Abstract
Purpose
Materials and Methods
Results
Figures and Tables
Fig. 1
MR images of a 59-year-old man with hepatocellular carcinoma. He had one hepatocellular carcinoma in liver segment 6. A hepatocellular carcinoma (arrow) in the liver is clearly shown on the axial breath-hold, T1-weighted 3D GRE image with CAIPIRINHA (a) in this cooperative patient. The intrahepatic vessel (arrowhead) is well-visualized in the T1-weighted 3D GRE image with CAIPIRINHA (b). Axial free-breathing 3D radial GRE with GA (c, d) and 3D radial GRE with ILAB (e, f) show blurred resolution of the tumor (arrows) and margin of the intrahepatic vessel (arrowheads).
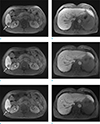
Fig. 2
MR images in a 75-year-old woman with intrahepatic, mass-forming cholangiocarcinoma. She had one intrahepatic, mass-forming cholangiocarcinoma in liver segments 4 and 8. Axial breath-hold, T1-weighted 3D GRE image with CAIPIRINHA (a) shows severe motion artifacts due to the patient's breathing. Axial free-breathing, 3D radial GRE with GA (b) and 3D radial GRE with ILAB (c) demonstrate substantially artifact reduction resistance to motion and high image quality in the patient who could not suspend respiration. The intrahepatic, mass-forming cholangiocarcinoma (arrows) and intrahepatic vessels (arrowheads) are better depicted in axial free-breathing, 3D radial GRE with GA and 3D radial GRE with ILAB.
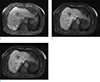
Table 1
Standard and Motion-Resistant Protocols at a 3.0T MR Scanner
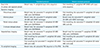
CAIPIRINHA = controlled aliasing in parallel imaging results in a higher acceleration; GA = golden angle; GRE = gradient-recalled echo; ILAB = interleaved angle-bisection; KWIC = k-space weighted image contrast technique; MP-RAGE = magnetization prepared, rapid-acquisition gradient echo; SS-ETSE = single-shot, echo-train, spin-echo; TSE = turbo spin echo; VIBE = volumetric interpolated breath-hold examination
Table 2
Parameters of Motion-Resistant Sequences Used at a 3.0T MR Scanner
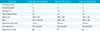
BH = breath-hold; BI = breathing-independent; CAIPIRINHA = controlled aliasing in parallel imaging results in a higher acceleration; FOV = field of view; GA = golden angle; GRE = gradient-recalled echo; ILAB = interleaved angle-bisection; TE = echo time; TR = repetition time; VIBE = volumetric interpolated breath-hold examination
Table 3
Results of Qualitative Analysis of Three, Motion-Resistant Sequences in Cooperative Patients
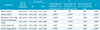
Values are the mean ± standard deviation. NA: Wilcoxon signed rank test was not available.
Wilcoxon signed rank test with Bonferroni correction was used for comparison between pairs of sequences. Pa Results were considered statistically significant at Pa < 0.016.
Friedman nonparametric test was used for statistical analysis of the three sequences. Pb Results were considered statistically significant at Pb < 0.05.
*Statistically significant
CAIPIRINHA = controlled aliasing in parallel imaging results in a higher acceleration; GA = golden angle; GRE = gradient-recalled echo; ILAB = interleaved angle-bisection; VIBE = volumetric interpolated breath-hold examination
Table 4
Results of Qualitative Analysis of Three, Motion-Resistant Sequences in Uncooperative Patients
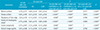
Values are the mean ± standard deviation. NA: Wilcoxon signed rank test was not available.
Wilcoxon signed rank test with Bonferroni correction was used for comparison between pairs of sequences. Pa Results were considered statistically significant at Pa < 0.016.
Friedman nonparametric test was used for statistical analysis of the three sequences. Pb Results were considered statistically significant at Pb < 0.05.
*Statistically significant
CAIPIRINHA = controlled aliasing in parallel imaging results in a higher acceleration; GA = golden angle; GRE = gradient-recalled echo; ILAB = interleaved angle-bisection; VIBE = volumetric interpolated breath-hold examination
Table 5
Results of Quantitative Analysis of Three, Motion-Resistant Sequences in Cooperative Patients
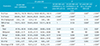
Values are the mean ± standard deviation. NA: Post hoc paired t-test with Bonferroni correction was not available.
Post hoc paired t-test with Bonferroni correction was used for comparison between pairs of sequences. Pa Results were considered statistically significant at Pa < 0.016.
Repeated measures analysis of variance was used for statistical analysis of the three sequences. Pb Results were considered statistically significant at Pb < 0.05.
* Statistically significant
CAIPIRINHA = controlled aliasing in parallel imaging results in a higher acceleration; CNR = contrast-to-noise ratio; GA = golden angle; GRE = gradient-recalled echo; ILAB = interleaved angle-bisection; SD = standard deviation; SI = signal intensity; SNR = signal-to-noise ratio; VIBE = volumetric interpolated breath-hold examination
Table 6
Results of Quantitative Analysis of Three, Motion-Resistant Sequences in Uncooperative Patients
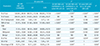
Values are the mean ± standard deviation. NA: Post hoc paired t-test with Bonferroni correction was not available.
Post hoc paired t-test with Bonferroni correction was used for comparison between pairs of sequences. Pa Results were considered statistically significant at Pa < 0.016.
Repeated measures analysis of variance was used for statistical analysis of the three sequences. Pb Results were considered statistically significant at Pb < 0.05.
*Statistically significant
CAIPIRINHA = controlled aliasing in parallel imaging results in a higher acceleration; CNR = contrast-to-noise ratio; GA = golden angle; GRE = gradient-recalled echo; ILAB = interleaved angle-bisection; SD = standard deviation; SI = signal intensity; SNR = signal-to-noise ratio; VIBE = volumetric interpolated breath-hold examination