Abstract
Purpose
This study investigated the MRI, MR angiography (MRA) and MR perfusion findings of seizure-related cerebral cortical lesions during the periictal period.
Materials and Methods
From a retrospective review of the institutional database between 2011 and 2014, a total of 21 patients were included in this study. Two radiologists assessed periictal MRI, including MRA and MR perfusion, in patients with seizure-related cortical lesions. The parameters examined include: location of cortical abnormality, multiplicity of the affected cortical region, cerebral vascular dilatation, perfusion abnormality and other parenchymal lesions.
Results
All patients showed T2 hyperintense cerebral cortical lesions with accompanying diffusion restriction, either unilateral (18/21, 85.7%) or bilateral (3/21, 14.3%). Of the 21 patients enrolled, 10 (47.6%) had concurrent T2 hyperintense thalamic lesions, and 10 (47.6%) showed hippocampal involvement. Of the 17 patients (81%) who underwent MRA, 13 (76.5%) showed vascular dilatation with increased flow signal in the cerebral arteries of the affected cortical regions. On MR perfusion, all 5 patients showed cortical hyperperfusion, corresponding to the region of cortical abnormalities.
Seizures are relatively common, and about 10% the population experience a seizure during their lifetime (1). In addition, periictal neurologic deficits and neuroimaging findings of cerebral cortical T2 hyperintensity and diffusion restriction are well-known mimics of other central nervous system (CNS) disease, such as stroke. Although an exact explanation of these imaging abnormalities is still unclear, seizure-related changes on various imaging studies are believed to be a reflection of pathophysiologic alterations concerning seizure activity (23). According to the wide range of possible seizure-related lesions on neuroimaging studies, various disease entities are considered as a differential diagnosis, resulting in a potential risk of misdiagnosis and incorrect clinical management (4). Hence, there is a necessity to differentiate whether neuroimaging abnormalities in patients with seizure are caused by the seizure itself, or other underlying pathologic conditions such as stroke.
To the best of our knowledge, there are few studies regarding the seizure-related MR findings with combined use of conventional brain MRI, MR perfusion and MR angiography (MRA). The purpose of this study was to describe the MRI, MRA and MR perfusion findings of seizure-related cerebral cortical lesions during periictal period.
From the database of our institution between December 2011 and May 2014, we found 29 patients with seizure-related cerebral cortical lesions. Of these 29 patients, 21 met the following inclusion criteria: (a) had a history of seizure and (b) underwent MR examination during the ictal period or within 24 hours from the seizure onset. The 8 patients excluded from this study had taken the MR examination after 24 hours from the seizure onset. The final 21 patients who were included in this study included 18 males and 3 females (age range, 7 months - 87 years; mean age, 52.8 years). Our Institutional Review Board (IRB) approved this study, and informed consent was waived owing to the retrospective nature of the study.
MR imaging was performed using a 1.5-T system (Magnetom Avanto, Siemens Medical Solutions, Erlangen, Germany), according to the following sequences: diffusion-weighted image (DWI), T2-weighted image (T2WI), fluid-attenuated inversion recovery (FLAIR), T1-weighted image (T1WI), susceptibility-weighted image (SWI), and contrast-enhanced (CE) T1WI. Intracranial time of flight (TOF) MRA, CE cervical MRA, and dynamic susceptibility contrast (DSC) MR perfusion were also performed, but these images were not available for all patients. The parameters of the MR examinations were as follows: DWI (b values, 0 and 1000 s/mm2; repetition time (TR)/echo time (TE), 3200/91 msec; field of view (FOV), 22.0 × 22.0 cm; slice thickness, 5 mm; matrix, 192 × 192; number of slices, 20; and acquisition time, 1 minute 54 seconds); T2WI (TR/TE, 4300/98 msec; FOV, 17.5 × 22.0 cm; slice thickness, 5 mm; matrix, 384 × 214; number of slices, 20; and acquisition time, 2 minutes 35 seconds), FLAIR (TR/TE, 8000/114 msec; FOV, 17.5 × 22.0 cm; slice thickness, 5.0 mm; matrix, 256 × 143; number of slices, 20; and acquisition time, 2 minutes 42 seconds); T1WI (TR/TE, 414/11 msec; FOV, 17.6 × 22.0 cm; slice thickness, 5 mm; matrix, 320 × 154; number of slices, 20; and acquisition time, 1 minute 37 seconds); intracranial TOF MRA (TR/TE, 24/7 msec; FOV, 12.1 × 12.1 cm; slice thickness, 0.60 mm; matrix, 320 × 167; number of slices, 149; and acquisition time, 5 minutes 18 seconds); CE cervical MRA (TR/TE, 3.07/1.08 msec; FOV, 32.0 × 32.0 cm; slice thickness, 1.0 mm; matrix, 320 × 178; number of slices, 96; and acquisition time, 13 seconds); DSC MR perfusion (TR/TE, 1850/38 msec; FOV, 22.0 × 22.0 cm; slice thickness, 7 mm; matrix, 128 × 128; number of slices, 20; and acquisition time, 1 minute 40 seconds).
DSC MR perfusion study was performed using echo planar imaging (EPI) blood oxygen level dependent (BOLD) sequence. With a starting bolus injection of 0.1 mmol of gadobutrol (Gadovist; Schering, Berlin, Germany) per kilogram of body weight into the antecubital vein by using a power injector (Spectris; Medrad, Pittsburgh, PA, USA), dynamic scans of the whole brain were performed every 2 seconds. The analysis of MR perfusion data was post-processed with syngo Neuro (Siemens Medical Solutions, Erlangen, Germany), which is based on the “maximum slope model”. Parametric color-coded maps of the mean transit time (MTT), time-to-peak (TTP), relative cerebral blood flow (rCBF) and relative cerebral blood volume (rCBV) were obtained.
Two radiologists independently reviewed all MR examinations on PACS: the attending neuroradiologist (D.S.C.) had 25 years of experience in brain, head and neck imaging, and a 3rd year radiology resident (N.Y.K.). All reviewers were blinded to patient clinical data, except the current history of a seizure being the reason for examination. Images were evaluated, and following information was recorded: the presence and location of seizure-related cortical lesion and multiplicity of the affected cortical region on MRI, and ancillary MR findings such as cerebral vascular dilatation on MRA, perfusion abnormality, and other parenchymal lesions of potential seizure etiology. Multiplicity was defined as multilobular involvement of cortical lesions, regardless of bilaterality. The cerebral vascular dilatation was defined as an increase in the arterial diameter more than 0.5 mm compared to the contralateral side, or a progressive increase in the peripheral arterial diameter on intracranial TOF or cervical CE MRA. In cases of discrepancy, both reviewers later reached a consensus.
The clinical and radiological data of study patients are summarized in Table 1. Of the 21 patients, 9 patients (42.9%) showed neurologic symptoms which mimic acute stroke, namely: motor weakness (5/21, 23.8%), aphasia (2/21, 9.5%), and combined motor weakness and dysarthria (2/21, 9.5%). Other symptoms were fever (2/21, 9.5%) and decreased mentality (1/21, 4.7%); however, 9 (42.9%) had no accompanying symptom.
On conventional MRI, 13/21 patients (61.9%) had structural abnormalities which signified potential seizure etiology: tissue loss by various pathologies such as prior infarction, trauma or surgery (10/13, 69.2%), subdural hemorrhage (2/13, 15.3%), and left hippocampal sclerosis (1/13, 7.7%). Other potential etiologies for seizure included chronic alcohol intake, febrile convulsion, and transient metabolic disorders such as hyponatremia and hypoglycemia. However, 1 patient showed no potential seizure etiology.
MR examinations were obtained within 24 hours from the seizure onset, with average of 11 hours 21 minutes for the time from the seizure onset to MRI (range, 1 hour 7 minutes to 23 hours 54 minutes). All 21 patients showed seizure-related cortical abnormalities on periictal MRI, which were characterized as increased signal intensity with swelling of involved cortical gyri on T2 and FLAIR images in the unilateral cerebral hemisphere (18/21, 85.7%) (Figs. 1, 2), and bilateral cerebral hemispheres (3/18, 14.3%). DWI at the same location also revealed increased signal intensity with a reduction of apparent diffusion coefficient (ADC) value (Figs. 1, 2, 3). No single lobar cortical lesion was observed. In addition, 10 patients (47.6%) had hyperintense thalamic lesions in the pulvinar nuclei: ipsilateral (9/21, 42.8%) (Figs. 1, 2, 3) and bilateral (1/21, 7.8%). Mesial temporal structures were involved in 10 patients (47.6%) with unilateral predominance (Fig. 3). The multiplicity of cortical lesions and concurrent parenchymal lesions are listed in Table 2.
Of the 17 patients who underwent MRA, 13 (76.5%) showed cerebral vasodilatation and increased flow signal of the cerebral arteries in the regions corresponding to cortical abnormalities (Fig. 1). Of these 13 patients, 12 (92.3%) showed vasodilatation in the ipsilateral cerebral hemisphere of the cortical lesions, whereas one patient (7.7%) with bilateral cortical region revealed cerebral arterial dilatation in the dominant hemisphere of the affected cortical lesions.
Although only 5 patients underwent MR perfusion in this study, all of them showed obvious spatial hyperperfusion on CBF, MTT and TTP maps in the areas corresponding to cortical and thalamic abnormalities on conventional MRI (Fig. 3).
It is estimated that between 5 to 30% of cases identified as “brain attacks” are, in fact, due to stroke-mimicking conditions (56). Of these, seizure-related neurologic deficits with associated neuroimaging findings are well-known as great mimics of ischemic stroke. However, the exact pathophysiologic explanation of seizure-related changes has not yet been established (6). Many possible mechanisms account for the periictal state, including neurotransmitter depletion, neuronal desensitization, alteration of local CBF, and various kinds of active inhibition, for which the utility of multiple imaging modalities have been investigated in previous studies (78910). To our best knowledge, there are few studies regarding seizure-related brain changes with combined use of conventional MRI, MR perfusion and MRA. Particularly remarkable was our finding the presence of cortical lesions on T2, FLAIR and DWI, with ancillary hemodynamic changes in the affected cortical regions.
On brain MRI, the cerebral cortical T2 hyperintensity with diffusion restriction is commonly observed in patients with seizure, and these findings were usually accompanied by focal parenchymal swelling and increased vascularity (41112). The seizure-induced cellular disturbance occurs due to various phenomena, such as vasogenic and cytotoxic edema, increased permeability, loss of autoregulation, regional ischemia, and excitotoxicity (1314). These changes usually result in an increase of T2 and FLAIR signal intensity, and variable alterations on DWI and ADC (1516). Consistent with previous studies, we found that seizure-related cortical abnormalities in all 21 patients showed T2 and FLAIR hyperintensity with diffusion restriction. However, the exact explanation determining whether and when seizure-associated cellular changes become detectable cortical lesions on brain MRI, is not yet clearly understood. The seizure-related cortical abnormalities usually return to normal after the seizure is controlled, but they can be irreversible in case of severe or prolonged seizures (1217) (Fig. 1). In this study, only 3 patients underwent a follow-up MRI within 1 month: 1 patient revealed complete resolution of the preexisting cortical abnormalities, and the other 2 patients showed a decrease in the affected cortical regions (Fig. 3). Although the development of permanent structural abnormalities after seizures has been investigated, especially in the context of the long-lasting controversy, seizure-related hypoxia may be responsible for the evolution of the cortical lesions (18). In addition, our study revealed multilobular distribution in these cortical lesions. We thought that it might be related to the spread of a residual or ongoing ictal discharge, and further studies are required for proving this finding.
In our study, 9 patients had stroke-like symptoms such as motor weakness, dysarthria or aphasia. It is diagnostically challenging when cortical diffusion restriction is seen in patients with seizure. Since seizures can also occur in patients with acute stroke, it is often difficult to differentiate seizure-related changes from acute stroke in clinical practice. In these clinical situations, MR perfusion can serve as a practical tool to differentiate between these two pathologies, since the perfusion status may be opposing: hypoperfusion in case of infarction, versus hyperperfusion in case of seizure-related change (17192021). In the present study, all 5 patients who underwent perfusion MR imaging showed hyperperfusion in the corresponding area of seizure-related cortical lesions (Fig. 3). Seizure causes excessive neuronal activity in the early stage, and concomitant perfusion increases as a compensatory mechanism to the increased metabolic demand (222324). Therefore, the duration of ictal activity may be the critical factor responsible for the changes detected on MR perfusion (1720).
Our studies reveal that 10 of 21 patients had concurrent parenchymal abnormalities in the pulvinar nuclei of the thalami (Figs. 1, 2, 3). In previous studies, investigators have suggested that thalamic nuclei are involved in various epilepsy syndromes. Coexisting thalamic abnormalities in seizure patients are likely the result of excessive activity in the thalamic nuclei having reciprocal connections with the affected cerebral cortices (252627). Therefore, the thalamus may be related to the evolution and propagation of ictal discharge (27). In agreement with previous results (228), we observed hippocampal involvement in 10 patients (Fig. 3). As is well-documented, the hippocampus is one of common locations of seizure-induced brain abnormalities on the periictal MRI. This finding suggests that the mesial temporal structures are selectively vulnerable regions to excitotoxic brain injury initiated by seizure (228).
We also observed vascular changes on MRA in 13 patients. Of these, 12 patients showed cerebral vascular dilatation in the ipsilateral hemisphere of the cortical lesions (Fig. 1). The remaining patient with bilateral cortical lesions, demonstrated cerebral vascular dilatation in the dominantly affected hemisphere. Vasodilatation with increased flow signal of the cerebral arteries on MRA presumably reflects increased CBF to the active cortices by ictal discharge (121929). Although the exact mechanism of cerebral vascular dilatation associated with hyperperfusion has not yet to be completely defined, seizure-related lesions are differentiated from acute stoke on the basis of the presence or absence of cerebral vascular dilatation.
There are several limitations of this study. First, due to the retrospective nature of the study, there was an unavoidable selection bias. Second, a relatively small number of patients was included in this study. Therefore, our study had a weakness for generalization. Third, we did not investigate clinical and radiological follow-up data for assessing the long-term effect of the preexisting seizure-related lesions. Finally, MR perfusion and MRA could not be carried out in all patients. This heterogeneous MR protocol may have prevented us from comprehensively evaluating the radiologic data in the enrolled patients. Therefore, further studies with large sample size and homogenous MR protocol are required for the validation of our results.
In conclusion, our results demonstrate that seizure-related cerebral cortical lesions are characterized by T2 hyperintensity and diffusion restriction. We also observed ancillary periictal MR findings of regional cortical hyperperfusion and cerebral vascular dilatation, corresponding to the cortical abnormalities. In addition, these findings suggest ongoing or residual ictal discharge with high probability. Therefore, we propose that an awareness of the clinical and radiological findings can be helpful for differentiating seizure-related cortical lesions from other CNS diseases such as acute stroke, and reducing the risk of inappropriate management in clinical practice.
Figures and Tables
Fig. 1
A 42-year-old male patient with seizure and dysarthria (patient 13). Axial FLAIR (a) and DWI (b) show cortical hyperintensities in the right cerebral cortices (short arrows) and ipsilateral thalamus (long arrows). On ADC map image (c), the lesions (except for the insula) reveal diffusion restriction (arrows). MR angiography (d) reveals marked dilatation with increased flow signal of the right middle cerebral artery (arrows). On follow-up FLAIR (e) and DWI (f) obtained 8 months later, there is diffuse atrophy of the right cerebral hemisphere.
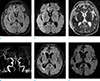
Fig. 2
A 58-year-old male patient with seizure (patient 18). Axial FLAIR (a, b) and DWI (c, d) show multiple cortical hyperintensities in the right cerebral cortices (short arrows) and ipsilateral thalamus (long arrows). ADC map images (e, f) reveal restricted water diffusion of the lesions (arrows).
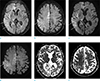
Fig. 3
A 75-year-old female patient with aphasia (aphasic status epilepticus) (patient 21). There are hyperintense lesions in the left temporooccipital lobes, hippocampus and thalamus (arrows) on DWI (a, b). On ACD map images (c, d), the lesions show restricted diffusion (arrows). Perfusion MR images (e, f) depict increased cerebral blood flow (e) and shortened time to peak (f) in the corresponding areas (arrows). On follow-up DWI (g, h) obtained 2 days later, the extent of lesions has decreased (arrows).
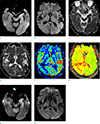
Table 1
Clinicoradiologic Characteristics of Patient Population
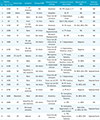
ACA = anterior cerebral artery; BG = basal ganglia; CP = complex partial; F = frontal; GT = generalized tonic; ICH = intracerebral hematoma; Lt. = left; MCA = middle cerebral artery; MRA = MR angiography; NA = nonapplicable; O = occipital; P = parietal; PCA = posterior cerebral artery; Rt. = right; SDH = subdural hematoma; SP = simple partial; T = temporal; TC = tonic-clonic
Table 2
Multiplicity of the Cortical Lesions with Concurrent Parenchymal Lesions
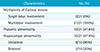
References
1. Annegers JF. The epidemiology of epilepsy. In : Wyllie E, editor. The treatment of epilepsy: principles and practice. 3rd ed. Philadelphia: Lippincott Williams & Wilkins;2001. p. 131–138.
2. Cole AJ. Status epilepticus and periictal imaging. Epilepsia. 2004; 45:Suppl 4. 72–77.
3. Briellmann RS, Wellard RM, Jackson GD. Seizure-associated abnormalities in epilepsy: evidence from MR imaging. Epilepsia. 2005; 46:760–766.
4. Cianfoni A, Caulo M, Cerase A, et al. Seizure-induced brain lesions: a wide spectrum of variably reversible MRI abnormalities. Eur J Radiol. 2013; 82:1964–1972.
5. Davis DP, Robertson T, Imbesi SG. Diffusion-weighted magnetic resonance imaging versus computed tomography in the diagnosis of acute ischemic stroke. J Emerg Med. 2006; 31:269–277.
6. Mathews MS, Smith WS, Wintermark M, Dillon WP, Binder DK. Local cortical hypoperfusion imaged with CT perfusion during postictal Todd's paresis. Neuroradiology. 2008; 50:397–401.
7. Weinand ME, Carter LP, el-Saadany WF, Sioutos PJ, Labiner DM, Oommen KJ. Cerebral blood flow and temporal lobe epileptogenicity. J Neurosurg. 1997; 86:226–232.
8. Moritani T, Smoker WR, Sato Y, Numaguchi Y, Westesson PL. Diffusion-weighted imaging of acute excitotoxic brain injury. AJNR Am J Neuroradiol. 2005; 26:216–228.
9. Warach S, Levin JM, Schomer DL, Holman BL, Edelman RR. Hyperperfusion of ictal seizure focus demonstrated by MR perfusion imaging. AJNR Am J Neuroradiol. 1994; 15:965–968.
10. Chan S, Chin SS, Kartha K, et al. Reversible signal abnormalities in the hippocampus and neocortex after prolonged seizures. AJNR Am J Neuroradiol. 1996; 17:1725–1731.
11. Goyal MK, Sinha S, Ravishankar S, Shivshankar JJ. Peri-ictal signal changes in seven patients with status epilepticus: interesting MRI observations. Neuroradiology. 2009; 51:151–161.
12. Huang YC, Weng HH, Tsai YT, et al. Periictal magnetic resonance imaging in status epilepticus. Epilepsy Res. 2009; 86:72–81.
13. Holmes GL. Seizure-induced neuronal injury: animal data. Neurology. 2002; 59:S3–S6.
14. Wang Y, Majors A, Najm I, et al. Postictal alteration of sodium content and apparent diffusion coefficient in epileptic rat brain induced by kainic acid. Epilepsia. 1996; 37:1000–1006.
15. Kim JA, Chung JI, Yoon PH, et al. Transient MR signal changes in patients with generalized tonicoclonic seizure or status epilepticus: periictal diffusion-weighted imaging. AJNR Am J Neuroradiol. 2001; 22:1149–1160.
16. Bouilleret V, Nehlig A, Marescaux C, Namer IJ. Magnetic resonance imaging follow-up of progressive hippocampal changes in a mouse model of mesial temporal lobe epilepsy. Epilepsia. 2000; 41:642–650.
17. Szabo K, Poepel A, Pohlmann-Eden B, et al. Diffusion-weighted and perfusion MRI demonstrates parenchymal changes in complex partial status epilepticus. Brain. 2005; 128:1369–1376.
18. Nevander G, Ingvar M, Auer R, Siesjo BK. Status epilepticus in well-oxygenated rats causes neuronal necrosis. Ann Neurol. 1985; 18:281–290.
19. Toledo M, Munuera J, Sueiras M, Rovira R, Alvarez-Sabin J, Rovira A. MRI findings in aphasic status epilepticus. Epilepsia. 2008; 49:1465–1469.
20. Calistri V, Caramia F, Bianco F, Fattapposta F, Pauri F, Bozzao L. Visualization of evolving status epilepticus with diffusion and perfusion MR imaging. AJNR Am J Neuroradiol. 2003; 24:671–673.
21. Hayward NM, Ndode-Ekane XE, Kutchiashvili N, Grohn O, Pitkanen A. Elevated cerebral blood flow and vascular density in the amygdala after status epilepticus in rats. Neurosci Lett. 2010; 484:39–42.
22. Unrath A, Muller HP, Ludolph AC, Kassubek J. Reversible cortical diffusion restriction, hyperperfusion and T2-hyperintensity caused by two different types of epileptic seizure. Clin Neuroradiol. 2012; 22:239–243.
23. Zhao M, Suh M, Ma H, Perry C, Geneslaw A, Schwartz TH. Focal increases in perfusion and decreases in hemoglobin oxygenation precede seizure onset in spontaneous human epilepsy. Epilepsia. 2007; 48:2059–2067.
24. Wasterlain CG, Fujikawa DG, Penix L, Sankar R. Pathophysiological mechanisms of brain damage from status epilepticus. Epilepsia. 1993; 34:Suppl 1. S37–S53.
25. Nagasaka T, Shindo K, Hiraide M, Sugimoto T, Shiozawa Z. Ipsilateral thalamic MRI abnormality in an epilepsy patient. Neurology. 2002; 58:641–644.
26. Shipp S. The functional logic of cortico-pulvinar connections. Philos Trans R Soc Lond B Biol Sci. 2003; 358:1605–1624.
27. Katramados AM, Burdette D, Patel SC, Schultz LR, Gaddam S, Mitsias PD. Periictal diffusion abnormalities of the thalamus in partial status epilepticus. Epilepsia. 2009; 50:265–275.
28. Eid T, Ghosh A, Wang Y, et al. Recurrent seizures and brain pathology after inhibition of glutamine synthetase in the hippocampus in rats. Brain. 2008; 131:2061–2070.
29. Tuan Huynh NN, Hiroyoshi A, Shozo N, Takashi T, Hideo T, Akira M. Reversible focal radiological changes due to non-convulsive status epilepticus of the right parietooccipital lobe. Turk Neurosurg. 2013; 23:278–281.