Abstract
This study evaluated the cellular localization of cyclic AMP-responsive element binding protein-binding protein (CBP) expression in pig retinas during postnatal development. Immunohistochemistry and Western blot analysis were performed on retinal tissue from 2-day-old, 5-week-old, and 6-month-old pigs. Western blot analysis detected the expression of CBP in the retinas of 2-day-old piglets and showed that it was significantly decreased in the retinas of 5-week-old and 6-month-old pigs. Immunohistochemically, CBP was intensely immunostained in protein kinase C alpha (PKCα)-positive-bipolar cells, glutamine synthetase-positive Müller cells, and in ganglion cells in 2-day-old piglets. CBP was detected weakly in the inner plexiform, outer nuclear, and rod and cone layers. CBP immunoreactivity in the ganglion cell layer was decreased in the retinas of 5-week-old and 6-month-old pigs, while clear CBP expression detected in the neurite of PKCα-positive bipolar cells in the inner nuclear layer. In addition, CBP immunoreactivity in Müller cells and glial fibrillary acidic protein-positive glial processes was particularly noteworthy in pig retinas, but not in rat retinas. The results indicate that CBP is expressed differentially in the retinal neurons and glial cells according to growth and animal species, and may play an important role in homeostasis in Müller cells, neurite extention in bipolar cells, and signal transduction in photoreceptor cells in the porcine retina.
Cyclic AMP responsive element binding protein (CREB) is a transcription factor [1] that is phosphorylated by protein kinase A (PKA) and that binds specifically to the nuclear protein CREB-binding protein (CBP) [2]. Signal cascades induce the expression or phosphorylation of CBP on extracellular stimulation. Subsequently, these cascades modulate cellular proliferation, differentiation, and apoptosis under normal and pathological conditions [3]. CBP activity has been examined in various tissues, and the highest levels have been detected in the brain, with relatively high levels detected in the lung, spleen, and heart, intermediate levels in the testes and skeletal muscles, and low levels in the liver and kidneys [4].
Furthermore, CBP is involved in a wide range of central nervous system (CNS) cellular and pathophysiological events, including hippocampal synaptic plasticity, hippocampus-dependent long-term memory formation [5], neurodegeneration [6], mammalian circadian clocks [7], neuronal survival [8], aging, and development [4]. The retina consists of neuronal and glial cell layers, and is classified as a remote CNS tissue. The role of CBP in Drosophila melanogaster retinal development has been studied [9]. However, there are few descriptions of CBP cellular expression patterns during mammalian retinal development. The pig retina shares many similarities with the human retina and can serve as an appropriate animal model [10]. We evaluated the cellular localization of CBP expression in the developing pig retina in this study.
Eyes from 2-day-old, 5-week-old, and 6-month-old female Landrace pigs (n=4-5 per group) were obtained from a local farm. The eyes were enucleated and the anterior segment of the globe was dissected. The retinas were detached carefully from the eyes in phosphate buffered saline (PBS; pH 7.4), fixed in 10% neutral-buffered formalin in 0.1 M PBS for histological analysis, and deep-frozen at -80℃ until used for Western blot analysis. Retinas recovered from female Sprague Dawley rats (7 to 8-week-old, 160-200 g) were used as a reference to compare the expression of CBP. All retinas had normal histological features. All experiments were performed in accordance with the Guide for the Care and Use of Laboratory Animals at Jeju National University.
Western blot analysis of the pig retinas was performed as reported previously [11], with minor modifications. Briefly, retinal tissues were thawed in lysis buffer consisting of 20 mM HEPES, pH 7.2 (Sigma-Aldrich, St. Louis, MO, USA), 1% Triton X-100 (AMRESCO, Solon, OH, USA), 1% deoxycholate (Sigma-Aldrich), 0.1% sodium dodecyl sulfate (SDS; Bio-Rad, Hercules, CA, USA), 150 mM NaCl (Junsei, Tokyo, Japan), 10 µg/ml leupeptin (Sigma-Aldrich), 10 µg/ml aprotinin (Sigma-Aldrich), and 1 mM phenylmethylsulfonyl fluoride (Sigma-Aldrich). Tissues were given 10 strokes in a Dounce homogenizer. After incubation for 60 minutes in an ice-bath, the homogenates were each centrifuged at 14,000 rpm for 20 minutes, and the supernatant was harvested. For the immunoblot assay, supernatant containing 40 µg of protein was loaded into individual lanes and subjected to 6% SDS-polyacrylamide gel electrophoresis. The resolved proteins were electroblotted onto nitrocellulose membranes (Schleicher and Schuell, Keene, NH, USA). The residual binding sites on the membranes were blocked by incubation with 5% nonfat milk in Tris-buffered saline (TBS; 10 mM Tris-HCl, pH 7.4, and 150 mM NaCl) for 1 hour at room temperature (RT). Subsequently, the membranes were incubated with rabbit anti-CBP (1 : 1,000, C-20 [sc-583], Santa Cruz Biotechnology, Santa Cruz, CA, USA) for 2 hours at RT. The membranes were washed three times in TBS containing 0.1% Tween-20 before being incubated with horseradish peroxidase-conjugated anti-rabbit IgG (1 : 2,000, Santa Cruz Biotechnology) for 1 hour at RT. The membranes were developed using an enhanced chemiluminescence reagent kit (Amersham Pharmacia Biotech, Uppsala, Sweden), employed according to the manufacturer's instructions, and exposed to medical X-ray film (Agfa Gevaert, Mortsel, Belgium). After imaging, the membranes were stripped and reprobed using monoclonal anti-β-tubulin antibody as the primary antibody (Sigma-Aldrich) using a similar protocol to that described above. The optical density (OD/mm2) of each band was measured with a GS-700 scanning laser densitometer (Bio-Rad) and the values are presented as the mean±standard error of the mean. The ratios of the density of the CBP band to that of the β-actin band were compared using Molecular Analyst software (Bio-Rad).
Immunohistochemistry of the pig retinas was performed as reported previously [12], with minor modifications. Five micrometer-thick paraffin wax embedded tissue sections were deparaffinized and rehydrated by routine protocols before being exposed to citrate buffer (0.01 M, pH 6.0) and heated in an autoclave for 10 minutes. They were then incubated in 0.3% hydrogen peroxide in methyl alcohol for 20 minutes to block the endogenous peroxidase activity. After three washes with PBS, the sections were incubated with 10% normal goat serum (Vector ABC Elite kit, Vector Laboratories, Burlingame, CA, USA) diluted in PBS, which was followed by incubation with rabbit polyclonal anti-CBP (1 : 400). After three washes in PBS, the sections were incubated with biotinylated anti-rabbit IgG (ABC Elite Kit, Vector Laboratories). After three washes in PBS the sections were incubated with avidin-biotin peroxidase complexes were formed using an Elite kit (Vector Laboratories). The peroxidase reaction was developed with a diaminobenzidine substrate kit (Vector Laboratories), and sections were counterstained with hematoxylin before mounting. As a negative control, the primary antiserum was omitted for a few test sections in each experiment; no specific labeling of cell bodies or fibers was detected in these sections (Fig. 2D). To examine the phenotype of CBP immunoreactive cells, double immunofluorescence was applied using cell-type-specific markers, including glial fibrillary acidic protein (GFAP), glutamine synthetase (GS), protein kinase C, alpha (PKCα), and parvalbumin for glial cells, Müller cells, bipolar cells, and amacrine cells, respectively. First, the sections prepared as described previously were incubated with rabbit anti-CBP overnight at 4℃, followed by biotinylated anti-rabbit IgG, and tetramethylrhodamine isothiocyanate (TRITC)-labeled streptavidin (Zymed, South San Francisco, CA, USA). The slides were then incubated with mouse monoclonal anti-GFAP (1 : 400, Sigma-Aldrich), anti-GS (1 : 5,000, Chemicon International, Temecula, CA, USA), anti-PKCα (Santa Cruz Biotechnology), or anti-parvalbumin (Sigma-Aldrich) overnight at 4℃, followed by fluorescein isothiocyanate (FITC)-labeled goat anti-mouse IgG (Sigma-Aldrich), for 1 hour at RT. Double immunofluorescencestained specimens were examined using a FV500 laser confocal microscope (Olympus, Tokyo, Japan).
CBP was strongly detected in the retinas of 2-day-old piglets (density value 6.8±1.63 OD/mm2) and was decreased significantly in the retinas of 5-week-old (2.23±0.44; P<0.05 vs. 2-day-old piglets) and 6-month-old pigs (2.34±0.57; P<0.05 vs. 2-day-old piglets) (Fig. 1).
The retinas of 2-day-old, 5-week-old, and 6-month-old pigs consisted of multiple layers, including the optic nerve fiber layer (ONF), ganglion cell layer (GCL), inner plexiform layer (IPL), inner nuclear cell layer (INL), outer plexiform layer (OPL), outer nuclear cell layer (ONL), and rod and cone layer (RCL). In the retinas of 2-day-old piglets, diffuse CBP immunoreactivity was detected in the IPL, OPL, and RCL. CBP was immunostained intensely in the glial process in the ONF, GCL, and IPL (Fig. 2A, E). Moderate CBP immunoreactivity was detected in the ganglion cells in the GCL (Fig. 2A, E). Additionally, intense CBP immunostaining was seen in some cells in the INL (Fig. 2A, H). Conversely, weak CBP immunostaining was identified in the ONL (Fig. 2A). In the retinas of 5-week-old (Fig. 2B, F, I) and 6-month-old pigs (Fig. 2C, G, J), CBP was immunostained in the ONF, GCL, IPL, INL, OPL, and ONL. CBP localization was similar in the retinas of 2-day-old piglets, but the CBP immunoreactivity in the ganglion cells was decreased (Fig. 2B, C). CBP immunoreactivity was also detected in the RCL. In the retinas of adult rats, CBP immunoreactivity was detected in ganglion cells in the GCL and some cells in the INL (Fig. 2K). Interestingly, there was no CBP immunostaining in the glial processes in the GCL or IPL, or in any neuronal or glial cells in the OPL, ONL, or RCL (Fig. 2K).
We performed double immunofluorescence staining with both GFAP (Fig. 3), and GS (Fig. 4) to ascertain the precise localization of CBP in the glial cells in the retina.
GFAP immunofluorescence was very rarely detected in the retinas of 2-day-old piglets, and it increased in the retinas of 5-week-old and 6-month-old pigs (Fig. 3). However, CBP immunoreactivity in the RCL was occasionally co-localized in a few GFAP-positive glial cells in the retinas of 5-week-old and 6-month-old pigs (Fig. 3). Most GS immunofluorescence was detected in the GCL and INL, but was limited in the IPL in the retinas of 2-day-old piglets (Fig. 4). Interestingly, most of the area in the GCL consisted of GS-positive Müller cells in the retinas of 2-day-old piglets (Fig. 4), indicating that the phenotype of glial cells in the piglets was GS-positive Müller cells, not GFAP-positive glial cells (Fig. 3). In the retinas of 5-week-old and 6-month-old pigs, GS immunoreactivity was seen in the GCL, IPL, and INL (Fig. 4). Most GS immunoreactivity in the GCL was co-localized with CBP in the GCL in all-age retinas, but occasionally co-localized with CBP in the IPL and INL (Fig. 4).
Next, we performed double immunofluorescence staining with both PKCα and parvalbumin to ascertain the precise localization of CBP in the bipolar cells, and amacrine cells in the INL, respectively. In the retina of all age pigs, CBP immunofluorescence in the INL co-localized with PKCα-positive bipolar cells (Fig. 5). Interestingly, some CBP immunoreactivity was detected in the processes of PKCα-positive bipolar cells in the retinas of 5-week-old pigs (Fig. 5), but immunoreactivity was not detected in the parvalbuminpositive amacrine cells in the retinas of all age pigs (data not shown).
To our knowledge, this is the first demonstration of CBP expression in retinal tissues from a domestic animal during development. CBP expression that decreased with aging was associated with retinal development after birth. In newborn piglets, GS-positive Müller cells in the GCL were more prominent, and had limited GFAP-positive radial fibers. Conversely, GFAP immunoreactivity was increased in the adult retina. CBP was abundant in GS-positive Müller cells, but was limited in GFAP-positive glial cells. The decrease in CBP expression with aging correlated with the population of GS-positive Müller cells and GFAP-positive glial cells in newborn and adult pigs.
Approximately 50% of ganglion cells in the mammalian retina die by apoptosis during development [13]. Neuron numbers in the GCL decrease with age in the human retina [14]. Furthermore, there are fewer ganglion cells in the adult pig retina than in the newborn retina [12], suggesting that CBP in the GCL may influence the postnatal reduction in ganglion cell numbers. Previous studies have demonstrated that CBP regulates apoptosis [15], and CBP activity decreases over time during neuronal apoptosis [6].
Additionally, CBP was presently detected in the bipolar cells in the INL, and its expression decreased. During early postnatal development, the growth or extension of axons and neurites in the INL of the retina is modulated by the deleted in colorectal cancer (DCC)/netrin-1 axonal guidance system [16]. DCC expression is detected in ganglion cell axons and IPL of the rat retina in the early postnatal period, and its expression declines with age [16]. Furthermore, increased cAMP or activated PKA in the neuronal cells increases DCC production and axon outgrowth [17, 18]. Also, we detected CBP expression in neurite of bipolar cells in the INL of 5-week-old pigs. Although the functional role of CBP in the bipolar cells was not proven here, it is possible that CBP plays a role in axonal growth and neurite extension in those cells in the early postnatal period, because CBP is an activator in the CREB pathway.
Regarding the cellular localization of CBP in retinal cells, we detected CBP in both the nuclei and cytoplasm of neurons and Müller cells in the pig retina, although the retinas examined in this study were not pathological. Furthermore, we detected CBP in the cytoplasm only in pigs, but not in rats. Thus, the functional role(s) of CBP in the retina may differ among species. We postulate that CBP translocates into the cytosol in Müller cells, particularly in pigs, but not in rats. These findings are supported by the previous detection of CBP in the cytoplasm during stress, as well as in the nucleus [3]. In addition, we detected more enhanced CBP expression in the vitread processes of Müller cells in ONF and GCL than in the cell bodies in INL. These observations indicate that the CBP in Müller cells may contribute to the support of the ganglion cells better than the neuronal cells in INL.
The CBP expression patterns in the inner segment of the RCL were of interest in this study. CBP immunoreactivity changes as a function of circadian time in the hamster suprachiasmatic nucleus [7]. Levels are significantly decreased at mid-night circadian times, compared with mid-day circadian times [7]. It was also reported that Xenopus retinal photoreceptor cells have a high-amplitude rhythm of CREB phosphorylation with a night-time peak [19]. Thus, CBP may be involved in the functional circadian clock, which is closely associated with photoreceptor cells. The functional significance of CBP in this layer remains to be determined.
In summary, we postulate that CBP is constitutively expressed in retinal cells, especially Müller cells and bipolar cells in porcine retina, and that CBP may have different functional roles in the retina according to growth and animal species.
Figures and Tables
Fig. 1
Western blot analysis of cyclic AMP-responsive element binding protein-binding protein (CBP) in the retinas of 2-day-old, 5-week-old, and 6-month-old pigs. (A) A representative photograph of a Western blot for CBP and β-tubulin. CBP and β-tubulin are detected at adove 200 kDa and at approximately 55 kDa, respectively, as confirmed by molecular weight markers. (B) Bar graph of densitometric data analysis (mean±standard error, n=4-5 pigs/group). The relative levels of CBP are calculated after normalization to β-tubulin bands. The value indicating CBP expression levels from the retina at 2-day-old is arbitrarily defined as 100 (B, bar graphs). *P<0.05 vs. 2-day-old piglets.
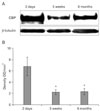
Fig. 2
Immunohistochemical analysis of cyclic AMP-responsive element binding protein-binding protein (CBP) in the retinas of 2-day-old (A, E, H), 5-week-old (B, F, L) and 6-month-old pigs (C, G, J). CBP immunoreactivity is detected in glial processes (arrowheads) and ganglion cells (arrow) in the ONF and GCL, some glial processes in the IPL, neuronal cells in INL, OPL, and RCL in the retinas of 2-day-old (A, E, H), 5-week-old (B, F, L) and 6-month-old pigs (C, G, J). (E and H, F and I, and G and J) are high-magnification images of GCL, and INL in (A, B, and C), respectively. No specific reaction product is seen in sections incubated with non-immune sera (D). In the rat retina, CBP immunostaining is evident in the ganglion cells (arrow) in the GCL, and some cells in the INL (K). Note the localization of CBP immunoreactivity mainly in the cytoplasm, the nucleus in the pig, and the retina in the rat. The retinal layers are indicated to the right: ONF, optic nerve fiber layer; GCL, ganglion cell layer; IPL, inner plexiform layer; INL, inner nuclear layer; OPL, outer plexiform layer; ONL, outer nuclear layer; RCL, layer of rods and cones. Counterstained with hematoxylin. Scale bars=25 µm (A-D, K), 12 µm (E-J).
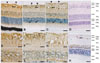
Fig. 3
Immunofluorescence staining of cyclic AMP-responsive element binding protein-binding protein (CBP, TRITC) with glial fibrillary acidic protein (GFAP, FITC) in the retina of 2-day-old, 5-week-old, and 6-month-old pigs. GFAP is detected very rarely in the retina of 2-day-old piglets, and it is abundant in the retina of 5-week-old and 6-month-old pigs. CBP immunoreactivity is occasionally co-localized in the GFAP-positive glial cells. ONF, optic nerve fiber layer; GCL, ganglion cell layer; IPL, inner plexiform layer; INL, inner nuclear layer; OPL, outer plexiform layer; ONL, outer nuclear layer; RCL, layer of rods and cones. Scale bars=50 µm.
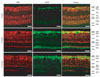
Fig. 4
Immunofluorescence staining of cyclic AMP-responsive element binding protein-binding protein (CBP, TRITC) with glutamine synthetase (GS, FITC) in the retina of 2-day-old, 5-week-old, and 6-month-old pigs. In ganglion cell layer (GCL), GS immunoreactivity is more abundant in retina of 2-day-old piglets compared to that of 5-week-old and 6-month-old pigs. CBP immunofluorescence is co-localized in GS-positive Müller cells. ONF, optic nerve fiber layer; IPL, inner plexiform layer; INL, inner nuclear layer; OPL, outer plexiform layer; ONL, outer nuclear layer; RCL, layer of rods and cones. Scale bars=50 µm.

Fig. 5
Immunofluorescence staining of cyclic AMP-responsive element binding protein binding protein (CBP, TRITC) with protein kinase C, alpha (PKCα, FITC) in the inner nuclear layer (INL) of retina of 2-day-old, 5-week-old, and 6-month-old pigs. CBP immunofluorescence is co-localized in PKCα-positive bipolar cells. INL, inner nuclear layer. Scale bars=20 µm.
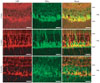
References
1. Brindle PK, Montminy MR. The CREB family of transcription activators. Curr Opin Genet Dev. 1992. 2:199–204.
2. Chrivia JC, Kwok RP, Lamb N, Hagiwara M, Montminy MR, Goodman RH. Phosphorylated CREB binds specifically to the nuclear protein CBP. Nature. 1993. 365:855–859.
3. Cohen HY, Lavu S, Bitterman KJ, Hekking B, Imahiyerobo TA, Miller C, Frye R, Ploegh H, Kessler BM, Sinclair DA. Acetylation of the C terminus of Ku70 by CBP and PCAF controls Bax-mediated apoptosis. Mol Cell. 2004. 13:627–638.
4. Li Q, Xiao H, Isobe K. Histone acetyltransferase activities of cAMP-regulated enhancer-binding protein and p300 in tissues of fetal, young, and old mice. J Gerontol A Biol Sci Med Sci. 2002. 57:B93–B98.
5. Wood MA, Kaplan MP, Park A, Blanchard EJ, Oliveira AM, Lombardi TL, Abel T. Transgenic mice expressing a truncated form of CREB-binding protein (CBP) exhibit deficits in hippocampal synaptic plasticity and memory storage. Learn Mem. 2005. 12:111–119.
6. Rouaux C, Jokic N, Mbebi C, Boutillier S, Loeffler JP, Boutillier AL. Critical loss of CBP/p300 histone acetylase activity by caspase-6 during neurodegeneration. EMBO J. 2003. 22:6537–6549.
7. Fiore P, Gannon RL. Expression of the transcriptional coactivators CBP and p300 in the hamster suprachiasmatic nucleus: possible molecular components of the mammalian circadian clock. Brain Res Mol Brain Res. 2003. 111:1–7.
8. Jin K, Mao XO, Simon RP, Greenberg DA. Cyclic AMP response element binding protein (CREB) and CREB binding protein (CBP) in global cerebral ischemia. J Mol Neurosci. 2001. 16:49–56.
9. Anderson J, Bhandari R, Kumar JP. A genetic screen identifies putative targets and binding partners of CREB-binding protein in the developing Drosophila eye. Genetics. 2005. 171:1655–1672.
10. Ruiz-Ederra J, García M, Hernández M, Urcola H, Hernández-Barbáchano E, Araiz J, Vecino E. The pig eye as a novel model of glaucoma. Exp Eye Res. 2005. 81:561–569.
11. Kim J, Moon C, Ahn M, Joo HG, Jin JK, Shin T. Immunohistochemical localization of galectin-3 in the pig retina during postnatal development. Mol Vis. 2009. 15:1971–1976.
12. Lee J, Kim H, Lee JM, Shin T. Immunohistochemical localization of heat shock protein 27 in the retina of pigs. Neurosci Lett. 2006. 406:227–231.
13. Kikuchi M, Tenneti L, Lipton SA. Role of p38 mitogen-activated protein kinase in axotomy-induced apoptosis of rat retinal ganglion cells. J Neurosci. 2000. 20:5037–5044.
14. Harman A, Abrahams B, Moore S, Hoskins R. Neuronal density in the human retinal ganglion cell layer from 16-77 years. Anat Rec. 2000. 260:124–131.
15. Dietze EC, Bowie ML, Mrózek K, Caldwell LE, Neal C, Marjoram RJ, Troch MM, Bean GR, Yokoyama KK, Ibarra CA, Seewaldt VL. CREB-binding protein regulates apoptosis and growth of HMECs grown in reconstituted ECM via laminin-5. J Cell Sci. 2005. 118(Pt 21):5005–5022.
16. Johansson K, Törngren M, Wasselius J, Månsson L, Ehinger B. Developmental expression of DCC in the rat retina. Brain Res Dev Brain Res. 2001. 130:133–138.
17. Bouchard JF, Moore SW, Tritsch NX, Roux PP, Shekarabi M, Barker PA, Kennedy TE. Protein kinase A activation promotes plasma membrane insertion of DCC from an intracellular pool: a novel mechanism regulating commissural axon extension. J Neurosci. 2004. 24:3040–3050.
18. Moore SW, Kennedy TE. Protein kinase A regulates the sensitivity of spinal commissural axon turning to netrin-1 but does not switch between chemoattraction and chemorepulsion. J Neurosci. 2006. 26:2419–2423.
19. Liu X, Green CB. Circadian regulation of nocturnin transcription by phosphorylated CREB in Xenopus retinal photoreceptor cells. Mol Cell Biol. 2002. 22:7501–7511.