Abstract
Oxidative stress-induced cell death leads to phosphorylation of 14-3-3ζ at serine 58. 14-3-3ζ is detected at significant levels in cerebrospinal fluid after kainic acid (KA)-induced seizures. Here we examined temporal changes in 14-3-3ζ phosphorylation in the hippocampus and amygdala of mice after KA treatment. Mice were killed at 2, 6, 24, or 48 h after KA (30 mg/kg) injection. We observed an increase in TUNEL and Fluoro-Jade B (FJB)-stained neurons in the hippocampus and amygdala of KA-treated mice. Phospho (p)-14-3-3ζ and p-JNK expression was increased in the hippocampus 2 and 6 h after KA treatment, respectively. In immunohistochemical analysis, p-14-3-3ζ-positive cells were present in the CA3 region of the hippocampus and the central nucleus of amygdala (CeA) of KA-treated mice. Thus, phosphorylation of 14-3-3ζ at serine 58 may play an important role in KA-induced hippocampal and amygdaloid neuronal damage.
Kainic acid (KA) treatment induces seizures, followed by hippocampal cell death via oxidative stress (Evans et al., 1984). This neuronal death following KA treatment may involve specific molecular pathways characterized by apoptosis (Savitz & Rosenbaum, 1998). Control of the intrinsic cell death pathway likely involves pro- and anti-apoptotic members of the Bcl-2 family, which integrate the stress signals that target mitochondria (Green & Reed, 1998). Subsequently, mitochondrial release of apoptogenic factors activates the caspase family of proteases, leading to cell death (Thornberry & Lazebnik, 1998).
14-3-3 proteins comprise a family of seven chaperone proteins (β, α, γ, ζ, ε, θ, η) that are highly expressed in the central nervous system (Aitken et al., 1992). They regulate protein-protein interactions in pathways that regulate neuronal development, intracellular signaling, cell cycling, and apoptosis (Berg et al., 2003). 14-3-3 proteins play protective roles in the cell by sequestering proapoptotic factors in a phosphorylation-dependent manner (Machintosh 2004). For example, Bax interacts with 14-3-3 proteins in the cytosol of healthy cells. In response to insults, however, Bax dissociates from 14-3-3 and redistributes to mitochondria leading to apoptosis (Nomura et al., 2003). Some isoforms of 14-3-3 have been shown to be phosphorylated by sphingosine-dependent protein kinase (SDK) (Megidish et al., 1998). SDK1 specifically phosphorylates 14-3-3ζ at serine 58 in the presence of sphingosine (Hamaguchi et al., 2003). The interconvertible sphingolipid metabolites ceramide, sphingosine, and sphingosine-1-phosphate (S1P) regulate apoptosis and cell survival (Hait et al., 2006). Sphingosine kinase (SK) phosphorylates sphingosine and controls cellular S1P levels (Okada et al., 2009). A recent study suggested that S1P is important for triggering spontaneous glutamate secretion (Kajimoto et al., 2007).
Studies of tissues obtained from autopsies and epilepsy surgery showed that amygdaloid damage is found in 10~76% of patients with temporal lobe epilepsy (TLE) (Miller et al., 1994). Furthermore, many animal studies have provided substantial evidence that the amygdala is sensitive to status epilepticus-induced histopathological changes (Tuunanen et al., 1999).
Thus, because KA-induced oxidative stress induces massive glutamate release and is followed by hippocampal cell death, we hypothesized that 14-3-3ζ phosphorylation at serine 58 may correlate with KA-induced neuronal cell death. In this study, we investigated the temporal dynamics of 14-3-3ζ phosphorylation and localization in the hippocampus and amygdala of mice after KA treatment.
Male ICR mice (4 weeks old) were purchased from KOATECH Co. (Pyeongtaek, South Korea) and were maintained in the animal facility at the Gyeongsang National University School of Medicine. Mice were treated in accordance with standard guidelines for laboratory animal care. All mice were provided with water and standard chow ad libitum for 1 week prior to any experimental procedures, and were allowed to acclimatize to their new surroundings.
Mice were treated with an intraperitoneal injection of 30 mg/kg KA (Ascent Scientific, North Somerset, UK) emulsified in 0.9% normal saline. All animals were seizure-naïve when tested. Mice in the control group (n=6) were injected with 0.9% normal saline. Seizure behavior was monitored for 2 h after KA injection. Animals in the experimental group (n=6) were sacrificed at 2, 6, 24, or 48 h after KA treatment.
For tissue analysis, animals (n=3 per group) were perfused transcardially with heparinized saline followed by 4% paraformaldehyde in 0.1 M phosphate-buffered saline (PBS). Brains were postfixed for 6 h, and then sequentially immersed in 0.1 M PBS containing 15~30% sucrose at 4℃ until they sank. The brains were cut into 40-µm coronal sections.
Evaluation of KA-induced neuronal degeneration was performed using Fluoro-Jade B (FJB, Chemicon international, CA, USA) staining according to the manufacturer's instructions. FJB-positive, degenerating neurons were visualized using a fluorescence microscope as glowing green cells. The number of FJB-positive, degenerating neurons was counted manually.
To examine the KA-induced neuronal cell death, TUNEL kit (Roche Molecular Biochemicals, Mannheim, Germany) staining was performed according to the manufacturer's instructions. TUNEL-positive neurons were visualized using a light microscope. The number of TUNEL-positive was counted manually.
Immunohistochemical staining was used to detect phospho (p)-14-3-3ζ. Briefly, free-floating sections were incubated with rabbit anti-p-14-3-3ζ antibody (diluted 1 : 500; Santa Cruz Biotechnology, CA, USA) overnight at 4℃. After washing with 0.1 M PBS, sections were incubated for 1 h at room temperature with the appropriate secondary biotinylated antibody (1 : 200). After washing, sections were incubated in an avidin-biotin-peroxidase complex solution (ABC solution, Vector Laboratories, CA, USA). Sections were developed with 0.05% diaminobenzidine (DAB, Sigma, CA, USA) containing 0.05% H2O2. Sections were mounted on gelatin-coated slides, warmer-dried, dehydrated through a graded series of alcohols, cleared in xylene, and coverslipped with Permount (Sigma). Digital images were captured and documented with a BX51 light microscope (Olympus, Tokyo, Japan).
For Western blot analysis, the brain was removed, and the hippocampus and amygdala were dissected. Frozen samples (n=3 per group) were transferred to sterile tubes containing 200 µL of lysis buffer (15 mM HEPES, pH 7.9, 0.25 M sucrose, 60 mM KCl, 10 mM NaCl, 1 mM EGTA, 1 mM PMSF, and 2 mM NaF). Homogenized tissues were incubated for 10 min on ice and then sonicated. Samples were centrifuged and supernatants were transferred to clean vials. Protein concentrations were quantified using the Bio-Rad protein assay (Bio-Rad, USA) and samples were stored at -80℃ until analysis. For p-JNK (Cell Signaling Technology, MA, USA), total 14-3-3ζ (Santa Cruz Biotechnology) and p-14-3-3ζ protein analysis, tissue lysates (20 µg) were separated by 10% sodium dodecyl sulfate-polyacrylamide gel electrophoresis followed by electrophoretic transfer onto a polyvinylidene difluoride membrane (Millipore). The membranes were probed with each antibody and visualized using ECL substrate (Pierce, Rockford, IL).
Differences between time-dependent groups and controls were determined by one-way analysis of variance (ANOVA), followed by post hoc analysis using the Bonferroni t-test. Values are expressed as the mean±standard error of the mean (SEM). A P-value<0.05 was considered statistically significant.
We examined neuronal death in the KA-treated mice using TUNEL and FJB histofluorescence staining (Fig. 1). In the KA-treated mice, TUNEL and FJB-positive cells were observed in the CA3 region of the hippocampus, the central nucleus of amygdala (CeA), and the basolateral nucleus of the amygdala (BLA) 48 h after KA treatment (Fig. 1B). No FJB-positive cells were seen in the hippocampus of control mice (data not shown).
In a previous study, we found that the number of caspase-3-positive cells increases 6 h after KA induction (Lee et al., 2009). This previous result suggests that KA-induced cell death begins at 6 h after the first KA injection. To evaluate the altered levels of p-JNK protein in the hippocampus and amygdala after KA treatment, we used Western blot analysis (Fig. 2). From Western blot analysis of KA-treated mice, JNK phosphorylation was increased in the hippocampus 6 h after KA injection (Fig. 2A). The anti-p-JNK antibody recognized two p-JNK bands of 46 kDa and 54 kDa. The 54 kDa band was increased more than the 46 kDa band (P=0.043) (Fig. 2A). However, there was no statistically significant change in p-JNK expression in the amygdala (Fig. 2B).
To examine the temporal dynamics of p-14-3-3ζ expression and localization in the hippocampus during KA-induced excitotoxicity, we used immunohistochemistry and Western blot analysis (Fig. 3). KA-treated mice showed p-14-3-3ζ-immunostained cells in the CA3 region of the hippocampus 2 h after KA treatment (Fig. 3A). Western blot analysis showed that the levels of p-14-3-3ζ protein expression were significantly upregulated 2 h after KA treatment and thereafter its expression was maintained (Figs. 3B and C).
We also examined the temporal dynamics of p-14-3-3ζ expression and localization in the amygdala during KA-induced excitotoxicity (Fig. 4). Many p-14-3-3ζ-immunostained cells were seen in the CeA 2 h after KA treatment except the BLA where we expected to see in KA-treated mice (Fig. 4A). Western blot analysis showed that the levels of p-14-3-3ζ protein expression were significantly upregulated 6 h after KA treatment and thereafter its expression was maintained (Fig. 4B).
In a recent study, phosphorylation of 14-3-3ζ at serine 58 was suggested to lead to oxidative stress-induced cell death. In our current study, we demonstrated that serine 58 phosphorylation of 14-3-3ζ and neurodegeneration were increased in the hippocampus and amygdala of mice after KA injection. P-14-3-3ζ-immunoreactivity in the hippocampus and CeA of KA-treated mice was consistent with TUNEL and FJB-staining results. However, TUNEL and FJB-staining results were not consistent with the p-14-3-3ζ-immunoreactivity in the BLA. Thus, our results suggest that phosphorylation of serine 58 in 14-3-3ζ in the hippocampus and CeA may play an important role in neurodegeneration after KA-induced oxidative stress.
It is well known that temporal lobe limbic structures are the most susceptible regions to seizurogenic insults. Thus, TLE is the most common form of epilepsy in humans (Pitkänen & Sutula, 2002). Both the hippocampus and the amygdala display extensive neuropathology in patients with TLE (Pitkänen et al., 1998). In an animal study examining different injections of KA, both intra-amygdala microinjection and intra-peritoneal injection induce neuronal death in the amygdala and hippocampus (Tuunanen et al., 1999; Mouri et al., 2008). In the amygdala complex, the higher sensitivity of BLA to KA-induced damage may be related to the direct toxic effects of KA on the amygdaloid neurons in areas that are rich with KA receptors (Tuunanen et al., 1999). A recent report demonstrated that neuronal degeneration in the BLA is greater than in the hippocampus (Aroniadou-Anderjaska et al., 2009). In the hippocampus, we previously demonstrated that the number of caspase-3 and FJB -positive cells increases 6 h after KA induction (Kwag et al., 2008; Lee et al., 2009; Lee et al., 2010). In the present study, we showed that TUNEL and FJB-positive cells in the hippocampus, CeA, and BLA were increased after KA treatment. This data indicates that KA treatment induces neuronal damage in the amygdala as well as in the hippocampus.
c-jun N-terminal kinases (JNKs) play a fundamental role in neuronal death after KA-induced seizures and excitotoxicity. Following KA-induced oxidative stress, JNK is activated and phosphorylated in the hippocampus (Mielke et al., 1999). This JNK activation increases and reaches a maximum 6~12 h after KA injection. Another recent study showed that a high number of p-JNK-positive cells in the CA3 region 3~6 h following treatment (Spigolon et al., 2010). Furthermore, treatment with the peptide D-JNKI1, a competitive JNK inhibitor, reduced the number of degenerating neurons in the hippocampus during status epilepticus (Spigolon et al., 2010). In our current study, we found that in contrast to JNK phosphorylation in the hippocampus, there was no statistically significant change in JNK phosphorylation in the amygdala. This observation suggests that oxidative stress-induced cell damage in the amygdala may be not greater than in the KA-treated hippocampus.
JNK-mediated phosphorylation of 14-3-3 induces the release of Bax from 14-3-3 and leads to apoptosis (Tsuruta et al., 2004). This JNK-mediated phosphorylation of 14-3-3β and 14-3-3ζ was found to occur at the previously identified phosphorylation sites; serine 186 of 14-3-3β and serine 184 of 14-3-3ζ (Aitken et al., 1995; Tsuruta et al., 2004). In particular, phosphorylation of serine 58 releases apoptosis signal-regulating kinase1 (ASK1) from 14-3-3ζ, and ASK1 then activates stress-activated protein kinase, leading to cell death (Zhang et al., 1999; Zhou et al., 2009). 14-3-3ζ at serine 58 has been shown to be phosphorylated by a SDK1 (Megidish et al., 1998). S1P, which is one of SDK1-mediated sphingosine metabolites, is important for triggering spontaneous glutamate secretion and regulates apoptosis and cell survival (Kajimoto et al., 2007). Our recent previous study showed that the expression of SK1 and S1P receptor 1 (S1P1) proteins steadily increases in the hippocampus after KA treatment (Lee et al., 2010). The increase of SK1-dependent S1P1 activation is correlated with depolarization-induced glutamate secretion. Thus, we suggest that upregulation of phosphorylation of 14-3-3ζ at serine 58 may be associated sphingosine-mediated glutamate excitoxicity in this animal model.
In patients with TLE, hippocampal 14-3-3ζ levels are higher than in controls (Schindler et al., 2006). 14-3-3ζ is detected at significant levels in cerebrospinal fluid (CSF) sampled 4 h after seizures compared with almost no expression of 14-3-3ζ in control CSF (Murphy et al., 2008). These studies suggested that the presence of 14-3-3ζ within CSF might be a biomarker of acute seizure damage. In general, the BLA in the amygdala complex is involved with neurodegeneration during KA-induced cell death (Aroniadou-Anderjaska et al., 2009). There is a report that the overall severity of neuronal damage in the CeA of KA-treated mice was mild in the other amygdaloid regions (Tuunanen et al., 1999). However, we found a significant increase in p-14-3-3ζ-positive cells in the CeA after KA treatment. There was no detection of p-14-3-3ζ-positive cells in the BLA. The reason for difference of p-14-3-3ζ-immunoreactivity and neuronal damage between the CeA and the BLA after KA treatment is uncertain. Thus, we suggest that oxidative stress-dependent phosphorylation of 14-3-3ζ at serine 58 might be play an important role in neuronal damage through neural network between the CeA and the hippocampus after KA-induced excitotoxicity, but not BLA.
In summary, our results first confirmed that phosphorylation of 14-3-3ζ at serine 58 is increased in an animal model of KA-induced seizures. This 14-3-3ζ phosphorylation was upregulated in the hippocampus, and coincided with increased numbers of FJB-positive neurons after KA treatment, but these observations were not seen in the amygdala. Thus, our data suggest that further study of the actual mechanism for the regulation of phosphorylation of 14-3-3ζ at serine 58 is needed for the understanding of oxidative stress-induced neuronal damage and neurodegenerative disorders.
Figures and Tables
Fig. 1
Effect of KA treatment on neuronal death and neurodegeneration in the hippocampus and amygdala. (A) Location of hippocampal cell death in a coronal brain section 48 h after KA treatment. (CA3, Cornu ammonis 3 of the hippocampus; CeA, Central nucleus of the amygdala; BLA, Basolateral nucleus of the amygdala). (B) Photo-micrograph of TUNEL and FJB-stained cells in the CA3 region of the hippocampus, CeA, and BLA 48 h after KA treatment. Scale bars = 200 µm.
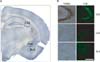
Fig. 2
Effect of KA treatment on p-JNK expression in the hippocampus and amygdala. Western blot showing the time course of p-JNK expression in the hippocampus (A) and amygdala (B) after KA treatment. p-JNK was normalized to α-tubulin and represented as arbitrary units. Data are presented as the mean±SEM. *indicates P<0.05.
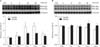
Fig. 3
Effect of KA treatment on p-14-3-3ζ expression in the hippocampus. (A) Representative micrographs showing p-14-3-3ζ immunoreactivity in mouse hippocampus after KA treatment. (B) Western blot showing the time course of p-14-3-3ζ and total 14-3-3ζ expression in the hippocampus after KA treatment. (C) p-14-3-3ζ was normalized to 14-3-3ζ and represented as ratio. Data are presented as the mean±SEM. *indicates P<0.05. Scale bar = 100 µm. Arrows indicate p-14-3-3ζ-immunostained cells from CA3 region of the hippocampus.
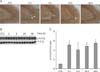
Fig. 4
Effect of KA treatment on p-14-3-3ζ expression in the amygdala. (A) Representative micrographs showing p-14-3-3ζ immunoreactivity in the CeA after KA treatment. (B) Western blot showing the time course of p-14-3-3ζ and total 14-3-3ζ expression in the hippocampus after KA treatment. (C) p-14-3-3ζ was normalized to 14-3-3ζ and represented as a ratio. Data are presented as the mean±SEM. *indicates P<0.05. Scale bar=100 µm.
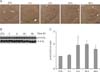
Acknowledgements
This work was supported by the National Research Foundation (NRF) of Korea grant funded the Korea government (MEST) (Nos. R13-2005-012-01001-0 and 2009-0064327).
References
1. Aitken A, Collinge DB, van Heusden BP, et al. 14-3-3 proteins: a highly conserved, widespread family of eukaryotic proteins. Trends Biochem Sci. 1992. 17:498–501.
2. Aitken A, Howell S, Jones D, Madrazo J, Patel Y. 14-3-3 alpha and delta are the phosphorylated forms of raf-activating 14-3-3 beta and zeta. In vivo stoichiometric phosphorylation in brain at a Ser-Pro-Glu-Lys MOTIF. J Biol Chem. 1995. 270:5706–5709.
3. Aroniadou-Anderjaska V, Figueiredo TH, Apland JP, Qashu F, Braga MF. Primary brain targets of nerve agents: the role of the amygdala in comparison to the hippocampus. Neurotoxicology. 2009. 30:772–776.
4. Berg D, Holzmann C, Riess O. 14-3-3 proteins in the nervous system. Nat Rev Neurosci. 2003. 4:752–762.
5. Evans MC, Griffiths T, Meldrum BS. Kainic acid seizures and the reversibility of calcium loading in vulnerable neurons in the hippocampus. Neuropathol Appl Neurobiol. 1984. 10:285–302.
6. Green DR, Reed JC. Mitochondria and apoptosis. Science. 1998. 281:1309–1312.
7. Hait NC, Oskeritzian CA, Paugh SW, Milstien S, Spiegel S. Sphingosine kinases, sphingosine 1-phosphate, apoptosis and diseases. Biochim Biophys Acta. 2006. 1758:2016–2026.
8. Hamaguchi A, Suzuki E, Murayama K, et al. Sphingosine-dependent protein kinase-1, directed to 14-3-3, is identified as the kinase domain of protein kinase C delta. J Biol Chem. 2003. 278:41557–41565.
9. Kajimoto T, Okada T, Yu H, et al. Involvement of sphingosine-1-phosphate in glutamate secretion in hippocampal neurons. Mol Cell Biol. 2007. 27:3429–3440.
10. Kwag SJ, Kim KH, Kim HJ, et al. Immunohistochemical study of glucose transporters 1 and 3 in the mouse hippocampus after kainic acid treatment. Korean J Anat. 2008. 41:213–221.
11. Lee DH, Jeon BT, Jeong EA, et al. Altered expression of sphingosine kinase 1 and sphingosine-1-phosphate receptor 1 in mouse hippocampus after kainic acid treatment. Biochem Biophys Res Commun. 2010. 393:476–480.
12. Lee JY, Jeon BT, Shin HJ, et al. Temporal expression of AMP-activated protein kinase activation during the kainic acid-induced hippocampal cell death. J Neural Transm. 2009. 116:33–40.
13. Mackintosh C. Dynamic interactions between 14-3-3 proteins and phosphoproteins regulate diverse cellular processes. Biochem J. 2004. 381:329–342.
14. Megidish T, Cooper J, Zhang L, Fu H, Hakomori S. A novel sphingosine-dependent protein kinase (SDK1) specifically phosphorylates certain isoforms of 14-3-3 protein. J Biol Chem. 1998. 273:21834–21845.
15. Mielke K, Brecht S, Dorst A, Herdegen T. Activity and expression of JNK1, p38 and ERK kinases, c-Jun N-terminal phosphorylation, and c-jun promoter binding in the adult rat brain following kainate-induced seizures. Neuroscience. 1999. 91:471–483.
16. Miller LA, McLachlan RS, Bouwer MS, Hudson LP, Munoz DG. Amygdalar sclerosis: preoperative indicators and outcome after temporal lobectomy. J Neurol Neurosurg Psychiatry. 1994. 57:1099–1105.
17. Mouri G, Jimenez-Mateos E, Engel T, et al. Unilateral hippocampal CA3-predominant damage and short latency epileptogenesis after intra-amygdala microinjection of kainic acid in mice. Brain Res. 2008. 1213:140–151.
18. Murphy N, Yamamoto A, Henshall DC. Detection of 14-3-3zeta in cerebrospinal fluid following experimentally evoked seizures. Biomarkers. 2008. 13:377–384.
19. Nomura M, Shimizu S, Sugiyama T, et al. 14-3-3 Interacts directly with and negatively regulates pro-apoptotic Bax. J Biol Chem. 2003. 278:2058–2065.
20. Okada T, Kajimoto T, Jahangeer S, Nakamura S. Sphingosine kinase/sphingosine 1-phosphate signalling in central nervous system. Cell Signal. 2009. 21:7–13.
21. Pitkänen A, Sutula TP. Is epilepsy a progressive disorder? Prospects for new therapeutic approaches in temporal-lobe epilepsy. Lancet Neurol. 2002. 1:173–181.
22. Pitkänen A, Tuunanen J, Kälviäinen R, Partanen K, Salmenperä T. Amygdala damage in experimental and human temporal lobe epilepsy. Epilepsy Res. 1998. 32:233–253.
23. Savitz SI, Rosenbaum DM. Apoptosis in neurological disease. Neurosurgery. 1998. 42:555–572.
24. Schindler CK, Heverin M, Henshall DC. Isoform- and subcellular fraction-specific differences in hippocampal 14-3-3 levels following experimentally evoked seizures and in human temporal lobe epilepsy. J Neurochem. 2006. 99:561–569.
25. Spigolon G, Veronesi C, Bonny C, Vercelli A. c-Jun N-terminal kinase signaling pathway in excitotoxic cell death following kainic acid-induced status epilepticus. Eur J Neurosci. 2010. 31:1261–1272.
26. Thornberry NA, Lazebnik Y. Caspases: enemies within. Science. 1998. 281:1312–1316.
27. Tsuruta F, Sunayama J, Mori Y, et al. JNK promotes Bax translocation to mitochondria through phosphorylation of 14-3-3 proteins. EMBO J. 2004. 23:1889–1899.
28. Tuunanen J, Lukasiuk K, Halonen T, Pitkänen A. Status epilepticus-induced neuronal damage in the rat amygdaloid complex: distribution, time-course and mechanisms. Neuroscience. 1999. 94:473–495.
29. Zhang L, Chen J, Fu H. Suppression of apoptosis signal-regulating kinase 1-induced cell death by 14-3-3 proteins. Proc Natl Acad Sci U S A. 1999. 96:8511–8515.
30. Zhou J, Shao Z, Kerkela R, et al. Serine 58 of 14-3-3zeta is a molecular switch regulating ASK1 and oxidant stress-induced cell death. Mol Cell Biol. 2009. 29:4167–4176.