Abstract
Nitric oxide (NO) production by endothelial nitric oxide synthase (eNOS) plays a protective role in cerebral ischemia by maintaining vascular permeability, whereas NO derived from neuronal and inducible NOS is neurotoxic and can participate in neuronal damage occurring in ischemia. Matrix metalloproteinases (MMPs) are up-regulated by ischemic injury and degrade the basement membrane if brain vessels to promote cell death and tissue injury. We previously reported that agmatine, synthesized from L-arginine by arginine decarboxylase (ADC) which is expressed in endothelial cells, has shown a direct increased eNOS expression and decreased MMPs expression in bEnd3 cells. But, there are few reports about the regulation of eNOS by agmatine in ischemic animal model. In the present study, we examined the expression of eNOS and MMPs by agmatine treatment after transient global ischemia in vivo. Global ischemia was induced with four vessel occlusion (4-VO) and agmatine (100 mg/kg) was administered intraperitoneally at the onset of reperfusion. The animals were euthanized at 6 and 24 hours after global ischemia and prepared for other analysis. Global ischemia led severe neuronal damage in the rat hippocampus and cerebral cortex, but agmatine treatment protected neurons from ischemic injury. Moreover, the level and expression of eNOS was increased by agmatine treatment, whereas inducible NOS (iNOS) and MMP-9 protein expressions were decreased in the brain. These results suggest that agmatine protects microvessels in the brain by activation eNOS as well as reduces extracellular matrix degradation during the early phase of ischemic insult.
Transient global ischemia continues to be an important clinical problem with limited treatment options (Lo et al., 2003). It is a consequence of severe arrhythmia, cardiac arrest and hemorrhagic shock, leading to a delayed neuronal damage in the hippocampus and the cerebral cortex (Kohno et al., 1997). Global brain ischemia leads to cell death in hippocampal CA1 pyramidal neurons and cortical neurons in the third to fifth layers of the cerebral cortex, starting 2~3 days after reperfusion (Katsuta et al., 2003). It also induces excessive nitric oxide production, a major free radical, which causes neuronal death after ischemic injury (Moro et al., 2004).
Nitric oxide (NO) is a signaling molecule in the central nervous system, with functions as a neurotransmitter, as well as a regulator of cerebral blood flow (Idecola 1997). NO is generated from L-arginine by nitric oxide synthase (NOS). It may have both neuroprotective and neurotoxic functions in the ischemic brain. NOS can be classified into three groups, numbered in the order in which they were cloned. The endothelial NOS (eNOS) expressed in the endothelial cells and the NO generated by this enzyme, has been suggested to have beneficial effects (Raghavan & Dikshit, 2004). eNOS not only promotes vascular dilation but also increases vascular smooth muscle cell proliferation and migration, and thereby enhances arteriogenesis after stroke (Cui et al., 2009). Therefore, the regulation of eNOS may play an important role in ischemia-reperfusion injury. On the contrary, the overproduction of NO by inducible NOS (iNOS) has been tightly linked to neuroinflammation and neurodegeneration associated with ischemic injury (Moro et al., 2004). It can also participate in neuronal damage occurring in the late phase of focal ischemia (Iadecola 1997). Agmatine, which is synthesized from the decarboxylation of L-arginine by arginine decarboxylase (ADC), is capable of inhibiting iNOS activity (Blantz et al., 2000; Raasch et al., 2001) and protected the neurons from NO induced toxicity by inhibiting its production in the microglia (Abe et al., 2000). Agmatine also has been shown to exert neuroprotective properties against ischemia-hypoxia injury (Feng et al., 2002; Kim et al., 2004; Lee et al., 2009; Kim et al., 2010; Mun et al., 2010).
So far data suggest that extracellular pathophysiology may also influence cerebral ischemic outcomes. One of evidence supporting this idea was the finding that mice deficient in tissue plasminogen activator were protected against hippocampal injury after kainic acid injections (Tsirka et al., 1996). It has been shown that another class of extracellular proteases, the matrix metalloproteinases (MMPs), may also degrade extracellular matrix and trigger anoikis-like cell death in neurons (Gu et al., 2002). MMPs comprise an important family of proteases associated with basement membrane and extracellular matrix remodeling and are involved in both physiological and pathological CNS processes (Pagenstecher et al., 1998; Yong et al., 1998). In particular, the gelatinases (MMP-2 and MMP-9) have been implicated specifically in cerebral ischemia (Lo et al., 2002). Recently, it has been suggested that MMPs may be upregulated in hippocampus after global brain ischemia (Rivera et al., 2002; Zalewska et al., 2002).
Agmatine was shown to stimulate nitrite formation as well as Ca2+ uptake in endothelial cells (Joshi et al., 2007). It was initially speculated that agmatine might function as an alternative substrate for eNOS and contribute towards vasodilation by generating NO (Morrissey & Klahr, 1997). We previously reported exogenous agmatine treatment attenuated the MMP-2 and MMP-9 expression and this expression was mediated by the increase of eNOS after oxygen-glucose depriviation (OGD) and reperfusion injury in the cerebral endothelial cells (Yang et al., 2007). Moreover, agmatine treatment decreased the expression of MMP-2 and MMP-9 after transient focal cerebral ischemia in mice (Kim et al., 2008). However, changes in regional distribution and the timing of eNOS expression by agmatine treatment have not been fully characterized, especially during the early time after cerebral ischemia/reperfusion.
This study examines the effect of agmatine on the eNOS and MMPs levels in rat brain at the immediate (6 h) and 24 h postischemic periods. A global ischemia model was used because it more closely matches the clinical situation in humans. We used complementary immunohistochemical and immunoblot approaches to characterize the effect of ischemia on the parietal cortex and hippocampus.
A total of 22 healthy, adult, specific pathogen-free, male Sprague Dawley rats, aged 9~11 weeks and weighing 300~350 g, were obtained from Sam tako, INC. (Osan, Korea). All animal procedures were carried out according to a protocol approved by the Yonsei University Animal Care and Use Committee, which was in accordance with the NIH guidelines. All experiments attempted to minimize the number of animals used and their suffering.
Transient global cerebral ischemia was induced with four vessel occlusion (4-VO), as described previously (Pulsinelli & Brierley, 1979). In brief, animals were anesthetized with 3% isoflurane and maintained at a level of 2% isoflurane in 70% N2O and 30% O2 with spontaneous breathing during surgery. The skin was incised on the neck and the connective tissue and muscles were gently pulled aside. The right and left alar foramina of the first cervical vertebrae were exposed, and then both vertebral arteries were electrocauterized and permanently occluded. On the following day, both common carotid arteries (CCA) were occluded with aneurysm clips to induce cerebral ischemia for 20 minutes. During surgery and for 2 h into the recovery period, rectal temperature was controlled at 37±0.5℃ with a feedback-regulated heating blanket (Homeothermic Blanket Control Unit, Harvard apparatus; Edenbridge, UK). An EEG was monitored to ensure isoelectricity after carotid artery occlusion. The animals were randomized into three groups including a normal control (n=10) and two global ischemic groups: global ischemia with agmatine treatment (Agm, n=6) or without agmatine treatment (EC, n=6). The rats in global ischemic groups were euthanized at 6, and 24 hours after ischemia.
Agmatine was dissolved in normal saline (100 mg/kg IP, Sigma, St Louis, MO, USA) and administered with CCA reperfusion. Controls received normal saline in equivalent volumes. Dose of agmatine was based on previous experience which was shown to be neuroprotective (Feng et al., 2002; Kim et al., 2004 and 2010; Kim et al., 2006; Mun et al., 2009).
Histological study was conducted at 6, and 24 hours (n=5/NC, n=3/EC and Agm per each time point) after reperfusion. Animals were perfused transcardially with 200 mL of saline followed by 300 mL of 4% paraformaldehyde solution and were decapitated. Brains were removed and postfixed in the same paraformaldehyde solution for days and then embedded in paraffin. Five µm sections were cut through the dorsal hippocampus (anteroposterior coordinate, bregma - 3.8 mm) on a microtome (Microm, Germany). Brain sections were stained with hematoxylin-eosin (H&E) and dehydrated by immersing in serial ethanol baths, and they were then mounted with Vecta Mount (Vector Laboratories Inc, CA, USA).
Brain sections were immersed in a citric buffer, pH 6.0, heated in a microwave oven for 15 min, and labeled with an in situ Cell Death Detection Kit (Roche, Indianapolis, IN, USA). A mixture of Fluorescein-labeled nucleotides and terminal deoxynucleotidyl transferase was applied on sections for 60 min at 37℃ in a dark humidified chamber as previously described (Mun et al., in press). The stains were observed under a fluorescence microscope (LSM 510 META, Carl Zeíss, Germany).
The expressions of eNOS, iNOS and MMPs proteins in global cerebral ischemia were estimated by standard protocol (Lee et al., 2001). Briefly, the cerebral cortex in rat brains was dissected, homogenized to extract cellular proteins with a lysis buffer containing 1× PBS, 1% nonidet P-40, 0.5% sodium deoxycholate, 0.1% SDS, proteinase inhibitor (PMSF, aprotinin and sodium orthovanadate) and centrifuged at 13,000 rpm for 17 min at 4℃. The protein concentration in the supernatant was determined by the BCA method (PIERCE, Rockford, USA). Equal amounts of protein (40 µg) per condition were separated on 10% SDS-polyacrylamide gel electrophoresis (PAGE) and electrotransferred onto Immobilon-NC membranes (Milipore, Bedford, MA, USA). Immunoblotting was performed using anti-eNOS (Transduction Lab, Hercules, CA, USA; 1 : 1,000), anti-iNOS (Chemicon, Temecula, CA, USA; 1 : 5,000), anti-MMP-2, -9 (Chemicon Temecula, CA, USA; 1 : 2,000) and anti-actin (Santa Cruz Biotech, Santa Cruz, CA, USA; 1 : 1,000). Immunoreactive bands were visualized with the ECL detection system (Amersham Life Science, Buckinghamshire, UK) using X-ray film (Kodak, Rochester, NY, USA). Optical density of the bands was quantified in a computer-assisted image analysis system (NIH Image J), and normalized with respect to beta actin.
Brain sections were rehydrated and immunostained with primary antibodies against eNOS (Transduction Lab, Hercules, CA, USA; 1 : 500) followed by an appropriate biotinylated secondary antibody. Stains were visualized using the ABC kit (Vector Laboratories Inc, CA, USA; Lee et al., 2001), then reacted with diaminobenzidine (DAB; Sigma, St. Louis, USA) as a substrate and counterstained with hematoxylin. Images were observed and captured on an Olympus AX80 microscope equipped with a DP-70 digital camera (Olympus, Japan). All incubation steps were performed in a humidified chamber.
At 6 and 24 hours, the rats were euthanized with five animals per NC, three animals per EC and Agm used for cell count study. Brain structures were identified according to a standard rat brain atlas (Paxinos & Watson, 1998). Sections were prepared through dorsal hippocampus (anteroposterior coordinate, bregma - 3.8 mm). All of the data for immunohistochemistry were collected from 4 regions, which are 2 regions (CA1 sector of both hippocampus, magnification ×200), 2 regions (parietal cortex of both hemisphere, magnification ×100) per animal. The positive cells of eNOS were identified based on stained endothelium of vessels and capillaries per each animal. All histological preparations were analyzed by a blinded investigator with the aid of Image J software (NIH).
First, hematoxylin and eosin was used to stain the brain tissues described in the method to observe pathological alterations after global ischemia. And TUNEL staining was performed to confirm the patterns of neuronal injury observed in the hippocampus.
The results demonstrated that normal neurons had round and pale stained nuclei (Fig. 1Aa), whereas dying neurons showed pyknotic nuclei in the hippocampus (Fig. 1Ab) and had already vacuolated in the parietal cortex (Fig. 1Ah). H-E staining showed eosinophilia which is one of the earliest histochemical markers of neuronal degeneration after global ischemia. In the well-studied hippocampal CA1 region, the severity of the neuronal degeneration due to the ischemic insults was observed with eosinophilic neurons as early as 6 h postischemia (Fig. 1Ab). But in the agmatine treatment group, few eosinophilic neurons were observed in the hippocampus (Fig. 1Ac). For cortical neurons, many pyramidal neurons were observed in the parietal cortex of the control group (Fig. 1Ag). While marked morphological changes were visualized in the untreated group: neuronal loss, nuclei shrinkage, neuronal vacuolization, and dark staining of neurons, agmatine treatment markedly attenuated these pathological changes at 6 h postischemia in the parietal cortex (Fig. 1Ah and Ai). Moreover, few TUNEL-positive cells were shown in the agmatine treatment group compared with the untreated group in the hippocampal CA1 region at 6 h postishcmeia (Fig. 1Ae and Af).
At 24 h after ischemia the neuronal damage was more severe than at 6 h postischemia in both H-E and TUNEL staining (Fig. 1Bb, Be and Bh). At this time point cell loss and ghost cells were noted in the CA1 hippocampal sector and a large population of cortical neurons was also damaged. However, agmatine treatment attenuated CA1 cell death and cortical neuronal damage at 24 h postischemia compared with the untreated group (Fig. 1Bc, Bf and Bi).
At 6 h after transient global ischemia, eNOS level was decreased compared with the control level in the hippocampus (Fig. 2A and B). However, agmatine treatment increased the ischemia-induced decrease in eNOS at 6 h postischemia, by 1.79 fold compared with experimental group in the hippocampus. Densitometric data revealed that global ischemia induced a significant increase in the eNOS level at 24 h, by 1.70 fold compared with 6 h postischemia (Fig. 2B). Agmatine treatment sustained the increased eNOS level during 24 h after global ischemia.
The rats exhibited an increase the level of iNOS at 6 h postischemia, by 2 folds in the hippocampus compared with the control (Fig. 2C, D). Moreover, iNOS protein levels were gradually increased following the reperfusion periods in spite of the early phase of ischemia/reperfusion injury. However, agmatine treatment decreased iNOS level by 13.35% at 6 h and more effectively by 46.3% at 24 h in the hippocampus compared with the untreated animals after ischemia.
Endothelial NOS level was also a little decreased compared with the control level in the parietal cortex at 6 h postischemia (Fig. 3A, B). But, agmatine treatment increased the ischemia-induced decrease in eNOS by 1.3 fold in the parietal cortex. Densitometric data revealed that global ischemia significantly induced the eNOS level by 1.44 fold at 24 h after ischemia compared with 6 h postischemia (Fig. 3B). Agmatine treatment sustained the increased eNOS level by 1.33 fold at 6 h, but reduced eNOS level by 23.64 % at 24 h compared with the untreated global ischemia.
The level of iNOS was increased by 1.55 fold in the parietal cortex at 6 h postischemia, compared with the control (Fig. 3C, D). It was also gradually increased following the reperfusion periods in the cortex similar to the hippocampus. However, agmatine treatment decreased iNOS level at 6 h and significantly reduced the level by 45.5% at 24 h after global ischemia in the parietal cortex compared with the untreated animals.
In the control rats, eNOS positive cells with nuclear staining were localized to blood vessels in the hippocampus and parietal cortex (Fig. 4A). Those positive cells were seen in the endothelial layers of large vessels and many capillaries, especially in the stratum radiatum of the hippocampus and the entire parietal cortex. In the ischemia groups, a few microvessels, including capillaries and arteriolae, faintly expressed eNOS immunoreactivity in the hippocampus (Fig. 4Ab) and parietal cortex (Fig. 4Ae) at 6 h after CCA occlusion. However, agmatine treatment resulted in an increase of eNOS immunoreactivity compared with ischemia alone (Fig. 4Ac and Af).
At 24 h after transient global ischemia, a number of eNOS positive microvessels were observed both in the untreated animals and agmatine treatment group (Fig. 4Ah~Ai and 4Ak~Al). This immunoreactivity was significantly higher than at 6 h postischemia. In addition, most microvessels expressed more intense eNOS immunoreactivity in the agmatine treatment group compared with the untreated group (Fig. 4Ai and Al).
Evaluated by counting, the number of eNOS positive microvessels per microscopic field was 11.33±5.03 and 20±14 in the hippocampus and parietal cortex of the control group, respectively (Fig. 4B, C). At 6 h postischemia, a few microvessels expressed eNOS immunoreactivity in the hippocampus and parietal cortex, but the numbers significantly increased by 3.47 fold in the hippocampus and 2.23 fold in the parietal cortex of the agmatine treatment group, compared with the untreated groups.
Marked induction in the number of eNOS positive microvessels was found at 24 h after ischemia, 31.67±10.06 and 104.67±48.21 in the hippocampal CA1 region and parietal cortex, respectively. The number of eNOS positive mircovessels was a little increased 33.33±14.29 in the hippocampus and 118±26.45 in the parietal cortex with the agmatine treatment, compared with the untreated groups.
In normal brains, 72 kDa forms of MMP-2 were present as well as MMP-9 at a low density in the hippocampus (Fig. 5A). Significant elevations in MMP-2 were not observed until 24 h after ischemia whereas MMP-9 level was significantly increased until 24 h after ischemia (Fig. 5B). Agmatine treatment decreased MMP-9 expression at 24 h in the hippocampus after ischemia.
In cerebral cortex after global ischemia, the level of MMP-2 was increased in ischemic control group as well as MMP-9 during 24 h after ischemia (Fig. 5C). However, agmatine treatment decreased MMP-9 expression at 6 h and maintained the level during 24 h in the cerebral cortex after ischemia.
In the present study, H-E staining and TUNEL showed that agmatine provides a robust histological protection of selectively vulnerable hippocampal CA1 region and parietal cortex after global ischemia. According to previous reports, agmatine treatment reduced infarct sizes (Kim et al., 2004) and brain edema at 22 h after 2 h of middle cerebral artery occlusion (MCAO) in mice (Kim et al., 2010), and also protected neurons in cortical layer III~V cortex following global ischemia (Mun et al., 2010) and this result consistent with other reports.
Agmatine, formed by the decarboxylation of L-arginine by ADC, has been shown to exert certain neuromodulatory functions in the central nervous system (Li et al., 1994). Being structurally similar to arginine, agmatine may protect the brain from ischemic injury by interfering with nitric oxide signaling. Agmatine has been shown to be a competitive inhibitor of both neuronal NOS (nNOS) and iNOS (Auguet et al., 1995), but the relationship between agmatine and endothelial cells to synthesize NO, where NO is a vasodilator in cultured endothelial cells (Morrissey & Klahr, 1997) has not been clearly elucidated. During ischemia reperfusion injury, NO produced mainly by eNOS is known to play an important role in the regulation of vascular reactivity, and exerts a protective role within the vascular system by its vasodilatory effect. A NO-dependent delayed vasodilation has been observed 12 to 24 hours after cerebral hypoxic ischemia in fetal sheep, and a blockade of this response is correlated with increased cellular injury (Marks et al., 1996). Moreover, in adult models of focal cerebral ischemia, early exposure to NO donors appears to attenuate injury, whereas infarct size is enlarged in eNOS-deficient mice (Atochin et al., 2003). A rapid increase in eNOS level is consistent with the potential beneficial role of this enzyme during the initial recovery period after transient global ischemia. In addition, it was initially speculated that agmatine might function as an alternative substrate for eNOS and contribute towards relaxation (Santhanam et al., 2007). We previously demonstrated that agmatine induced the expression of eNOS and appeared to act directly on endothelial cells to increase the synthesis of NO in cerebral endothelial cells after OGD or ischemic-like injury (Yang et al., 2007). In the present study, a significant increase in eNOS levels was observed at 6 h after global cerebral ischemia with agmatine treatment both in rat hippocampus and parietal cortex. Using immunohistochemical study, which is a semiquantitative technique, the eNOS level has been reported to increase rapidly in the cerebral cortex and striatum after focal cerebral ischemia in rodents (Veltkamp et al., 2002). In our study, the number of eNOS positive microvessels were increased both 6 and 24 h after 4-VO occlusion with agmatine treatment. However, the physiologic significance of this selective increase of eNOS is unclear. We present evidence that agmatine activates eNOS expression in the early time of ischemia/reperfusion injury in the in vivo model.
In contrast, iNOS has been implicated as an important mediator of inflammatory responses during ischemia and reperfusion (Moro et al., 2004). Induction of iNOS results in delayed neuronal cell death and also exacerbates glutamate toxicity (Huan 2004). Also, NO derived from iNOS and its oxidative byproduct peroxynitrite are thought to contribute to neuronal death occurring in the late phase of cerebral ischemia (Iadecola 1997; Pannu & Singh, 2006). According to our previous studies, agmatine treatment reduced the number of iNOS immunopositive cells in a mouse model of transient focal cerebral ischemia (Kim et al., 2004) as well as transient global ischemia in the hippocampal CA1 region (Mun et al., 2009) and cerebral cortex (Mun et al., 2010). However, the protein level of iNOS was not investigated at the early time of transient global cerebral ischemia in our previous report. During ischemia and reperfusion, the expression of NOSs and the level of NO production by eNOS and iNOS can be regulated differentially in neurons and endothelial cells. In addition, the time course of NOS isoform activity shows to be delicately controlled and reliant on the period of ischemic injury. In this study, global ischemia resulted in an increase of iNOS protein level during 24 h. However, agmatine treatment decreased iNOS level since 6 h and significantly at 24 h postischemia both in the hippocampus and parietal cortex. Agmatine modulates iNOS expression after global ischemia even though at the early time of injury. Briefly, agmatine treatment induces the expression of eNOS and lessens iNOS expression in the early phase of cerebral ischemia.
We previously reported agmatine inhibits MMP-2 and MMP-9 expression via eNOS in cerebral endothelial cells (Yang et al., 2007). In addition, we suggested that exogenous and retrovirus induced endogenous agmatine inhibited MMP-2 and MMP-9 protein expression mediated by eNOS, NO and ATF3 expression in brain capillary endothelial cells (Jung et al., 2010). We also observed a decrease of MMP-9 expression with agmatine treatment in the hippocampus and cortex after transient global ischemia in the present study. The above results were in well corroboration with our previous findings in vitro (Yang et al., 2007; Jung et al., 2010). A role for MMPs in brain injury after focal ischemia and trauma has been proposed to maintaining the vascular extracellular matrix, a constituent of the basal lamina constructing the integrity of blood brain barrier (BBB) (Yong et al., 1998; Lo et al., 2002; Rosenberg 2002). MMPs, especially MMP-2 and MMP-9, degrade the basal lamina that surrounds the cerebral blood vessels in ischemic condition. At least in mouse systems, a dominant role has been ascribed to MMP-9 because MMP-9 knock-out mice were protected against ischemia and trauma (Wang et al., 2000; Asahi et al., 2001b), whereas MMP-2 knock-out mice were not protected (Asahi et al., 2001a). Here we demonstrated that MMP-9 is upregulated in postischemic hippocampus and cerebral cortex even though the expression of MMP-2 is not significant versus MMP-9 against global ischemic insult.
Our study demonstrated that an exogeneous agmatine treatment produced neuroprotection after transient global ischemia. However, it was administered without agmatine in a critical time for the development of cell death program, which starts acutely after global ischemia. Therefore, it seems that the application of agmatine treatment in the acute post-ischemic phase may be both required and sufficient for the therapeutic effect.
The question remains as to whether NOS-derived NO is eNOS-dominant, nNOS-dominant or iNOS-dominant after ischemia/reperfusion injury. A rapid increase in eNOS levels is consistent with the potential beneficial role of this enzyme during the initial recovery period after transient global ischemia. Thus, future studies should seek to define the stimulatory effect of agmatine on eNOS and the regulation of vascular homeostasis. Determination of the therapeutic window of opportunity for agmatine after transient global ischemia also warrants further investigation.
Figures and Tables
Fig. 1
Neuronal damages in rat hippocampus (a~f) and cortex (g~i) at 6 (A) and 24 h (B) after transient global ischemia. H-E and TUNEL staining were analyzed for histology, especially in the CA1 region of hippocampus and in the parietal cortex of cerebral cortex. NC, normal control; EC, experimental controls without agmatine treatment; Agm, agmatine treatment groups. Arrows point to necrotic and apoptotic neurons compared with the control. Scale bar=100 µm.
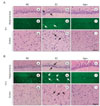
Fig. 2
Effects of eNOS and iNOS expression by agmatine treatment following transient global ischemia in rat hippocampus. Representative expressional levels of eNOS (A) and iNOS (C) at 6 h after agmatine treatment (100 mg/kg, i.p), and densitometric data (B, D). Data represent means±SD for n=5/NC, n=3/EC and Agm group per each time point. *P<0.05, vs. normal control; †P<0.05, vs. experimental control at same onset of reperfusion. NC, normal control; EC6, at 6 h after reperfusion without agmatine; Agm6, at 6 h after reperfusion with agmatine; EC24, at 24 h after reperfusion without agmatine; Agm24, at 24 h after reperfusion with agmatine.
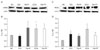
Fig. 3
Effects of eNOS and iNOS expression by agmatine treatment following transient global cerebral ischemia in rat cerebral cortex. Representative expressional levels of eNOS (A) and iNOS (C) at 24 h after agmatine treatment (100 mg/kg, i.p), and densitometric data (B, D). Data represent means±SD for n=5/NC, n=3/EC and Agm group per each time point. *P<0.05, vs. normal control; †P<0.05, vs. experimental control at same onset of reperfusion. NC, normal control; EC6, at 6 h after reperfusion without agmatine; Agm6, at 6 h after reperfusion with agmatine; EC24, at 24 h after reperfusion without agmatine; Agm24, at 24 h after reperfusion with agmatine.
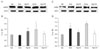
Fig. 4
Photomicrographs of eNOS expression by agmatine treatment (A) and quantification of eNOS positive cells (B, C) in rat brain after global ischemia. Representative expressional levels of eNOS in bregman - 3.8 mm of naïve rats at 6 and 24 h after agmatine treatment (A). The number of eNOS positive cells in the CA 1 sector of hippocampus (B) and in the parietal region of the cerebral cortex (C). Data represent means±SD for n=5/NC, n=3/EC and Agm group per each time point. *P<0.05, vs. normal control; †P<0.05, vs. experimental control at same onset of reperfusion. NC, normal control; EC6, at 6 h after reperfusion without agmatine; Agm6, at 6 h after reperfusion with agmatine; EC24, at 24 h after reperfusion without agmatine; Agm24, at 24 h after reperfusion with agmatine; SO, stratum oriens; SP, stratum pyramidale; SR, stratum radiatum; HiF, hippocampal fissure. Higher magnifications of cells indicated by squares show eNOS positive cells in the endothelium of vessels and capillaries. Arrows point to positive cells that showed higher intensity of immunostaining with hematoxylin in rat brain, compared with the control. Scale bar=50 µm (hippocampus), and 100 µm (cortex).
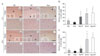
Fig. 5
Regulations of MMPs expression by agmatine treatment following transient global ischemia in rat brain. Representative expressional levels of MMP-2 and MMP-9 6 and 24 h after agmatine treatment (100 mg/kg, i.p) in the hippocampus (A), in the cerebral cortex (C), and densitometric data (B, D). Data represent means±SD for n=5/NC, n=3/EC and Agm group per each time point. *P<0.05, vs. normal control. NC, normal control; EC6, at 6 h after reperfusion without agmatine; Agm6, at 6 h after reperfusion with agmatine; EC24, at 24 h after reperfusion without agmatine; Agm24, at 24 h after reperfusion with agmatine.
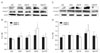
Acknowledgments
This work was supported by Mid-career Researcher Program through NRF grant funded by the MEST (No. 2009-0052626).
References
1. Abe K, Abe Y, Saito H. Agmatine suppresses nitric oxide production in microglia. Brain Res. 2000. 872:141–148.
2. Asahi M, Sumii T, Fini ME, Itohara S, Lo EH. Matrix metalloproteinase 2 gene knockout has no effect on acute brain injury after focal ischemia. Neuroreport. 2001a. 12:3003–3007.
3. Asahi M, Wang X, Mori T, et al. Effects of matrix metalloproteinase-9 gene knock-out on the proteolysis of blood-brain barrier and white matter components after cerebral ischemia. J Neurosci. 2001b. 21:7724–7732.
4. Atochin DN, Clark J, Demchenko IT, Moskowitz MA, Huang PL. Rapid cerebral ischemic preconditioning in mice deficient in endothelial and neuronal nitric oxide synthases. Stroke. 2003. 34:1299–1303.
5. Auguet M, Viossat I, Marin JG, Chabrier PE. Selective inhibition of inducible nitric oxide synthase by agmatine. Jpn J Pharmacol. 1995. 69:285–287.
6. Blantz RC, Satriano J, Gabbai F, Kelly C. Biological effects of arginine metabolites. Acta Physiol Scand. 2000. 168:21–25.
7. Cui X, Chopp M, Zacharek A, Zhang C, Roberts C, Chen J. Role of endothelial nitric oxide synthetase in arteriogenesis after stroke in mice. Neuroscience. 2009. 159:744–750.
8. Feng Y, Piletz JE, Leblanc MH. Agmatine suppresses nitric oxide production and attenuates hypoxic-ischemic brain injury in neonatal rats. Pediatr Res. 2002. 52:606–611.
9. Gu Z, Kaul M, Yan B, et al. S-nitrosylation of matrix metalloproteinases: signaling pathway to neuronal cell death. Science. 2002. 297:1186–1190.
10. Huang PL. Nitric oxide and cerebral ischemic preconditioning. Cell Calcium. 2004. 36:323–329.
11. Iadecola C. Bright and dark sides of nitric oxide in ischemic brain injury. Trends Neurosci. 1997. 20:132–139.
12. Joshi MS, Ferguson TB Jr, Johnson FK, Johnson RA, Parthasarathy S, Lancaster JR Jr. Receptor-mediated activation of nitric oxide synthesis by arginine in endothelial cells. Proc Natl Acad Sci U S A. 2007. 104:9982–9987.
13. Jung HJ, Yang MZ, Kwon KH, et al. Endogenous agmatine inhibits cerebral vascular matrix metallo-proteinases expression by regulating activating transcription factor 3 and endothelial nitric oxide synthesis. Curr Neurovasc Res. 2010. 7:201–212.
14. Katsuta K, Umemura K, Ueyama N, Matsuoka N. Pharmacological evidence for a correlation between hippocampal CA1 cell damage and hyperlocomotion following global cerebral ischemia in gerbils. Eur J Pharmacol. 2003. 467:103–109.
15. Kim DJ, Kim DI, Lee SK, et al. Protective effect of agmatine on a reperfusion model after transient cerebral ischemia: temporal evolution on perfusion MR imaging and histopathologic findings. AJNR Am J Neuroradiol. 2006. 27:780–785.
16. Kim JH, Lee YW, Kim JY, Lee WT, Park KA, Lee JE. The effect of agmatine on expression of MMP2 and MMP9 in Cerebral ischemia. Korean J Anat. 2008. 41:97–104.
17. Kim JH, Lee YW, Park KA, Lee WT, Lee JE. Agmatine attenuates brain edema through reducing the expression of aquaporin-1 after cerebral ischemia. J Cereb Blood Flow Metab. 2010. 30:943–949.
18. Kim JH, Yenari MA, Giffard RG, Cho SW, Park KA, Lee JE. Agmatine reduces infarct area in a mouse model of transient focal cerebral ischemia and protects cultured neurons from ischemia-like injury. Exp Neurol. 2004. 189:122–130.
19. Kohno K, Higuchi T, Ohta S, Kohno K, Kumon Y, Sakaki S. Neuroprotective nitric oxide synthase inhibitor reduces intracellular calcium accumulation following transient global ischemia in the gerbil. Neurosci Lett. 1997. 224:17–20.
20. Lee JE, Yenari MA, Sun GH, et al. Differential neuroprotection from human heat shock protein 70 overexpression in in vitro and in vivo models of ischemia and ischemia-like conditions. Exp Neurol. 2001. 170:129–139.
21. Lee WT, Hong S, Yoon SH, et al. Neuroprotective effects of agmatine on oxygen-glucose deprived primary-cultured astrocytes and nuclear translocation of nuclear factor-kappa B. Brain Res. 2009. 1281:64–70.
22. Li G, Regunathan S, Barrow CJ, Eshraghi J, Cooper R, Reis DJ. Agmatine: an endogenous clonidine-displacing substance in the brain. Science. 1994. 263:966–969.
23. Lo EH, Dalkara T, Moskowitz MA. Mechanisms, challenges and opportunities in stroke. Nat Rev Neurosci. 2003. 4:399–415.
24. Lo EH, Wang X, Cuzner ML. Extracellular proteolysis in brain injury and inflammation: role for plasminogen activators and matrix metalloproteinases. J Neurosci Res. 2002. 69:1–9.
25. Marks KA, Mallard CE, Roberts I, Williams CE, Gluckman PD, Edwards AD. Nitric oxide synthase inhibition attenuates delayed vasodilation and increases injury after cerebral ischemia in fetal sheep. Pediatr Res. 1996. 40:185–191.
26. Moro MA, Cárdenas A, Hurtado O, Leza JC, Lizasoain I. Role of nitric oxide after brain ischaemia. Cell Calcium. 2004. 36:265–275.
27. Morrissey JJ, Klahr S. Agmatine activation of nitric oxide synthase in endothelial cells. Proc Assoc Am Physicians. 1997. 109:51–57.
28. Mun CH, Kim JH, Park KA, Lee WT, Baik JH, Lee JE. Agmatine attenuates nitric oxide synthesis and protects ER-structure from global cerebral ischemia in rats. Korean J Anat. 2009. 42:149–160.
29. Mun CH, Lee WT, Park KA, Lee JE. Agmatine reduced the expressions of nitric oxide synthase and peroxynitrite formation in rat cerebral cortex after transient global cerebral ischemia. Neural Regen Res. In Press.
30. Pagenstecher A, Stalder AK, Kincaid CL, Shapiro SD, Campbell IL. Differential expression of matrix metalloproteinase and tissue inhibitor of matrix metalloproteinase genes in the mouse central nervous system in normal and inflammatory states. Am J Pathol. 1998. 152:729–741.
31. Pannu R, Singh I. Pharmacological strategies for the regulation of inducible nitric oxide synthase: neurodegenerative versus neuroprotective mechanisms. Neurochem Int. 2006. 49:170–182.
32. Paxinos G, Watson C. The rat brain in stereotaxic coordinates. 1998. 4th ed. New York: Academic Press.
33. Pulsinelli WA, Brierley JB. A new model of bilateral hemispheric ischemia in the unanesthetized rat. Stroke. 1979. 10:267–272.
34. Raasch W, Schäfer U, Chun J, Dominiak P. Biological significance of agmatine, an endogenous ligand at imidazoline binding sites. Br J Pharmacol. 2001. 133:755–780.
35. Raghavan SA, Dikshit M. Vascular regulation by the L-arginine metabolites, nitric oxide and agmatine. Pharmacol Res. 2004. 49:397–414.
36. Rivera S, Ogier C, Jourquin J, et al. Gelatinase B and TIMP-1 are regulated in a cell- and time-dependent manner in association with neuronal death and glial reactivity after global forebrain ischemia. Eur J Neurosci. 2002. 15:19–32.
37. Rosenberg GA. Matrix metalloproteinases in neuroinflammation. Glia. 2002. 39:279–291.
38. Santhanam AV, Viswanathan S, Dikshit M. Activation of protein kinase B/Akt and endothelial nitric oxide synthase mediates agmatine-induced endothelium-dependent relaxation. Eur J Pharmacol. 2007. 572:189–196.
39. Tsirka SE, Rogove AD, Strickland S. Neuronal cell death and tPA. Nature. 1996. 384:123–124.
40. Veltkamp R, Rajapakse N, Robins G, Puskar M, Shimizu K, Busija D. Transient focal ischemia increases endothelial nitric oxide synthase in cerebral blood vessels. Stroke. 2002. 33:2704–2710.
41. Wang X, Feuerstein GZ. Role of immune and inflammatory mediators in CNS injury. Drug News Perspect. 2000. 13:133–140.
42. Yang MZ, Mun CH, Choi YJ, et al. Agmatine inhibits matrix metalloproteinase-9 via endothelial nitric oxide synthase in cerebral endothelial cells. Neurol Res. 2007. 29:749–754.
43. Yong VW, Krekoski CA, Forsyth PA, Bell R, Edwards DR. Matrix metalloproteinases and diseases of the CNS. Trends Neurosci. 1998. 21:75–80.
44. Zalewska T, Ziemka-Nacz M, Sarnowska A, Domańska-Janik K. Involvement of MMPs in delayed neuronal death after global ischemia. Acta Neurobiol Exp (Wars). 2002. 62:53–61.