Abstract
Our previous research demonstrated that calponin-immunoreactivity was localized in myofibroblasts of the periglomerular region of human kidney specimens obtained at the time of transplantation from organ recipients. In the present study we examined calponin expression in two chronic nephropathy models, puromycin aminonucleoside (PAN) nephropathy and subtotal nephrectomy (SNx), to investigate the role of calponin in chronic renal injury. Male Sprague-Dawley rats were used, and both nephropathy models were established at 1, 2, 4, and 8 weeks after surgery. There were no periglomerular calponin-positive cells in sham, PAN 1 and 2 week, and SNx 1, 2, and 4 week groups. In SNx 8 week and PAN 4 and 8 week groups, only a few glomeruli with periglomerular calponin-reactivity, which covered half or a very small part of the periglomerular space, were observed. All glomeruli with periglomerular calponin-reactivity showed sclerotic changes, especially thickening of parietal epithelial cells (PECs). In conjunction with our previous report, this data represents the first documentation of the expression of calponin in renal myofibroblasts. We suggest that interactions between PECs and calponin-positive myofibroblasts may play a key role in the late stage of glomerulosclerosis.
Progressive renal diseases lead to a common histological and functional end point referred to as end-stage renal disease. Histologically, end-stage renal disease manifests as glomerulosclerosis, vascular sclerosis, and tubulointerstitial fibrosis, with tubulointerstitial fibrosis having consistently been shown to be the most accurate histological predictor of disease progression (Bohle et al., 1987). During the development of tubulointerstitial fibrosis many types of cells accumulate in the renal interstitium. Among those cells, myofibroblasts have a major role in mediating tubulointerstitial fibrosis as they are the source of several components of extracellular materials and many cytokines that contribute to renal damage (Stahl & Felsen, 2001).
Myofibroblasts are usually immunostained using several specific markers, such as alpha-smooth muscle actin (ASMA), vimentin, and calponin in several tissues (Frangogiannis et al., 2000; Chen et al., 2009; Ferguson et al., 2009) However, to the authors' knowledge there has been no report of calponin expression in renal myofibroblasts, while calponin expression in the renal vessels, mesangial cells, and renal tumor cells has been reported (Islam et al., 2004a & b).
We recently reported for the first time that calponin-immunoreactivity was localized in the myofibroblasts of the periglomerular region of human kidney specimens obtained from kidney transplant recipients at the time of surgery but not from normal specimens obtained from kidney segments of patients undergoing nephrectomy surgery for renal tumors despite the presence of ASMA-positive myofibroblasts in the periglomerular region (Choi et al., 2008). These findings suggest that calponin may be expressed and play a role at the very final stage of glomerular damage.
Therefore, we hypothesized that renal myofibroblasts may also express calponin, especially in the periglomerular region, at the very end stage of disease in an experimentally induced animal model of chronic renal failure. Thus, in this study we aimed to examine calponin expression in two experimental models, puromycin aminonucleoside (PAN) nephropathy and subtotal nephrectomy (SNx), to investigate the role of calponin in chronic renal injury.
All experimental procedures performed on animals were conducted with the approval of the Catholic Ethics Committee of the Catholic University of Korea.
Male Sprague-Dawley rats (Orient Bio Co., Korea) weighing approximately 180 to 200 g, were housed under a 12-h light/dark cycle with food and water available ad libitum. Rats were divided into sham control, PAN nephropathy, and SNx groups. During the experimental procedures, animals were anesthetized with an intravenous injection of Zoletil (30 mg/kg; Virbac Korea, Korea) and Rompun (10 mg/kg; Bayer Korea, Korea) and placed on a temperature-regulated table. After surgery animals were returned to their cages once they recovered from anesthesia. All surgical tools were sterilized with 70% ethyl alcohol.
In order to establish the nephropathy model by reducing the renal mass, the left kidney was exposed via flank incision and a complete subcapsular nephrectomy performed under anesthesia. After closing the incision, PAN nephropathy was induced by a single intravenous injection to the femoral vein of PAN (5 mg/100 g) (Sigma Chemicals, St. Louis, Mo., USA) diluted in 0.9% saline. Sham animals underwent the same operation but received only 0.9% saline. On weeks 1, 2, 4, and 8 after PAN injections, rats (n=5 in each group at each time point) were euthanized. All sham rats were euthanized after 8 weeks (n=5).
Rats were subjected to subtotal nephrectomy under anesthesia in two operations performed 1 week apart. In the first operation the left kidney was exposed via flank incision and both superior and inferior poles of the kidney were excised with scissors leaving approximately 2/3 of the single kidney mass. Spongostan (Johnson & Johnson Medical, UK) was applied to the cut surface to control bleeding. One week later under anesthesia the right kidney was completely removed through a flank incision during a second operation. On weeks 1, 2, 4, and 8 after the second surgery, rats (n=5 in each group at each time point) were euthanized. A control group which underwent sham operations without nephrectomy were euthanized after 8 weeks (n=5).
One day before the end of the study period, rats were individually housed in metabolic cages for 24 hr for subsequent determination of urine protein excretion using the 3% sulfosalicylic acid method. Blood samples were collected from the abdominal aorta at euthanasia. Serum creatinine was determined by the Jaffe' method using creatinine reagent (BCS Co., South Korea). Blood urea nitrogen (BUN) was measured by the Urease-Indophenol method using urea-nitrogen reagent (BCS Co.)
Kidneys were fixed by perfusion with 2% paraformaldehyde, 125 mM lysine, and 10 mM periodate, pH 7.4 (PLP solution) for 10 min through the abdominal aorta. The kidneys were then removed and cut into sagittal slices 1- to 2-mm thick and postfixed overnight in PLP solution at 4℃. The fixed kidney slices were routinely processed, embedded in paraffin, and 4-um sections prepared for histological staining.
Hematoxylin-eosin and Masson's trichrome stains were performed to examine histopathological changes. To examine calponin expression and structural changes of periglomerular calponin-positive glomeruli, five serial tissue sections were stained with periodic acid-methenamine silver (PAMS), alpha-smooth muscle actin (ASMA) antiserum, calponin antiserum, vimentin antiserum, and the periodic acid-Schiff (PAS) method.
PAMS staining was performed using an ACCUSTAIN Silver Stain kit (Sigma Chemicals) according to the manufacture's protocol. For immunohistochemistry, deparaffinized sections were incubated with 3% H2O2 solution for 30 min to inactivate the endogenous peroxidase then washed with PBS three times. Nonspecific binding sites were blocked with normal donkey serum diluted 1 : 10 in PBS for 60 min and incubated overnight at 4℃ with primary antiserum. After rinsing in PBS, sections were incubated in peroxidase-conjugated donkey anti-mouse IgG (Jackson ImmunoResearch Laboratories Inc., West Grove, PA, USA) for 1 h. For coloration, sections were incubated with a mixture of 0.05% 3,3-diaminobenzidine containing 0.01% H2O2 at room temperature until a brown color was visible, after which they were washed with PBS, counterstained with hematoxylin, and observed with light microscopy. The primary antisera were as follows: mouse monoclonal antibodies against ASMA at 1 : 2,000 (Sigma Chemicals), calponin at 1 : 500 (Dako Inc., Carpinteria, CA, USA), and vimentin at 1 : 2,000 (Biogenex, CA, USA).
The number of glomeruli was determined from each of the five differently stained serial sections. In each section of those stained with the PAS method, half of the cortical area was examined and the percentage of glomeruli with sclerosis was analyzed under 100X magnification. In the same area from serial sections stained with calponin-antiserum, glomeruli with calponin-positive periglomerular cells were counted.
The functional parameters of the animal groups are shown in Table 1. From 1 to 8 weeks after surgery, serum creatinine and BUN levels were significantly increased in both SNx and PAN groups compared with the sham group indicating deterioration of renal function. All experimental PAN groups exhibited significantly elevated urinary protein excretion compared to the sham group. SNx animals also demonstrated a progressive increase in urinary protein excretion which only became significant at 4 weeks after surgery.
After surgery, histological renal damage of the renal cortices progressed with time in both SNx and PAN rats. At 1 week there was little morphological change observed except proteinaceous casts in some tubuli. From 2 weeks after surgery, mild histopathological findings were detected, including tubular expansion, tubulointerstitial and periglomerular fibrosis, and mononuclear leukocyte infiltration. Sclerotic glomerular lesions were evident from 4 weeks and were more severe at 8 weeks after surgery in both SNx and PAN rats (Figs. 1 and 2). The kidneys of sham animals showed no distinct nephritic changes.
Table 2 shows the percentage of glomeruli with sclerosis in both experimental animal models. In sham animals no glomeruli with sclerotic changes were detected. At 1 and 2 weeks, only a very low percentage of glomeruli showed sclerotic changes (~1% in both SNx and PAN groups); thereafter, glomerulosclerosis progressed more rapidly in both SNx and PAN groups (24.74% and 59.41%, respectively) at 8 weeks post-surgery.
Table 3 shows the number of glomeruli with periglomerular calponin-positive cells. There were no periglomerular calponin-positive cells in the sham, PAN 1 and 2 week, and SNx 1, 2, and 4 week groups (data not shown), despite the presence of calponin-positivity in the renal vessels of the same groups (Fig. 3C). In the SNx 8 week and PAN 4 and 8 week groups, only a few glomeruli with periglomerular calponin-reactivity, which covered half or a very small part of periglomerular space, were observed. No cases of periglomerular calponin-positivity covering the entire periglomerular space were detected. As shown in Tables 2 and 3 for glomeruli in PAN rats at 8 weeks, approximately 59.4% glomeruli were sclerotic and approximately 11.7% (37/316) were calponin-positive, so around 19.7% (11.7%/59.4%) sclerotic glomeruli were calponin-positive.
The periglomerular calponin-positive cells were also both ASMA- and vimentin-positive, but not vice versa. All the glomeruli with periglomerular calponin-reactivity showed sclerotic changes, especially thickening of parietal epithelial cells (PECs) known as Bowman's capsule. PAMS staining showed that basement membranes of PECs covered by periglomerular calponin-positive cells were still intact. Weak calponin-immunostaining was detected in some glomeruli (Fig. 3).
The models of SNx and PAN in experimental animals have been widely used to investigate the progressive nature of chronic renal disease in humans (Grond et al., 1988; Ardiles et al., 2003). We used functional parameters and general histochemical results to confirm the reliability of the animal models. In the present study we tested the hypothesis that renal myofibroblasts in the periglomerular region may express calponin at the very end stage of chronic renal failure in the experimental animal kidney. Our previous study had shown that calponin-immunoreactivity was localized in the myofibroblasts of the periglomerular region of human kidney specimens obtained from recipients at the time of kidney transplantation (Choi et al., 2008 Abstract). Results from the present study are consistent with our previous study and imply that animals with chronic glomerulosclerotic injury may be useful models for examining the role of calponin in chronic renal damage.
Calponin was first purified from chicken gizzard smooth muscle as troponin-T like protein, named to reflect its ability to bind calmodulin, and to cross-react with anti-troponin T antibodies (Takahashi et al., 1986). Three isoforms of calponin (h1, h2, and acidic) have been identified in higher vertebrates as products of three homologous genes. H1-calponin is basic (pH 9.4), h2-calponin is near neutral (pH 7.5), whereas acidic calponin has a low pH of 5.2. H1-calponin is the predominant isoform specifically expressed in differentiated smooth muscle cells as well as other cell types including myofibroblasts in the context of diverse pathological situations (for review, Rozenblum & Gimona, 2008). The calponin antibody used in the present study is reported to detect h1-calponin (Yanagisawa et al., 2008).
Myofibroblasts express the mesenchymal marker ASMA and share features of both fibroblasts and smooth muscle cells. Known to be a major source of extracellular matrix proteins in fibrotic lesions in the kidney and other tissues, these cells are involved in wound healing and contraction as well as scarring (Högemann et al., 1993; Zhang et al., 1996; Tang et al., 1997; Badid et al., 2000). A prominent feature in the development of renal fibrosis and fibrotic lesions in general is the accumulation of myofibroblasts. The appearance of interstitial ASMA-positive myofibroblasts is the best prognostic indicator of disease progression in human and experimental glomerulonephritis (Alpers et al., 1994; Goumenos et al., 1994; Zhang et al., 1995; Essawy et al., 1997; Roberts et al., 1997; Badid et al., 1999).
Calponin is a specific marker for myofibroblasts in many tissues. To our knowledge, the present study is the first report of calponin expression in the myofibroblasts of animal kidneys. Our previous report detailed calponin expression in the myofibroblasts of human kidneys (Choi et al., 2008). In the present study, calponin was induced in some periglomerular myofibroblasts.
The function of calponin in the periglomerular myofibroblasts, especially around the sclerotic glomeruli, is obscure. The functional difference between calponin-positive and calponin-negative periglomerular myofibroblasts remains unknown and further investigation is required. There are some reports of calponin-positive and calponin-negative myofibroblasts; however, they only describe that some myofibroblasts within lesions were focally immunostained for calponin suggesting a functional heterogeneity of myofibroblasts (Lazard et al., 1993; Prasad et al., 1999; Barbareschi et al., 2001).
We observed a thickening of the PECs covered by calponin-positive myofibroblasts. PECs are known to play an active role in the progression of some renal diseases (Boucher et al., 1987; Goumenos et al., 1998; Asano et al., 2005). PECs also produce several bioactive substances in periglomerular and intraglomerular spaces (Cockwell et al., 1998; Cockwell et al., 1999; Nakamura 2005). Taken together with the active role of PECs in renal disease, we propose that interactions between PECs and calponin-positive myofibroblasts may play a key role in the late stage of glomerulosclerosis.
Figures and Tables
Fig. 1
Light microscopic findings of glomeruli representing glomeruloscrelosis lesions at each time point of disease rat. (A) SNx model sham, (B) SNx model 8 weeks, (C) PAN model sham, (D) PAN model 8 weeks. Note periglomerular and tubulointerstitial fibrosis in blue (B & D) with Masson's trichrome stain. Scale bar is 200 um.
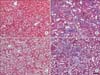
Fig. 2
Light microscopic findings of glomeruli representing glomeruloscrelosis lesions at each time point of disease rat. (A) SNx model sham, (B) SNx model 8 weeks, (C) PAN model sham, (D) PAN model 8 weeks. Glomeruli in B & D show segmental glomerulosclerotic change. Arrows indicate the infiltrated cells. Masson's trichrome stain. Scale bar is 100 um.
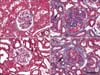
Fig. 3
Five serial sections (A to E, F to J) stained with periodic acid-methenamine silver method (A, F), alpha-smooth muscle actin (ASMA) antiserum (B, G), calponin antiserum (C, H), periodic acid-Schiff method (D, I) and vimentin antiserum (E, J) from the subtotal nephrectomy and puromycin model 8 weeks after operation. Almost basement membrane of Bowman's capsules (arrows in A, F) was intact. Calponinpositive cells (arrows in C, H) were also ASMA- and vimentin positive. Note the thickened membrane (arrows in D, I) covered by calponin-positive cells compared with the thin membrane of the glomerulus (G in H) without periglomerular calponin-positivity. Focal segemental glomerulosclerosis is shown (open arrowhead in D). Scale bar is 100 um.
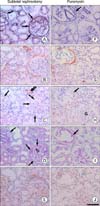
References
1. Alpers CE, Hudkins KL, Floege J, Johnson RJ. Human renal cortical interstitial cells with some features of smooth muscle cells participate in tubulointerstitial and crescentic glomerular injury. J Am Soc Nephrol. 1994. 5:201–209.
2. Ardiles LG, Figueroa CD, Mezzano SA. Renal kallikrein-kinin system damage and salt sensitivity: insights from experimental models. Kidney Int Suppl. 2003. 10. 86:S2–S8.
3. Asano T, Niimura F, Pastan I, Fogo AB, Ichikawa I, Matsusaka T. Permanent genetic tagging of podocytes: fate of injured podocytes in a mouse model of glomerular sclerosis. J Am Soc Nephrol. 2005. 16:2257–2262.
4. Badid C, Desmoulière A, McGregor B, et al. Interstitial alphasmooth muscle actin: A prognostic marker in membranous nephropathy. Clin Nephrol. 1999. 52:210–217.
5. Badid C, Mounier N, Costa AM, Desmoulière A. Role of myofibroblasts during normal tissue repair and excessive scarring: Interest of their assessment in nephropathies. Histol Histopathol. 2000. 15:269–280.
6. Barbareschi M, Pecciarini L, Cangi MG, et al. p63, a p53 homologue, is a selective nuclear marker of myoepithelial cells of the human breast. Am J Surg Pathol. 2001. 25:1054–1060.
7. Bohle A, Mackensen-Haen S, von Gise H. Significance of tubulointerstitial changes in the renal cortex for the excretory function and concentration ability of the kidney: a morphometric contribution. Am J Nephrol. 1987. 7:421–433.
8. Boucher A, Droz D, Adafer E, Noèl LH. Relationship between the integrity of Bowman's capsule and the composition of cellular crescents in human crescentic glomerulonephritis. Lab Invest. 1987. 56:526–533.
9. Chen W, Chu Y, Zhu D, et al. Perivascular gene transfer of dominant-negative N19RhoA attenuates neointimal formation via inhibition of TGF-beta1-Smad2 signaling in rats after carotid artery balloon injury. Biochem Biophys Res Commun. 2009. 389:217–223.
10. Choi JY, Lee SY, Jin DC, et al. Expression of Calponin in Immunoglobulin A Nephropathy: Proteomics and Immunohistochemical Study. 2008. In : Renal week; American Society of Nephrology;[Abstract].
11. Cockwell P, Brooks CJ, Adu D, Savage CO. Interleukin-8: a pathogenetic role in antineutrophil cytoplasmic autoantibodyassociated glomerulonephritis. Kidney Int. 1999. 55:852–863.
12. Cockwell P, Howie AJ, Adu D, Savage CO. In situ analysis of C-C chemokine mRNA in human glomerulonephritis. Kidney Int. 1998. 54:827–836.
13. Essawy M, Soylemzoglu O, Muchaneta-kubara EC, Shortland J, Brown CB, el Nahas AM. Myofibroblasts and the progression of diabetic nephropathy. Nephrol Dial Transplant. 1997. 12:43–50.
14. Ferguson HE, Kulkarni A, Lehmann GM, et al. Electrophilic peroxisome proliferator-activated receptor-γ ligands have potent antifibrotic effects in human lung fibroblasts. Am J Respir Cell Mol Biol. 2009. 41:722–730.
15. Frangogiannis NG, Michael LH, Entman ML. Myofibroblasts in reperfused myocardial infarcts express the embryonic form of smooth muscle myosin heavy chain (SMemb). Cardiovasc Res. 2000. 48:89–100.
16. Goumenos D, Brown CB, Shortland J, El Nahas AM. Myofibroblasts, predictors of progression of mesangial IgA nephropathy? Nephrol Dial Transplant. 1994. 9:1418–1425.
17. Goumenos DS, Tsomi K, Iatrou C, et al. Myofibroblasts and the progression of crescentic glomerulonephritis. Nephrol Dial Transplant. 1998. 13:1652–1661.
18. Grond J, Weening JJ, van Goor H, Elema JD. Application of puromycin aminonucleoside and adriamycin to induce chronic renal failure in the rat. Contrib Nephrol. 1988. 60:83–93.
19. Högemann B, Gillessen A, Böcker W, Rauterberg J, Domschke W. Myofibroblast-like cells produce mRNA for type I and III procollagens in chronic active hepatitis. Scand J Gastroenterol. 1993. 28:591–594.
20. Islam AH, Ehara T, Kato H, et al. Calponin h1 expression in renal tumor vessels: correlations with multiple pathological factors of renal cell carcinoma. J Urol. 2004a. 171:1319–1323.
21. Islam AH, Ehara T, Kato H, Hayama M, Nishizawa O. Loss of calponin h1 in renal angiomyolipoma correlates with aggressive clinical behavior. Urology. 2004b. 64:468–473.
22. Lazard D, Sastret X, Frid MG, Glukhova MA, Thiery JP, Koteliansky VE. Expression of smooth muscle-specific proteins in myoepithelium and stromal myofibroblasts of normal and malignant human breast tissue. Proc Natl Acad Sci U S A. 1993. 90:999–1003.
23. Nakamura H, Kitazawa K, Honda H, Sugisaki T. Roles of and correlation between alpha-smooth muscle actin, CD44, hyaluronic acid and osteopontin in crescent formation in human glomerulonephritis. Clin Nephrol. 2005. 64:401–411.
24. Prasad AP, Savera AT, Gown AM, Zarbo RJ. The myoepithelial immunophenotype in 135 benign and malignant salivary gland tumors other than pleomorphic adenoma. Arch Pathol Lab Med. 1999. 123:801–806.
25. Roberts IS, Burrows C, Shanks JH, Venning M, Mcwilliam LJ. Interstitial myofibroblasts: Predictors of progression in membranous nephropathy. J Clin Pathol. 1997. 50:123–127.
26. Rozenblum GT, Gimona M. Calponins: adaptable modular regulators of the actin cytoskeleton. Int J Biochem Cell Biol. 2008. 40:1990–1995.
27. Stahl PJ, Felsen D. Transforming growth factor-beta, basement membrane, and epithelial-mesenchymal transdifferentiation: implications for fibrosis in kidney disease. Am J Pathol. 2001. 159:1187–1192.
28. Takahashi K, Hiwada K, Kokubu T. Isolation and characterization of a 34000 Dalton calmodulin- and factin-binding protein from chicken gizzard smooth muscle. Biochem Biophys Res Commun. 1986. 141:20–26.
29. Tang WW, Van GY, Qi M. Myofibroblast and alpha1(III) collagen expression in experimental tubulointerstitial nephritis. Kidney Int. 1997. 51:926–931.
30. Yanagisawa Y, Takeoka M, Ehara T, Itano N, Miyagawa S, Taniguchi S. Reduction of Calponin h1 expression in human colon cancer blood vessels. Eur J Surg Oncol. 2008. 34:531–537.
31. Zhang G, Moorhead PJ, El Nahas AM. Myofibroblasts and the progression of experimental glomerulonephritis. Exp Nephrol. 1995. 3:308–318.
32. Zhang HY, Gharaee-Kermani M, Zhang K, Karmiol S, Phan SH. Lung fibroblast alpha-smooth muscle actin expression and contractile phenotype in bleomycin-induced pulmonary fibrosis. Am J Pathol. 1996. 148:527–537.