Abstract
Among the several rotenoids, amorphigenin is isolated from the leaves of Amopha Fruticosa and it is known that has anti-proliferative effects and anti-cnacer effects in many cell types. The main aim of this study was to investigate the effects of amorphigenin on osteoclast differentiation in vitro and on LPS treated inflammatory bone loss model in vivo. We show here that amorphigenin inhibited RANKL-induced osteoclast differentiation from bone marrow macrophages in a dose dependent manner without cellular toxicity. Anti-osteoclastogenic properties of amorphigenin were based on a down-regulation of c-fos and NFATc1. Amorphigenin markedly inhibited RANKL-induced p38 and NF-κB pathways, but other pathways were not affected. Micro-CT analysis of the femurs showed that amorphigenin protected the LPS-induced bone loss. We concluded that amorphigenin can prevent inflammation-induced bone loss. Thus we expect that amorphigenin could be a treatment option for bone erosion caused by inflammation.
Osteoclasts are bone resorbing multinuclear cells originating from the hematopoietic stem cells. They attach to the exterior of the bone and erode the surface with numerous enzymes and acids (Teitelbaum 2000; Boyle et al., 2003). The main role of osteoclasts is bone remodeling, they constantly dissolve and get rid of old or damaged bones making way for the osteoblasts make new ones. Decrease in bone mass resulting from imbalance between bone resorption by osteoclasts and bone formation by osteoblasts can be caused by various hormones and cytokines, which often leads to bone diseases such as autoimmune arthritis, periodontitis, postmenopausal osteoporosis, paget's disease, and bone tumors (Rodan & Martin, 2000; Takayanagi et al., 2000).
The first line treatment that are currently used in treating osteoporosis primarily include bisphosphonates (e.g. alendronate, risedronate), along with estrogen replacement therapy, selective estrogen receptor modulators (SERM), and calcitonin for postmenopausal osteoporosis. However, such therapies are associated with adverse effects, including breast cancer, endometritis, thromboembolism, hypercalcemia, GI problems, and hypertension (Lloyd 1998; Rodan & Martin, 2000; O'Regan & Gradishar, 2001; Body 2002; Watts 2003).
Most of the drugs we use to treat osteoporosis have many side effects. Thus, many plant-derived natural products have been used in traditional medicine for the treatment of various diseases. Recently, several previous reports have shown that several compounds derived from natural products suppress osteoclast differentiation and function (Li et al., 2007; Hu et al., 2008). Also, we have previously reported that curcumin, a compound derived from Curcuma aromatica, Stewartia koreana extract, tanshinone IIA derived from Danshen (also called Tanshen, the deried roots of Salvia miltiorrhiza) exert an inhibitory effect on osteoclast differentiation and bone resorption in vitro and in vivo (Bharti et al., 2004; Kwak et al., 2006; Park et al., 2007). More recently, we reported that rotenone, which is derived from Derris elliptica, significantly inhibits osteoclast differentiation, bone resorption, and bone erosion (Kwak et al., 2010). Thus, we screened rotenone derivate and examined the effect of amorphigenin in osteoclast differentiation and bone resorption in vitro and in vivo. Amorphigenin (also known as 8'-hydroxyrotenone), an aglycone of the rotenoid glycoside amorphin from plants of the genus Amorpha, was initially identified as a flavonoids isolated from Amorpha fructiosa (Kloutek et al., 1985). Up to now little is known about the biological function of amorphigenin.
In this study, we found that amorphigenin suppressed osteoclast differentiation of osteoclast precursors, bone marrow-derived macrophages (BMMs). The effect of amorphigenin may be involved to the inhibition of c-Fos and NFATc1 expression in BMMs treated RANKL. Amorphigenin considerably inhibits bone erosion in a mouse model of bone loss.
Amorphogen, TRAP assay kit, LPS, and anti-actin antibody were purchased from Sigma Aldrich (St. Louis, MO, USA). Human soluble RANKL and M-CSF were from PeproTech EC (London, UK). For cytotoxicity test, XTT assay kit was purchased from Roche Diagnostics (Indianapolis, IN, USA). Antibodies against c-Fos and NFATc1 were from Santa Cruz Biotechnology (Santa Cruz, CA, USA). Antibodies against Phospho (p)-p38, p-JNK, p-ERK, p-Akt, Akt, I-κB was purchased from Cell signaling Technology (Beverly, MA). ICR mice were from Dae Han Biolink Co. Ltd. (Chungbuk, Korea).
Bone marrow cells (BMCs) were isolated from long bones of 5-week-old ICR mouse by flushing with α-minimum essential medium (α-MEM; Welgene, Daegu, Korea) containing antibiotics (Sigma Aldrich), and further red blood cells (RBC) were removed with RBC lysis buffer (Sigma Aldrich). The cells were placed on 90-mm culture dishes (Corning, NY, USA) and incubated for 1 day in α-MEM containing 10% fetal bovine serum (Gibco BRL, Grand Island, NY) and antibiotics (Gibco BRL) in the presence of M-CSF (10 ng/ml). Nonadherent cells were seeded on 90-mm petri dishes and then cultured for 3 days in the presence of M-CSF (30 ng/ml). Adherent cells were used as bone marrow-derived macrophages (BMMs), namely osteoclast precursors. To generate osteoclasts from osteoclast precursors, BMMs were further cultured for 4 days in the presence of M-CSF (30 ng/ml) and RANKL (50 ng/ml) with or without amorphigenin in various concentrations.
Total RNA was isolated from treated cells by using TRIzol reagent (Invitrogen, Carlsbad, CA, USA) according to the manufacturer's protocol. cDNA was synthesized from 1 µg of total RNA using oligo dT, dNTP, dithiothreitol, RNase inhibitor, 1x PCR buffer, and Superscript II reverse transcriptase (Invitrogen). 1 µl of cDNA was amplified by PCR using Taq polymerase (Invitrogen). The following primers were used: c-Fos sense, 5'-CTGGTGCAGCCCACTCTGGTC-3'; c-Fos antisense, 5'-CTTTCAGCAGATTGGCAATCTC-3'; NFATc1 sense, 5'-CAACGCCCTGACCACCGATAG-3'; N FATc1 antisense, 5'-GGCTGCCTTCCGTCTCATAGT-3'; TRAP sense, 5'-ACTTCCCAGCCCTTACTAC-3'; TRAP antisense, 5'-TCAGCACATAGCCCACACCG-3'; GAPD H sense, 5'-ACCACAGTCCATGCCATCAC-3'; OSCAR sense, 5'-CTGCTGGTAACGGATCAGCTCCCCAGA-3'; OSCAR antisense, 5'-CCAAGGAGCCAGAACCTTCGAAACT-3'; GAPDH antisense, 5'-TCCACCACCCTGTTGCTGTA-3'. PCR products were electrophoresed on 1% agarose gels and visualized under UV after ethidium bromide staining.
Cells were lysed in lysis buffer containing 50 mM Tris-Cl, 150 mM NaCl, 5 mM EDTA, 1% Triton X-100, 1 mM sodium fluoride, 1 mM sodium vanadate, 1% deoxycholate, and protease inhibitors. Proteins were boiled in SDS sample buffer, resolved by 10% SDS-polyacrylamide gel electrophoresis, and transferred to a PVDF membrane (Amersham Biosciences, Piscataway, NJ, USA). The membrane was probed with antibodies, as indicated, washed extensively, and then incubated with secondary antibody conjugated to horseradish peroxidase. Bound antibody was detected by using enhanced chemiluminescent reagents (ECL plus, GE Healthcare).
BMMs (1×104 cells/well) were seeded in triplicate in 96-well plates in α-MEM and then incubated for 3 days with M-CSF (30 ng/ml) in the presence or absence of amorphigenin in various concentrations. After 3 days incubation, 50 µl of XTT reagents were added to each well and incubated for 4 h. Absorbance was measured at 450 nm using an ELISA plate reader (Thermomax; Molecular Devices, Sunnyvale, CA).
6-week-old ICR mice were intraperitoneally administered amorphigenin (10 µg/g of body weight) or PBS 1 day before the injection of LPS. Amorphigenin was intraperitoneally administered every other day for 8 days. Mice were injected intraperitoneally with LPS (5 µg/g of body weight) on days 0 and 4. The femurs were obtained on the day 8 after the first injection of LPS and were fixed in 4% paraformaldehyde for 1 day. Radiographic images were taken with micro-CT scan (NFR-Polaris-S160: Nano Focus Ray, Iksan, Korea). Bone volume/tissue volume was calculated by using the VG Studio program.
All quantitative results are expressed as mean±S.D. from at least 3 independent experiments. Differences among groups were assessed using the one-factor analysis of variance (ANOVA) followed by Duncan's multiple range test. Values of p<0.05 were considered as statistically significant and expressed as asterisk.
Because rotenone significantly inhibits osteoclast differentiation and bone erosion in vitro and in vivo as reported previously (Kwak et al., 2010), we examined whether amorphigenin, a rotenone derivate, can inhibit osteoclast differentiaion. Osteoclasts were differentiated from mouse BMMs in the presence of M-CSF (30 ng/ml) plus RANKL (50 ng/ml). The BMMs of the control group differentiated into mature TRAP-positive multinucleated osteoclasts while amorphigenin reduced the formation and numbers of TRAP-positive multinucleated cells in a dose-dependent manner (Fig. 1).
Amorphigenin generated a highly negative effect on osteoclastogenesis. We measured the effects of amorphigenin on BMMs with the XTT assay to exclude the possibility that the inhibition was due to cytotoxicity. amorphigenin demonstrated no cytotoxic effects at the same doses which effectively inhibited osteoclast differentiation (Fig. 2). Our results suggest that osteoclast formation inhibited by amorphigenin was not due to toxic effects on BMMs.
RANKL activates a variety of signal transducers that are involved in osteoclastogenesis, including p38, JNK, ERK, MAPK, Akt, and transcription factor NF-κB (Boyle et al., 2003), which are recognized as the key factors of osteoclast differentiation. Osteoclast precursors were pretreated with amorphigenin and stimulated with RANKL at various time points. Different signaling pathways were observed. Phosphorylation of p38, Akt, and I-κB by RANKL were significantly inhibited by amorphigenin, but amorphigenin did not affect the phosphorylation of JNK and ERK (Fig. 3). This raises the possibility that the negative effect of amorphigenin is dependent on its inhibitory effect of certain signal transduction molecules such as p38 and NF-κB.
We know from numerous previous reports that c-Fos and NFATc1 play a vital role in osteoclast differentiation. We examined the potential effects of amorphigenin on the expression of c-Fos and NFATc1 in response to RANKL. As shown in Fig. 4, amorphigenin inhibited the expression of c-Fos, NFATc1 and OSCAR mRNA expression in response to RANKL. Therefore, c-Fos and NFATc1 may be targets of amorphigenin in the inhibition of osteoclast differentiation induced by RANKL.
To elucidate the inhibitory effect of amorphigenin in mRNA expression, we next examined whether amorphigenin inhibits the protein levels of c-Fos and NFATc1 induced by RANKL, as shown by RT-PCR results. BMMs were treated with RANKL in the presence or absence of amorphigenin. Protein levels of c-Fos and NFATc1 were increased in BMMs treated with RANKL, as shown by RT-PCR analysis. However, the expression of both c-Fos and NFATc1 protein was significantly inhibited by amorphigenin (Fig. 5). These results suggest that the inhibitory effect of amorphigenin may be involved in the inhibition of c-Fos and NFATc1 induced by RANKL.
Osteoclasts are responsible for bone destruction in inflammatory bone disease, and inhibition of osteoclast differentiation by amorphigenin treatment may exert anti-bone resorptive effect in vivo. Therefore to examine the effect of amorphigenin on bone erosion in vivo, an inflammatory bone disease model was created by intraperitoneal injection of LPS into mice. Oral administration of DW with or without amorphigenin was performed. On micro-CT scan, LPS injection induced severe trabecular bone loss in the femur. On the contrary, scans from mice in the oral amorphigenin-treated group showed significant reduction of bone loss (Fig. 6A). Furthermore, loss of bone volume (bone volume/tissue volume) induced by LPS was prevented in amorphigenin-administered mice (Fig. 6B).
Bone is restructured at such a high speed that approximately 10% of the total bone content is replaced every year in human adults (Rodan & Martin, 2000). This balance is tightly regulated by numerous factors, and the most important factor is RANKL. Any tip towards one side of this balance will lead to osteoporosis or osteopetrosis (Walsh et al., 2006). Most of the bone related metabolic disease has osteoclast as a main executor. Either a hyperactive or an inactive osteoclast is responsible for osteoporosis, inflammatory bone disease such as rheumatoid arthritis and periodontitis, paget disease and osteopetrosis (Bartold et al., 2005; Everts et al., 2009). Therefore, a compound to increase osteoblast activity and inhibit osteoclast activity would show potential as an ideal therapeutic for osteoporosis. Here we investigated the effect of amorphigenin in osteoclast differentiation that is derived from the leaves of Amopha Fruticosa. Amorphigenin considerably inhibited RANKL-induced osteoclast differentiation from BMMs in a dose-dependent manner without toxicity (Figs. 1 and 2).
The genes responsible for the differentiation of osteoclasts are expressed in different stages of development. In the early stage, PU.1 regulates differentiation of primitive hematopoietic cells (Hayashi et al., 1998). M-CSF is responsible for inducing RANK in the surface of the hematopoietic stem cells, along with Bcl-2 for the regulation of survival and apoptosis (Zhao et al., 2007). RANKL, RANK, TRAF6, NF-κB, NFATc1, c-Fos, and MITF are the major contributors for the differentiation of TRAP-positive mononuclear osteoclasts (Boyle et al., 2003; Teitelbaum & Ross, 2003; Takayanagi 2005). And the key transcription factor is NFATc1, for this is the master regulator of osteoclast differentiation (Takayanagi et al., 2002). NFATc1 transcribes DC-STAMP and Atp6v0d2 for cell fusion, cathepsin K, TRAP, OSCAR, MMP9 etc for function (Takayanagi 2007; Kim et al., 2008). We also found that amorphigenin significantly inhibited expression of c-Fos and NFATc1 induced by RANKL, and the mRNA expression levels of markers of osteoclast such as TRAP and OSCAR was also inhibited by amorphigenin (Figs. 4 and 5). These results suggest that the differentiating mechanism of osteoclast differentiation influenced by amorphigenin is related to decreased expression of c-Fos and NFATc1.
LPS, a potent inducer of inflammation in vivo, induced bone erosion, although the mechanism of this effect remains unknown (Miyaura et al., 2003). We previously have shown that LPS greatly induce bone erosion in vivo (Kwak et al., 2010). Thus, we examined the effect of amorphigenin in LPS-mediated bone erosion. Importantly, LPS-injected mice showed a profound decrease in the density of trabeculae in the femur. However, amorphigenin significantly protected bone erosion induced by LPS (Fig. 6). Our results indicated that amorphigenin inhibits osteoclastic bone resorption as well as differentiation into osteoclasts in vivo.
The mainline drug that is currently used in the treatment of osteoporosis is bisphosphonates. The main function of bisphophonates is the binding of the drug to the hydroxyapatite of the bone, this can distort the absorption capacity of the osteoclasts (Russell et al., 1999). Osteoclasts can also take this drug into their system, thus triggering an automated cell death system and inducing osteoclast apoptosis. Recent reports of bisphosphonates-related osteonecrosis of the jaw (Filleul et al., 2010) and other adverse effects can limit the use of bisphosphonates. The demand for new drugs other than the traditional treatment modalities has constantly been up for grabs. Therefore, we assert that amorphigenin could be used not only for the treatment of post-menopausal osteoporosis and senile osteoporosis but also in numerous other areas such as in inflammatory arthritis and infectious conditions of bone such as osteomyelitis and chronic periodontitis.
Figures and Tables
Fig. 1
Amorphigenin inhibits osteoclast differentiation in BMMs. (A) BMMs were cultured with M-CSF (30 ng/ml) and RANKL (50 ng/ml) in the presence of amorphigenin for 4 days. Then the cells were fixed in 3.7% formalin, permeabilized in 0.1% Triton X-100, and stained for TRAP. Arrows indicate the multinuclear osteoclasts. Arrowheads indicate the mononuclear osteoclasts (B) TRAP-positive cells were counted as osteoclasts. Askerisks indicate statistical difference from the control.
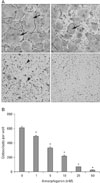
Fig. 2
Effect of amorphigenin on cytotoxicity. BMMs were seded onto 96-well plate. Then the cells were cultured for 3 days with the indicated concentration of amorphigenin in the presence of M-CSF (30 ng/ml). After 3 days, XTT reagents were added to each well. The optical density was read at 450 nm in an ELISA plate reader after 4 hours of incubation. Cell viability was determined relative to the control.
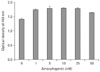
Fig. 3
Effects of amorphigenin in RANKL signaling. BMMs were pretreated with or without amorphigenin and stimulated with RANKL (100 ng/ml) for the indicated times. The cells were lysed in lysis buffer. Cell lysates were performed by Wesern blotting with the indicated antibodies.
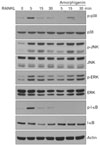
Fig. 4
Amorphigenin suppresses RANKL-induced gene expression. BMMs were pretreated with or without amorphigenin and then stimulated with RANKL (100 ng/ml) for the indicated times. Total RNA was isolated from the treated cells. The mRNA expression of c-fos, NFATc1, TRAP, OSCAR, and GAPDH was analyzed by RT-PCR.
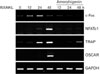
Fig. 5
Amorphigenin inhibits RANKL-mediated c-Fos and NFATc1 expression. BMMs were pretreated with or without amorphigenin and stimulated with RANKL (100 ng/ml) for the indicated times. The cells were lysed in lysis buffer. c-Fos, NFATc1, or actin proteins were detected by Western blotting with an anti-c-fos, and anti-NFATc1, or anti-actin-antibody, respectively.
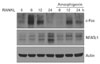
Fig. 6
Suppression of LPS-induced bone loss by amorphigenin. Mice were injected intraperitonealy with amorphigenin (10 µg/g of body weight) on days -1, 1, 3, 5, and 7 days. LPS was injected intraperitoneally on days 0 and 4. The mice were killed 8 days after the first injection of LPS, and femurs were collected. (A) The femurs were imaged with a micro-CT machine. (B) Bone volume/tissue volume (BV/TV) was analyzed using the histomorphometric results. Asterisks indicate statistical difference between the indicated groups.
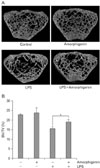
References
1. Bartold PM, Marshall RI, Haynes DR. Periodontitis and rheumatoid arthritis: a review. J Periodontol. 2005. 76:11 Suppl. 2066–2074.
2. Bharti AC, Takada Y, Aggarwal BB. Curcumin (diferuloylmethane) inhibits receptor activator of NF-kappa B ligand-induced NF-kappa B activation in osteoclast precursors and suppresses osteoclastogenesis. J Immunol. 2004. 172:5940–5947.
3. Body JJ. Calcitonin for the long-term prevention and treatment of postmenopausal osteoporosis. Bone. 2002. 30:5 Suppl. 75S–79S.
4. Boyle WJ, Simonet WS, Lacey DL. Osteoclast differentiation and activation. Nature. 2003. 423:337–342.
5. Everts V, de Vries TJ, Helfrich MH. Osteoclast heterogeneity: lessons from osteopetrosis and inflammatory conditions. Biochim Biophys Acta. 2009. 1792:757–765.
6. Filleul O, Crompot E, Saussez S. Bisphosphonate-induced osteonecrosis of the jaw: a review of 2,400 patient cases. J Cancer Res Clin Oncol. 2010. 136:1117–1124.
7. Hayashi S, Yamane T, Miyamoto A, et al. Commitment and differentiation of stem cells to the osteoclast lineage. Biochem Cell Biol. 1998. 76:911–922.
8. Hu JP, Nishishita K, Sakai E, et al. Berberine inhibits RANKL-induced osteoclast formation and survival through suppressing the NF-kappaB and Akt pathways. Eur J Pharmacol. 2008. 580:70–79.
9. Kim K, Lee SH, Ha Kim J, Choi Y, Kim N. NFATc1 induces osteoclast fusion via up-regulation of Atp6v0d2 and the dendritic cell-specific transmembrane protein (DC-STAMP). Mol Endocrinol. 2008. 22:176–185.
10. Kloutek E, Popov A, Drenska D, Uzunov P. Experimental research on the hepatoprotective activity of flavonoids isolated from Amorpha fructiosa. Eksp Med Morfol. 1985. 24:50–54.
11. Kwak HB, Lee BK, Oh J, et al. Inhibition of osteoclast differentiation and bone resorption by rotenone, through down-regulation of RANKL-induced c-Fos and NFATc1 expression. Bone. 2010. 46:724–731.
12. Kwak HB, Yang D, Ha H, et al. Tanshinone IIA inhibits osteoclast differentiation through down-regulation of c-Fos and NFATc1. Exp Mol Med. 2006. 38:256–264.
13. Li YJ, Kim TH, Kwak HB, Lee ZH, Lee SY, Jhon GJ. Chloroform extract of deer antler inhibits osteoclast differentiation and bone resorption. J Ethnopharmacol. 2007. 113:191–198.
14. Lloyd M. Treatment of postmenopausal osteoporosis. N Engl J Med. 1998. 339:202.
15. Miyaura C, Inada M, Matsumoto C, et al. An essential role of cytosolic phospholipase A2alpha in prostaglandin E2-mediated bone resorption associated with inflammation. J Exp Med. 2003. 197:1303–1310.
16. O'Regan RM, Gradishar WJ. Selective estrogen-receptor modulators in 2001. Oncology (Williston Park). 2001. 15:1177–1185.
17. Park CK, Kim HJ, Kwak HB, et al. Inhibitory effects of Stewartia koreana on osteoclast differentiation and bone resorption. Int Immunopharmacol. 2007. 7:1507–1516.
18. Rodan GA, Martin TJ. Therapeutic approaches to bone diseases. Science. 2000. 289:1508–1514.
19. Russell RG, Rogers MJ, Frith JC, et al. The pharmacology of bisphosphonates and new insights into their mechanisms of action. J Bone Miner Res. 1999. 14:Suppl 2. 53–65.
20. Takayanagi H. Mechanistic insight into osteoclast differentiation in osteoimmunology. J Mol Med. 2005. 83:170–179.
21. Takayanagi H. Osteoimmunology: shared mechanisms and crosstalk between the immune and bone systems. Nat Rev Immunol. 2007. 7:292–304.
22. Takayanagi H, Iizuka H, Juji T, et al. Involvement of receptor activator of nuclear factor kappaB ligand/osteoclast differentiation factor in osteoclastogenesis from synoviocytes in rheumatoid arthritis. Arthritis Rheum. 2000. 43:259–269.
23. Takayanagi H, Kim S, Koga T, et al. Induction and activation of the transcription factor NFATc1 (NFAT2) integrate RANKL signaling in terminal differentiation of osteoclasts. Dev Cell. 2002. 3:889–901.
24. Teitelbaum SL. Bone resorption by osteoclasts. Science. 2000. 289:1504–1508.
25. Teitelbaum SL, Ross FP. Genetic regulation of osteoclast development and function. Nat Rev Genet. 2003. 4:638–649.
26. Walsh MC, Kim N, Kadono Y, et al. Osteoimmunology: interplay between the immune system and bone metabolism. Annu Rev Immunol. 2006. 24:33–63.
27. Watts NB. Bisphosphonate treatment of osteoporosis. Clin Geriatr Med. 2003. 19:395–414.
28. Zhao Q, Shao J, Chen W, Li YP. Osteoclast differentiation and gene regulation. Front Biosci. 2007. 12:2519–2529.