, Ahn HJ, Park JH, Kim YH, Kirsh M, Cha JH, Park JH, Kim YS, Kim J, Bang BK. Upregulation of ciliary neurotrophic factor (CNTF) and CNTF receptor alpha in rat kidney with ischemia-reperfusion injury. J Am Soc Nephrol. 2001. 12:749–757.
11. Kim J, Kim YH, Cha JH, Tisher CC, Madsen KM. Intercalated cell subtypes in connecting tubule and cortical collecting duct of rat and mouse. J Am Soc Nephrol. 1999. 10:1–12.
12. Davis S, Aldrich TH, Valenzuela DM, Wong VV, Furth ME, Squinto SP, Yancopoulos GD. The receptor of ciliary neurotrophic factor. Science. 1991. 253:59–63.
13. Truong LD, Petrusevska G, Yang G, Gurpinar T, Shappell S, Lechago J, Rouse D, Suki WN. Cell apoptosis and proliferation in experimental chronic obstructive uropathy. Kidney Int. 1996. 50:200–207.
14. Nguyen HT, Wu HY, Baskin LS, Kogan BA. High urinary flow accelerates renal injury in young rats with partial unilateral ureteral obstruction. J Urol. 2000. 163:1904–1907.
15. Adler R. Ciliary neurotrophic factor as an injury factor. Curr Opin Neurobiol. 1993. 3:785–789.
16. Sleeman MW, Anderson KD, Lambert PD, Yancopoulos GD, Wiegand SJ. The ciliary neurotrophic factor and its receptor, CNTFR alpha. Pharm Acta Helv. 2000. 74:265–272.
17. Stöckli KA, Lottspeich F, Sendtner M, Masiakowski P, Carroll P, Götz R, Lindholm D, Thoenen H. Molecular cloning, expression and regional distribution of rat ciliary neurotrophic factor. Nature. 1989. 342:920–923.
18. Lillien LE, Sendtner M, Rohrer H, Hughes SM, Raff MC. Type-2 astrocyte development in rat brain cultures is initiated by a CNTF-like protein produced by type-1 astrocytes. Neuron. 1988. 1:485–494.
19. Lin LF, Mismer D, Lile JD, Armes LG, Butler ET 3rd, Vannice JL, Collins F. Purification, cloning, and expression of ciliary neurotrophic factor (CNTF). Science. 1989. 246:1023–1025.
20. Kamiguchi H, Yoshida K, Sagoh M, Sasaki H, Inaba M, Wakamoto H, Otani M, Toya S. Release of ciliary neurotrophic factor from cultured astrocytes and its modulation by cytokines. Neurochem Res. 1995. 20:1187–1193.
21. Lee MY, Naumann T, Kirsch M, Frotscher M, Hofmann HD. Transient up-regulation of ciliary neurotrophic factor receptor-alpha mRNA in axotomized rat septal neurons. Eur J Neurosci. 1997. 9:622–626.
22. Chevalier RL, Goyal S, Wolstenholme JT, Thornhill BA. Obstructive nephropathy in the neonatal rat is attenuated by epidermal growth factor. Kidney Int. 1998. 54:38–47.
23. Chevalier RL, Goyal S, Kim A, Chang AY, Landau D, LeRoith D. Renal tubulointerstitial injury from ureteral obstruction in the neonatal rat is attenuated by IGF-1. Kidney Int. 2000. 57:882–890.
24. Truong LD, Gaber L, Eknoyan G. Obstructive uropathy. Contrib Nephrol. 2011. 169:311–326.
25. Elbjeirami WM, Truong LD, Tawil A, Wang W, Dawson S, Lan HY, Zhang P, Garcia GE, Wayne Smith C. Early differential expression of oncostatin M in obstructive nephropathy. J Interferon Cytokine Res. 2010. 30:513–523.
26. Akin M, Demirbilek S, Ay S, Gurunluoglu K, Turkmen E, Tas E, Aksoy RT, Baykarabulut A, Edali MN. Attenuation of ureteral obstruction-induced renal injury by polyenyl phos phatidylcholine. Int J Urol. 2007. 14:350–356.