Abstract
Ultrastructural parameters related to synaptic release and their correlation with synaptic connectivity were analyzed in the low-threshold mechanoreceptive vibrissa afferent boutons in laminae III and IV of the trigeminal caudal nucleus (Vc). Rapidly adapting vibrissa afferents were intra-axonally labeled, and quantitative ultrastructural analyses with serial sections were performed on the labeled boutons and their presynaptic endings (p-endings). The volume of the labeled boutons was widely distributed from small to large ones (0.8~12.3 µm3), whereas the p-endings were small and uniform in size. The volume of the labeled boutons was positively correlated with the ultrastructural parameters such as mitochondrial volume (correlation coefficient, r=0.96), active zone area (r=0.82) and apposed surface area (r=0.79). Vesicle density (r=-0.18) showed little correlation to the volume of labeled boutons, suggesting that the total vesicle number of a bouton is proportional to its volume. In addition, the bouton volume was positively correlated with the number of p-endings (r=0.52) and with the number of dendrites postsynaptic to the labeled bouton (r=0.83). These findings suggest that low-threshold mechanoreception conveyed through vibrissa afferents is processed in a bouton size-dependent manner in the Vc, which may contribute to the sensory-motor function of laminae III/IV in Vc.
Trigeminal sensory nuclei (TSN) receive somatosensory information from craniofacial region and project to higher brain center like as ventroposteromedial nucleus of the thalamus and to other related nuclei including adjacent reticular formation and trigeminal motor nucleus (Shigenaga et al., 1973, 1974, 1976, 1983, 1988; Mizuno et al., 1983; Hu & Sessle, 1984). It is subdivided into trigeminal principal (Vp), oral (Vo), interpolar (Vi) and caudal (Vc) nuclei by cytoarchitectonical and functional differences (Olszewski 1950). In the Vc, craniofacial nociceptive information projects to the laminae I and II, and low threshold mechanoreceptive information projects to the laminae III and IV (for review, see Sessle 2000).
Vibrissa is involved in various sensory functions including the detection of object localization, distance, orientation, texture, and air movements. Recently, we showed, in electron microscopy combined with electrophysiological characterization of vibrissa afferent fibers, that boutons arising from rapidly adapting, low threshold mechanoreceptive vibrissa afferents, show distinctive synaptic connectivity pattern in the Vi and Vc (Bae et al., 2005). It suggests that mechanoreceptive information conveyed through single vibrissa afferent is processed differently in the Vi and Vc. In addition, we also showed that, in the Vi, the vibrissa afferent receives extensive presynaptic modulation through axoaxonic synapse and large majority of p-endings presynaptic to the vibrissa afferent boutons contain both γ-aminobutyric acid (GABA) and glycine. The vibrissa afferent boutons were also quantitatively analyzed in the Vi and Vp (Nakagawa et al., 1997; Moon et al., 2008). Thus, bouton volume of the vibrissa afferents is much larger than the p-endings, and the bouton size is more widely distributed in the Vi than in the Vp. However, at present, little information is available on the size of vibrissa afferent boutons in the Vc.
Synaptic strength is determined by many factors such as release probability, extent of presynaptic firing, fiber excitability, degree of calcium influx, efficiency of reuptake system and postsynaptic receptor density (Atwood & Marin, 1983; Propst & Ko, 1987; Yeow & Peterson, 1991; Pierce & Lewin, 1994). It is also highly correlated with the amount of neurotransmitter released. Pierce & Mendell (1993) proposed ultrastructural "size principle" in Ia-motoneuron synapse in the spinal cord that bouton volume is positively correlated with sizes of the ultrastructural parameters related to synaptic release such as mitochondrial volume, active zone area and apposed surface area. It is uncertain whether the size principle is also applicable to the somatosensory afferent boutons in the Vc.
To address this issue, we performed quantitative ultrastructural analysis on the boutons arising from rapidly adapting vibrissa afferents in Vc by electron microscopy based on the serial thin sections.
Experiments were conducted on two adult cats weighing 2.5~3.0 kg. All animal procedures were in accordance with the Kyungpook National University and the National Institutes of Health guidelines, and approved by the Kyungpook National University Intramural Animal Care and Use Committee. Anesthesia was initially induced by ketamine (35 mg/kg, i.m.) followed by sodium pentobarbital (40 mg/kg, i.v.). Supplementary dose of sodium pentobarbital (10 mg/ml) was given throughout the experiment as necessary to maintain a deep level of anesthesia. The depth of anesthesia was monitored frequently by checking pupil size, pulse rate and the absence of changes in response to noxious stimuli throughout the experiment. Additional sodium pentobarbital was administered immediately prior to perfusion. End-tidal %CO2, rectal temperature, and electrocardiogram were monitored continuously during the experiments and maintained within physiological limits. After the animal was fixed to a stereotaxic apparatus, it was immobilized with pancuronium bromide (0.07 mg/kg, i.v.) and artificially ventilated.
Intra-axonal recordings and horseradish peroxidase (HRP) injections were made through glass microelectrodes with beveled tips (0.7~1.2 µm) filled by capillary action with a solution of 5~7% HRP (Toyobo, Japan) in 0.3 M KCl and 0.05 M Tris buffer at pH 7.6. Penetrations were made into the spinal trigeminal tract at the caudal level of the Vo, and intra-axonal placements were identified by a sudden negative DC potential shift and by the appearance of a large amplitude action potential evoked by stimulation of the infraorbital nerve (0.2 msec duration at 1 Hz). After an axon was penetrated, it was characterized physiologically as to its receptive field and response properties (to deflection of vibrissa or non-vibrissa hairs). Once rapidly adapting vibrissa afferent was identified, HRP was iontophoresed with 10~15 nA depolarizing continuous current for 5~10 minutes. In the two animals, two and five fibers, respectively, were filled with the HRP.
Fifteen or sixteen hours after the injection of HRP, the cats were perfused through the ascending aorta with 2.5% glutaraldehyde, 1.0% paraformaldehyde, 0.1% picric acid and 0.2 mM CaCl2 in 0.1 M phosphate buffer (PB; pH 7.4) for 40 minutes. The lower brain stem was removed and postfixed in the same fixative overnight at 4℃. Sixty µm-thick serial sections of brain stem were cut transversely on a Vibratome and processed with 3,3'-diaminobenzidine tetrahydrochloride (DAB). Sections containing HRP-labeled boutons in the Vc were selected by examining wet sections. The sections were postfixed with 1% osmium tetroxide (in 0.1 M PB, pH 6.0) for 40 minutes, dehydrated in a graded series of alcohol, flat embedded in Durcupan ACM (Fluka, Switzerland), and cured for 48 hours at 58℃. Areas containing many HRP-labeled boutons were cut out and glued onto blank plastic blocks with cyanoacrylate. Serial ultrathin sections were collected on formvar-coated single slot nickel grids, counterstained with uranyl acetate and lead citrate, and examined with an electron microscope (Hitachi H-7500, Japan).
For morphometric analysis of the ultrastructural parameters related to synaptic release, photomicrographs were taken from every other section through entire boutons in a series of grids. Labeled boutons reconstructed from serial sections that completely encompassed the entire bouton were analyzed. Bouton and mitochondrial volumes were calculated by measuring each cross sectional area and assuming an average section thickness of 75 nm. Active zone area and apposed surface area were calculated by measuring the length of the active zone and the portion of bouton surface apposed to its pre- and post-synaptic profiles. Morphometric measurements were performed on electron micrographs (×15,000, printed at ×23,000), using ImageJ software (version 1.38×; NIH, Bethesda, MD, USA). Interanimal variability in the ultrastructural data of the boutons was insignificant, and the data obtained from the animals could be pooled. Statistical significance was examined by unpaired Student's t-test (P<0.05), and correlation analysis with Fisher's r to z transformation for significance was used to test relationships.
HRP-labeled vibrissa afferents issued axon collaterals, which showed many en passant and terminal boutons in the laminae III/IV in Vc (Figs. 1A and B). At electron microscopic observation, the HRP-labeled boutons were identifiable by the electron-dense DAB reaction products deposited within the axoplasm (Figs. 1C~E). Labeled boutons varied from round or elongated to scalloped shape and contained many round vesicles. They made asymmetrical synapses with dendrites and were usually postsynaptic to p-endings containing pleomorphic vesicles, a mixture of round, oval, and flattened vesicles (Figs. 1C~E).
Of 28 HRP-labeled boutons examined, nine boutons (32%, 9/28) made synaptic contacts with dendrites only and the others (68%, 19/28) made synaptic contacts with both dendrites and p-endings (Table 1). Total 34 p-endings were presynaptic to the labeled boutons. Labeled boutons made synaptic contacts with 1 to 9 dendrites (2.3 dendrites per a bouton).
The volume of labeled boutons varied (0.8~12.3 µm3) widely, whereas p-endings (0.1~1.1 µm3) showed small and uniform in size (Fig. 2). The average volume of labeled boutons (3.55±2.48 µm3) was about 7 times larger than that of p-endings (0.47±0.23 µm3). The volume of labeled boutons was positively correlated with the number of postsynaptic dendrites (correlation coefficient, r=0.83) and of p-endings (r=0.52) (Fig. 3). In parallel with the size difference between the labeled boutons and the p-endings, the ultrastructural parameters related to the synaptic release, i.e., apposed surface area, active zone area, and mitochondrial volume were also significantly larger in the labeled boutons than in the p-endings (P<0.05). However, there was no significant difference between the size of the ultrastructural parameters in the labeled boutons showing synaptic contacts only with dendrites and those making contacts with both dendrites and p-endings. There were positive correlations between the volume of labeled boutons and their ultrastructural parameters, mitochondrial volume (r=0.96), apposed surface area (r=0.79) and active zone area (r=0.82) (P<0.0001, Fig. 4). Vesicle density (r=-0.18) showed little correlation with the volume of labeled boutons.
The main finding of the present study is that there is a positive correlation between the bouton volume of the vibrissa afferent and the size of the ultrastructural parameters related to the synaptic release in the Vc. In addition, size of the vibrissa afferent bouton was positively correlated with the number of postsynaptic dendrites as well as p-endings.
In the present study, bouton volume of the vibrissa afferents was linearly related to the size of the ultrastructural parameters related to the synaptic release such as mitochondrial volume, active zone area and apposed surface area in the Vc. In addition, very low correlation between bouton volume and vesicle density indicates that total vesicle number is also linearly related to the bouton size. These characteristics suggest that bouton size is directly associated with its capacity for synaptic release and may underlie potential synaptic efficacy or strength of the boutons (Pierce & Lewin, 1994). This is consistent with the findings of the tooth pulp afferents in the TSN (Bae et al., 2004), vibrissa afferents in the Vp (Nakagawa et al., 1997) and Vi (Moon et al., 2008), and slowly adapting lingual afferents in the Vp and Vo (Zhang et al., 2001). These findings also suggest that the "size principle" proposed by the Pierce & Mendell (1993) in the Ia-motoneuron synapse is also applicable to the trigeminal sensory afferents in the 1st relay nuclei of the brain stem.
The volume of vibrissa afferent boutons was positively correlated with the number of their postsynaptic dendrites. It indicates that the large-sized boutons make synaptic contacts with a large number of postsynaptic dendrites in a bouton size-dependent manner. Since a presynaptic bouton is known to make synaptic contact only with a single postsynaptic profile of a neuron (Yoshida et al., 2001), it has been postulated that the number of dendrites postsynaptic to a presynaptic bouton corresponds to the number of postsynaptic neurons. Thus, these findings provide a notion that amount of neurotransmitter assigned to single postsynaptic neuron may be similar to each other, although there are differences in the amount of synaptic release from each bouton.
Primary afferent boutons terminating into the TSN often receive presynaptic modulation through axoaxonic synapses with p-endings that contain neurotransmitters, GABA and/or glycine (Lovick 1981, 1983; Bae et al., 2000, 2005; Moon et al., 2008). Unlike the wide size variation of vibrissa afferent boutons, the p-endings are small, uniform in volume in the Vc and are about 7 times smaller in average volume than the vibrissa afferent boutons. Similarly, ultrastructural parameters related to synaptic release of p-endings such as mitochondrial volume, apposed surface area and active zone area were also smaller than those of vibrissa afferent boutons. It suggests that synaptic strength of the p-endings is fairly uniform each other in the Vc. In addition, bouton volume of vibrissa afferents was positively correlated with the number of p-endings, indicating that large boutons receive presynaptic inputs from a large number of p-endings. These findings signify that the strength of presynaptic modulation for the activity of vibrissa afferent boutons depends on the number of p-endings in the Vc. It is also observed in the vibrissa afferent boutons in Vi and Vp (Nakagawa et al., 1997; Moon et al., 2008), pulpal afferents in TSN (Bae et al., 2004) and Ia spindle afferents in the spinal ventral horn (Pierce & Mendell, 1993). It provides a notion that presynaptic modulation depending on the number of p-endings may be a general rule controlling the synaptic release of the primary afferent boutons.
Vibrissa afferent boutons in the Vc of the present study are smaller in average size (3.6 µm3 vs 8.6 µm3 in Vc vs Vi, respectively) and narrower in size distribution (0.8~12.3 µm3 vs 0.9~29.6 µm3 in Vc vs Vi, respectively) than those in Vi (Moon et al., 2008). In addition, boutons showing complex synaptic arrangement are far less frequently observed in the Vc than in the Vi (Bae et al., 2005). These findings also suggest that bouton size is closely related to the complexity of its synaptic arrangement. Taken together, differences in average size of bouton and distribution pattern of bouton size between Vi and Vc may be closely associated with the function of the two nuclei: sensory-motor function of lamina III/IV of Vc and sensory discriminative function of Vi (Sessle 1987).
Figures and Tables
Fig. 1
Light (A, B) and electron (C~E) micrographs showing HRP-labeled vibrissa afferent boutons in the laminae III/IV of the cat trigeminal caudal nucleus (Vc). (A, B) Light micrographs showing the HRP-labeled boutons (arrowheads) in laminae III/IV of Vc. B is an enlargement of boxed area in A. (C) An electron micrograph on a labeled bouton (asterisk) showing a simple synaptic connection with a dendrite (d). (D, E) Electron micrographs on adjacent thin sections showing a labeled bouton (asterisk). The bouton shows an example of complex synaptic arrangement with four dendrites (d1~d4) and presynaptic endings (p1) on adjacent thin sections. Arrows indicate the direction of synaptic transmission. Scale bars, 200 µm in (A); 20 µm in (B) 500 nm in (C~E).
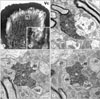
Fig. 2
Distribution of the volume of vibrissa afferent boutons and their presynaptic endings (p-endings) in the cat trigeminal caudal nucleus (Vc).
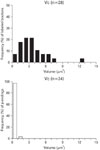
Fig. 3
Correlations between the volume of vibrissa afferent boutons and the number of presynaptic endings (p-endings), and between the volume of vibrissa afferent boutons and number of postsynaptic dendrites in the cat trigeminal caudal nucleus (Vc). r, correlation coefficient.
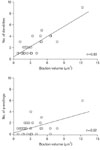
Fig. 4
Correlations between the volume of vibrissa afferent boutons and their mitochondrial volume, active zone area, apposed surface area and vesicle density in the cat trigeminal caudal nucleus (Vc). r, correlation coefficient.
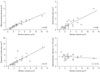
Table 1
Ultrastructural analysis of HRP-labeled vibrissa afferent boutons and their presynaptic endings (p-endings) in the cat trigeminal caudal nucleus
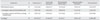
N indicates the number of HRP-labeled boutons and p-endings analyzed. *Vesicle density indicates number of vesicles per unit volume (µm3). †Values for the active zone area and apposed surface area indicate the data obtained from the contacts between HRP-labeled boutons and their p-endings. ‡Indicates statistically significant differences between HRP-labeled boutons and p-endings (unpaired t-test, P<0.05).
Acknowledgements
This work was supported by the Basic Science Research Program through the National Research Foundation of Korea (NRF) funded by the Ministry of Education, Science and Technology (314-2008-1-E00213).
References
1. Atwood HL, Marin L. Ultrastructure of synapses with different transmitter-releasing characteristics on motor axon terminals of a crab, Hyas areneas. Cell Tissue Res. 1983. 231:103–115.
2. Bae YC, Ahn HJ, Park KP, et al. The synaptic microcircuitry associated with primary afferent terminals in the interpolaris and caudalis of trigeminal sensory nuclear complex. Brain Res. 2005. 1060:118–125.
3. Bae YC, Ihn HJ, Park MJ, et al. Identification of signal substances in synapses made between primary afferents and their associated axon terminals in the rat trigeminal sensory nuclei. J Comp Neurol. 2000. 418:299–309.
4. Bae YC, Paik SK, Park KP, et al. Quantitative analysis of tooth pulp afferent terminals in the rat brain stem. Neuroreport. 2004. 15:2485–2489.
5. Hu JW, Sessle BJ. Comparison of responses of cutaneous nociceptive and nonnociceptive brain stem neurons in trigeminal subnucleus caudalis (medullary dorsal horn) and subnucleus oralis to natural and electrical stimulation of tooth pulp. J Neurophysiol. 1984. 52:39–53.
6. Lovick TA. Primary afferent depolarization of tooth pulp afferents by stimulation in nucleus raphe magnus and the adjacent reticular formation in the cat: effects of bicuculline. Neurosci Lett. 1981. 25:173–178.
7. Lovick TA. The role of 5-HT, GABA and opioid peptides in presynaptic inhibition of tooth pulp input from the medial brainstem. Brain Res. 1983. 289:135–142.
8. Mizuno N, Yasui Y, Nomura S, et al. A light and electron microscopic study of premotor neurons for the trigeminal motor nucleus. J Comp Neurol. 1983. 215:290–298.
9. Moon YS, Paik SK, Seo JH, et al. GABA- and glycine-like immunoreactivity in axonal endings presynaptic to the vibrissa afferents in the cat trigeminal interpolar nucleus. Neuroscience. 2008. 152:138–145.
10. Nakagawa S, Kurata S, Yoshida A, et al. Ultrastructural observations of synaptic connections of vibrissa afferent terminals in cat principal sensory nucleus and morphometry of related synaptic elements. J Comp Neurol. 1997. 389:12–33.
11. Olszewski J. On the anatomical and functional organization of the spinal trigeminal nucleus. J Comp Neurol. 1950. 92:401–413.
12. Pierce JP, Lewin GR. An ultrastructural size principle. Neuroscience. 1994. 58:441–446.
13. Pierce JP, Mendell LM. Quantitative ultrastructure of Ia boutons in the ventral horn: scaling and positional relationships. J Neurosci. 1993. 13:4748–4763.
14. Propst JW, Ko CP. Correlations between active zone ultrastructure and synaptic function studied with freeze-fracture of physiologically identified neuromuscular junctions. J Neurosci. 1987. 7:3654–3664.
15. Sessle BJ. The neurobiology of facial and dental pain: present knowledge, future directions. J Dent Res. 1987. 66:962–981.
16. Sessle BJ. Acute and chronic craniofacial pain: brainstem mechanisms of nociceptive transmission and neuroplasticity, and their clinical correlates. Crit Rev Oral Biol Med. 2000. 11:57–91.
17. Shigenaga Y, Matano S, Kusuyama M, Sakai A. Cortical neurons responding to electrical stimulations of the rat's incisor pulp. Brain Res. 1974. 67:153–156.
18. Shigenaga Y, Matano S, Okada K, Sakai A. The effects of tooth pulp stimulation in the thalamus and hypothalamus of the rat. Brain Res. 1973. 63:402–407.
19. Shigenaga Y, Nakatani Z, Nishimori T, Suemune S, Kuroda R, Matano S. The cells of origin of cat trigeminothalamic projections: especially in the caudal medulla. Brain Res. 1983. 277:201–222.
20. Shigenaga Y, Sakai A, Okada K. Effects of tooth pulp stimulation in trigeminal nucleus caudalis and adjacent reticular formation in rat. Brain Res. 1976. 103:400–406.
21. Shigenaga Y, Yoshida A, Mitsuhiro Y, Tsuru K, Doe K. Morphological and functional properties of trigeminal nucleus oralis neurons projecting to the trigeminal motor nucleus of the cat. Brain Res. 1988. 461:143–149.
22. Yeow MB, Peterson EH. Active zone organization and vesicle content scale with bouton size at a vertebrate central synapse. J Comp Neurol. 1991. 307:475–486.
23. Yoshida A, Fukami H, Nagase Y, et al. Quantitative analysis of synaptic contacts made between functionally identified oralis neurons and trigeminal motoneurons in cats. J Neurosci. 2001. 21:6298–6307.
24. Zhang LF, Moritani M, Honma S, Yoshida A, Shigenaga Y. Quantitative ultrastructure of slowly adapting lingual afferent terminals in the principal and oral nuclei in the cat. Synapse. 2001. 41:96–111.