Abstract
Purpose
To evaluate the knee joint after double-bundle anterior cruciate ligament (ACL) reconstruction with three-dimensional (3D) isotropic magnetic resonance (MR) image, and to directly compare the ACL graft findings on 3D MR with the clinical results.
Materials and Methods
From January 2009 to December 2014, we retrospectively reviewed MRIs of 39 patients who had reconstructed ACL with double bundle technique. The subjects were examined using 3D isotropic proton-density sequence and routine two-dimensional (2D) sequence on 3.0T scanner. The MR images were qualitatively evaluated for the intraarticular curvature, graft tear, bony impingement, intraosseous tunnel cyst, and synovitis of anteromedial and posterolateral bundles (AMB, PLB). In addition anterior tibial translation, PCL angle, PCL ratio were quantitatively measured. KT arthrometric values were reviewed for anterior tibial translation as positive or negative. The second look arthroscopy results including tear and laxity were reviewed.
Results
Significant correlations were found between an AMB tear on 3D-isotropic proton density MR images and arthroscopic proven AMB tear or laxity (P < 0.05). Also, a significant correlation was observed between increased PCL ratio on 3D isotropic MRI and the arthroscopic findings such as tear, laxities of grafts (P < 0.05). KT arthrometric results were found to be significantly correlated with AMB tears (P < 0.05) and tibial tunnel cysts (P < 0.05).
Anterior cruciate ligament (ACL) reconstruction is one of the most common surgical procedures conducted in sports medicine and can be performed using a variety of techniques (1).
Traditional ACL reconstruction is performed using the single anteromedial bundle technique and has a high clinical success rate (23). However, studies show that 15-25% of patients continue to experience pain and instability. Single bundle (SB) reconstructions sufficiently restore anteroposterior tibial translation but are also associated with increased tibial rotation, and thus, do not fully restore native kinetics of the knee joint (23). Double-bundle (DB) reconstruction has several advantages over SB reconstruction with respect to regaining a structure that closely resembles the normal ACL, because it provides wider contact areas between bone and grafted tendon than single bundle reconstruction. Furthermore, DB ACL reconstruction allows anteromedial and posterolateral bundles to be created anatomically and independently, which leads to better stability (4).
MRI evaluations of traditional single bundle ACL graft reconstruction and of its complications are well documented, but comparatively few reports have been issued on MRI evaluations of DB reconstruction. Furthermore, because DB reconstruction is becoming increasingly popular, it is important to become familiar with the normal postoperative appearance of these grafts and are able to differentiate between normal healing and common graft complications.
The complex anatomy of ACL grafts after double bundle reconstruction makes it difficult to visualize the entire course of these grafts on MR (magnetic resonance) images. Furthermore, the surgical complexity of DB reconstruction, as compared with SB reconstruction, can lead to different postoperative responses. For example, DB ACL reconstruction may be associated with less graft-to-tunnel healing in the posterolateral bundle, as compared with SB reconstruction, which might be due to a higher excursion pattern in double bundle grafts (5).
To understand the knee as a whole, it is essential that the graft and the relationship between it and the bone tunnel be considered. MRI is useful for assessing normal, injured, and reconstructed ACLs (67), but the interpretations of postoperative MR images is made difficult by multiple tunnels, fixation hardware, and the many graft materials used during DB reconstruction (8).
Conventional two dimensional (2D) images are limited in terms of evaluating the ACL or tunnel postoperatively. Unless scan planes are added, such as, coronal oblique or sagittal views, the courses and integrities of DB ACL are not fully visible. Previous studies have shown that oblique scans provide better evaluations of ACL integrity after DB reconstruction (9).
If 3D isotropic MRI is used, images can be reconstructed to fit the two graft bundles, four intraosseous tunnels in the entire course individually after one sequence. Furthermore, this technique can also be used to evaluate intraarticular conditions, such as, synovial hypertrophy. Hence, we undertook to analyze 3D MR images of the knee after DB ACL reconstruction, and to compare the ACL graft findings on 3D MR with the clinical and kinetic study results.
This retrospective study was approved by the Institutional Review Board at Gachon Gil Medical Center.
Seventy-three patients that underwent knee MRI after DB reconstruction from January 2009 to December 2014 at our institute were initially considered. 32 Patients who did not undergo second look arthroscopy and 2 patients without 3D isotropic MRI were excluded. As a result, the study cohort was composed of 39 patients (5 women [mean age 31 years, range 21-45 years] and 34 men [mean age 29 years, range 16-64 years], 37 complete tears and 2 partial tears preoperatively). Three orthopedic surgeons performed the ACL reconstructions. Mean time from operation to MR imaging was 23 months (range, 7-81).
The 39 study subjects were examined in the routine extended position with a dedicated knee coil using 3.0T MRI (Skyra and Verio, Siemens, Erlangen, Germany). 3D isotropic (Sampling Perfection with Application optimized Contrasts using different flip angle Evolution) images were obtained through the whole knee (slice thickness, 0.5 mm; TR/TE 1300/38; field of view [FOV], 150 x 150 mm; matrix, 320 x 276; average imaging time 4 min 40 s), in addition to standard sequences (coronal proton density, sagittal T1-weighted [T1W] and fat-saturated T2-weighted [T2W] images, axial fat-saturated T2W images, with slice thickness/gap 4/0.8 mm, FOV 110 x 160, matrix 448 x 364, average imaging time 2 min per sequence. TR (repetition time)/TE (echo time) for the T2WI ranged 3000-5000 msec and 30-80 msec, respectively. The 3D isotropic images were reformatted by multiplanar reconstruction until the best visualization of the each bundles was achieved, using Aquarius Thin Client software (TeraRecon Inc., Foster City, CA, USA). The images were interpreted by two radiologists unaware of clinical data; one was a musculoskeletal radiologist with 15 years and another was a radiologist with 3 years of experience. MRI findings were evaluated by consensus.
Radiological evaluations were classified according to primary and secondary variables. Primary variables indicated the direct MRI findings of the graft, and included arthroscopic curvature, graft tear and focal impingement. The secondary variables were adjacent synovitis and cystic lesions in each tunnels, because they were considered to be likely to provide additional information regarding articular conditions (10). Tunnel cyst were reviewed on combined 3D isotropic sequence and routine T1WI, T2WI. Other variables were reviewed on 3D isotropic sequence.
Subjective scoring of these variables was performed by radiologists using a 2 point scale (Table 1).
Intraarticular graft curvatures were classified according to degree of angulation; straight curvature as 0 points, and angulation 1 points. (Fig. 1a-c). Graft tear were classified as follows; none as 0, marginal fraying or fluid signal disrupting graft fibers as 1 (Fig. 2a, b).
Graft impingement was defined as a non-anatomical tunnel location whereby the graft rubbed against the roof of the femoral intercondylar notch resulting in extension loss. MRI findings of impingement are; posterior bowing of the graft, graft contact with the notch roof, and increased SI in T2W and PD images in the anterior graft region (6) (Fig. 3).
Synovitis was defined linear or nodular intermediate to low SI lesion within the knee joint near the AMB and PLB.
Cystic lesion of the graft tunnel was defined as high signal intensity lesion at the graft-bone interfaces of femoral or tibial tunnels of the anteromedial and posterolateral bundles (AMB, PLB) (Fig. 4), assisted by the fluid signal intensity findings on T1WI and fat-saturated T2WI.
Measurements were obtained in the midsagittal plane of the lateral compartment. Two lines were drawn perpendicular to the tibial plateau. One of these lines was placed along the most posterior cortical margin of the lateral femoral condyle, and the other along the most posterior aspect of the lateral tibial plateau. The perpendicular distance between these lines was measured (Fig. 5). Values of at least 5 mm were considered abnormal as in the study by Vahey et al. (11).
The Index was consisted of the ratio B/A, with B equaling the length of the line between the posterior inferior tibial and the superior anterior femoral attachment of the PCL, and A equaling the maximum perpendicular distance from this line to the PCL (13). PCL ratio was considered an objective and reproducible index measurement of PCL shape for normal and injured ACL in the study by Liu et al. (13). Buckled PCL would lead to a longer A relative to the B.
All patients underwent arthrometric evaluations of reconstructed and contralateral uninjured knees (KT-1000 arthrometer; Med-Metric, San Diego, CA, USA) (14). These evaluations were performed by one examiner unaware to MRI findings. Patients were positioned supine with the knee flexed to a mean of 300. A preconditioning force was secured to the anterior tibia with the tibial sensor pad in contact with the tuberosity and applied three times to determine the zero point. Results quoted are the means of three measurements.
Qualitative evaluations of reconstructed ACL grafts on 3D-isotropic MR images are summarized in Table 1.
Of the 39 study subjects, arced or angulated ACL graft was observed 12 (31%) AMBs and in 12 (31%) PLBs. An ACL graft tear was present in 6 AMBs (15%) and 10 PLBs (26%). Focal bony impingement was evident in 15 (38%) and 8 (21%), respectively, and synovitis around the bundle in 15 (38%) and 7 (18%), respectively. Cystic tunnel lesions were noted in 15 (38%) and 17 (44%) of tibial AMBs and PLBs, and in 7 (18%) and 17 (44%) femoral AMBs and PLBs, respectively (Table 1).
The quantitative evaluations of reconstructed ACL grafts on 3D-isotropic MR images are presented in Table 2. Using the 5 mm cutoff for anterior translation of the tibia by MRI, 18 patients (46%) had increased anterior translation, and using the 107° PCL angle cutoff, 21 patients (54%) had decreased PCL angle.
Second look arthroscopy scores are summarized in Table 3. An ACL graft tear was observed in 3/39 (1%) of AMBs and in 7/39 (18%) of PLBs. ACL grafts laxity was present in 4/39 (10%) AMBs and in 7/39 (18%) PLBs. The average sagittal laxity side to side difference by the KT arthrometer ranged from 0 to 8.
Significant correlations were found between an AMB tear on 3D-isotropic MR images and a perioperatively proven AMB tear (P < 0.05) or AMB laxity (P < 0.05) (Table 4).
The average ATT on MRI of patients with arthroscopy-proven tear and laxity of AMB was 4.8 and 5.1 vs. 4.7 and 4.7 without the tear/laxity, respectively. The average ATT with arthroscopy-proven PLB tear/laxity was 3.8/3.8 vs. 4.9/4.9 without the positive arthroscopy finding, respectively. No significant correlation was observed between the ATT and the operative findings.
The average PCL ratios of patients with arthroscopy-proven tear and laxity of AMB was 0.37 and 0.36, respectively, compared with 0.29 and 0.29 without the tear or laxity (P < 0.05). There was no statistically significant association between the PLB tear or laxity with PCL ratio.
No significant correlation was found between graft SI, curvature, impingement, synovitis, or PCL angle on 3D isotropic MR images with the graft tear or laxity.
The average sagittal laxity side to side difference by the KT arthrometer ranged from 0 to 8, and the correlative results are shown in table 5. Statistically significant correlation was found in MRI-visualized AMB tears and in the PLB tibial tunnel cysts with the arthrometric results (P < 0.05). Other imaging findings did not correlate with the KT arthrometric results. Comparing the KT arthrometric results with the arthroscopic findings, only the AMB tear showed significant association (P < 0.05).
MRI is the most frequently used diagnostic imaging procedure for suspected injuries of the ACL and several other evaluations related to ACL reconstruction, and is the most important postoperative procedure next to physical examination.
A small number of studies have been conducted to determine the effect of additional knee MRI views for grading ACL graft injury for DB and selective-bundle ACL reconstructions (9). After the double-bundle or selective-bundle ACL reconstruction, oblique sagittal and coronal images of the ACL provide better anatomic evaluation of the ACL than orthogonal views. The specificity and accuracy of diagnoses were improved when oblique views were combined. But, the previous studies did not explore the direct relationship between MR and clinical findings. Furthermore, the obliqueness of the 2D scans is planned by the technicians on the MR scanner; after the acquisition, it is difficult for the radiologists to look from various angles. In the present study, we could visualize each bundle separately by the 3D-isotropic images, and found a significant correlation between an AMB graft tear and a tear or laxity at surgery. The AMB is important in terms of resisting anterior laxity, whereas the PLB contributes to rotational stability (15). Hence, it is important to keep track of the AMB on postoperative MR images of the knee. Given the complex orientation of the two grafts, which do not run parallel to each other, ideally a single MRI sequence should be used that allows high resolution multiplanar reformation of individually oriented bundles (1016).
We found that the presence of tunnel cysts was correlated with arthrometric test results. Tunnel enlargement occurs postoperatively after SB or DB ACL reconstruction. Its etiology is considered to be multifactorial, and the result depend on excess graft motion in the tunnel, fixation methods, improper placement, and osteolytic cytokine reactions. Numerous questions remain to be answered, including those regarding the prevalence of or the association between tunnel cysts and knee stability (171819). We found femoral and tibial cysts were more commonly associated with the PLB than the AMB, and that the cysts on the tibial side were associated with unfavorable clinical scenario. Further study is required to find out the exact mechanism.
Naraghi et al. (20) used anterior translation, PCL angle, and PCL curvature as indirect signs of anterior instability in ACL reconstructed knee. We found that they did not correlate with operative findings in our institute. We believe that this is firstly due to different surgical technique, since they did not specifically include only the double bundle ACL reconstruction, and secondly due to the multi-angular appearance of the PCL on MR images, which was probably associated with postoperative adhesive changes. It would be more useful to use another measurement of PCL buckling such as PCL ratio, as we found in our study (13). In addition, it has been postulated that signs such as, anterior translation of the tibia may reflect concomitant rotational laxity rather than anterior laxity, leading to false results. The PCL ratio may better reflect the overall functional status in the ACL reconstructed knee rather than anterior tibial laxity alone (2021).
Some limitations of the present study require mention. First, our study included patients recruited at a single tertiary medical center and study group was limited. Second, few patients exhibited significant graft failure, causing statistical limitations. Third, although it is known to well-reflect sagittal knee joint laxity, the KT-1000 arthrometer is not immune to systematic errors (2223). Also, it does not measure the rotational laxity, hence limited in ACL evaluation as a whole. Fourth, we did not compare the differences between the 2D and 3D sequence, as the aim of this study was to directly associate the clinical and 3D MRI findings. Fifth, this study was a retrospective study conducted on electronic medical records at time of MRI, and rotational laxity results either by KT arthrometer or physical examination were not available for some patients. Sixth, the surgical techniques used by the three surgeons may have differed, as may have grafts and fixation devices. Lastly, MRI also has limitations, because it is a static test, and thus, evaluations of stability and tear depth and length are not possible.
Nonetheless, MRI is a highly valuable tool for the evaluation of double bundle anatomic ACL reconstruction. Due to the complexity of double bundle ACL anatomy, adding a high spatial resolution 3D sequence to the routine MRI knee protocol (especially for an AMB tear) can aid the evaluation of ACL grafts after double bundle ACL reconstruction.
In order to noninvasively follow up the patients after the double bundle reconstruction, we should try to manipulate the MRI planes to direct visualize the tear of AMB of the graft, intraosseous cysts on the tibial sides of the bundles, or to evaluate the PCL buckling. The other findings such as abnormal curvature or SI of the grafts, impingement or intraarticular synovitis were not related with the clinical laxity in this study. In view of the increasing use of double-bundle ACL reconstruction, radiologists should familiarize themselves with the MR images of patients that have undergone this procedure.
Figures and Tables
![]() | Fig. 1Intraarticular curvature on reformatted MRI.(a) 3D proton density-weighted image (PDWI) was taken in a 42-year-old man 50 months after double-bundle reconstruction. A 3D PDWI shows straight intraarticular curvature of AMB (arrows) from femoral tunnel to tibial tunnel. (b) 3D proton density-weighted image (PDWI) was taken in a 31-year-old man 12 months after double-bundle reconstruction. A 3D PDWI shows smooth arc (arrows) of AMB graft. Small hypointense lesion (arrowhead) anterior to the graft indicates nodular synovitis. (c) 3D proton density-weighted image (PDWI) was taken in a 44-year-old man 25 months after double-bundle reconstruction. A 3D PDWI show angulation of the AMB, showing abrupt change of course in the distal bundle (arrows).
|
![]() | Fig. 2Graft tears on reformatted MRI. (a) 3D proton density-weighted image (PDWI) was taken in a 52-year-old man 17 months after double-bundle reconstruction. A 3D PDWI show marginal fraying of PLB showing irregularity at the posterior margin of the distal graft just before it enters the tibial tunnel (arrow). (b) 3D proton density-weighted image (PDWI) was taken in a 30-year-old man 14 months after double-bundle reconstruction. A 3D PDWI show fluid component within the AMB, with disruption of proximal graft (black arrow). The white arrow indicates the rest of the ACL graft. |
![]() | Fig. 33D proton density-weighted image (PDWI) was taken in a 21-year-old man 12 months after double-bundle reconstruction. A 3D PDWI show bony impingement (black arrow) of an AMB, showing bony spur from femoral condyle (white arrow) abutting the thin and posteriorly sagging graft. |
![]() | Fig. 43D proton density-weighted image (PDWI) was taken in a 38-year-old man 18 months after double-bundle reconstruction. A 3D PDWI show intraosseous cystic lesions in the femoral side of AMB (arrowhead) and the femoral and tibial side of PLB (arrows). |
![]() | Fig. 53D proton density-weighted image (PDWI) was taken in a 43-year-old man 19 months after double-bundle reconstruction. A 3D PDWI show measurement of tibial translation on a MR image. |
![]() | Fig. 63D proton density-weighted image (PDWI) was taken in a 43-year-old man 19 months after double-bundle reconstruction. A 3D PDWI show measurement of PCL angle (a), and PCL ratio (b). |
Table 1
Result of MRI-Based Analysis of Anteromedial and Posterolateral ACL Bundles (unit: number of cases)
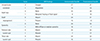
Table 2
Quantitative MRI Results (number of cases)
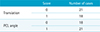
Score | Number of cases | |
---|---|---|
Translation | 0 | 21 |
1 | 18 | |
PCL angle | 0 | 18 |
1 | 21 |
Table 3
Second-Look Arthroscopy Results (number of cases)
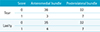
Score | Anteromedial bundle | Posterolateral bundle | |
---|---|---|---|
Tear | 0 | 36 | 32 |
1 | 3 | 7 | |
Laxity | 0 | 35 | 32 |
1 | 4 | 7 |
Table 4
Correlation of Arthroscopic Findings with 3D Isotropic MRI Findings (unit: number of cases)
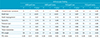
The numbers in the cells are positive MRI cases per positive arthroscopic finding, except the PCL ratio.
The numbers in the row of PCL angle represent the number of patients with the angle below the cut off of 107 degrees per arthroscopic finding.
The numbers in the PCL ratio represent the average of the calculated values in the study group.
AMB = anteromedial bundle of anterior cruciate ligament; PCL = posterior cruciate ligament PLB = posterolateral bundle of anterior cruciate ligament
Table 5
Correlation of KT Arthrometer Value with 3D Isotropic MRI Findings, and Arthroscopic Findings with 3D Isotropic MRI Findings (mean of ranges)
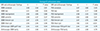
References
1. Csintalan RP, Inacio MC, Funahashi TT. Incidence rate of anterior cruciate ligament reconstructions. Perm J. 2008; 12:17–21.
2. Bach BR Jr, Tradonsky S, Bojchuk J, Levy ME, Bush-Joseph CA, Khan NH. Arthroscopically assisted anterior cruciate ligament reconstruction using patellar tendon autograft. Five- to nine-year follow-up evaluation. Am J Sports Med. 1998; 26:20–29.
3. Shelbourne KD, Gray T. Anterior cruciate ligament reconstruction with autogenous patellar tendon graft followed by accelerated rehabilitation. A two- to nine-year followup. Am J Sports Med. 1997; 25:786–795.
4. Asagumo H, Kimura M, Kobayashi Y, Taki M, Takagishi K. Anatomic reconstruction of the anterior cruciate ligament using double-bundle hamstring tendons: surgical techniques, clinical outcomes, and complications. Arthroscopy. 2007; 23:602–609.
5. Tanaka Y, Yonetani Y, Shiozaki Y, et al. MRI analysis of single-, double-, and triple-bundle anterior cruciate ligament grafts. Knee Surg Sports Traumatol Arthrosc. 2014; 22:1541–1548.
6. Recht MP, Kramer J. MR imaging of the postoperative knee: a pictorial essay. Radiographics. 2002; 22:765–774.
7. Papakonstantinou O, Chung CB, Chanchairujira K, Resnick DL. Complications of anterior cruciate ligament reconstruction: MR imaging. Eur Radiol. 2003; 13:1106–1117.
8. Ishibashi Y, Tsuda E, Fukuda A, Tsukada H, Toh S. Stability evaluation of single-bundle and double-bundle reconstruction during navigated ACL reconstruction. Sports Med Arthrosc. 2008; 16:77–83.
9. Kim SI, Park HJ, Lee SY, et al. Usefulness of oblique coronal and sagittal MR images of the knee after double-bundle and selective anterior cruciate ligament reconstructions. Acta Radiol. 2015; 56:312–321.
10. Duc SR, Pfirrmann CW, Koch PP, Zanetti M, Hodler J. Internal knee derangement assessed with 3-minute three-dimensional isovoxel true FISP MR sequence: preliminary study. Radiology. 2008; 246:526–535.
11. Vahey TN, Hunt JE, Shelbourne KD. Anterior translocation of the tibia at MR imaging: a secondary sign of anterior cruciate ligament tear. Radiology. 1993; 187:817–819.
12. Gentili A, Seeger LL, Yao L, Do HM. Anterior cruciate ligament tear: indirect signs at MR imaging. Radiology. 1994; 193:835–840.
13. Liu SH, Osti L, Dorey F, Yao L. Anterior cruciate ligament tear. A new diagnostic index on magnetic resonance imaging. Clin Orthop Relat Res. 1994; 147–150.
14. Fleming BC, Brattbakk B, Peura GD, Badger GJ, Beynnon BD. Measurement of anterior-posterior knee laxity: a comparison of three techniques. J Orthop Res. 2002; 20:421–426.
15. Amis AA. The functions of the fibre bundles of the anterior cruciate ligament in anterior drawer, rotational laxity and the pivot shift. Knee Surg Sports Traumatol Arthrosc. 2012; 20:613–620.
16. Steckel H, Vadala G, Davis D, Fu FH. 2D and 3D 3-tesla magnetic resonance imaging of the double bundle structure in anterior cruciate ligament anatomy. Knee Surg Sports Traumatol Arthrosc. 2006; 14:1151–1158.
17. Bencardino JT, Beltran J, Feldman MI, Rose DJ. MR imaging of complications of anterior cruciate ligament graft reconstruction. Radiographics. 2009; 29:2115–2126.
18. Ghazikhanian V, Beltran J, Nikac V, Feldman M, Bencardino JT. Tibial tunnel and pretibial cysts following ACL graft reconstruction: MR imaging diagnosis. Skeletal Radiol. 2012; 41:1375–1379.
19. Neddermann A, Willbold E, Witte F, et al. Tunnel widening after anterior cruciate ligament reconstruction: an experimental study in sheep. Am J Sports Med. 2009; 37:1609–1617.
20. Naraghi AM, Gupta S, Jacks LM, Essue J, Marks P, White LM. Anterior cruciate ligament reconstruction: MR imaging signs of anterior knee laxity in the presence of an intact graft. Radiology. 2012; 263:802–810.
21. Zampeli F, Ntoulia A, Giotis D, et al. The PCL index is correlated with the control of rotational kinematics that is achieved after anatomic anterior cruciate ligament reconstruction. Am J Sports Med. 2014; 42:665–674.
22. Barcellona MG, Morrissey MC, Milligan P, Amis AA. The effect of thigh muscle activity on anterior knee laxity in the uninjured and anterior cruciate ligament-injured knee. Knee Surg Sports Traumatol Arthrosc. 2014; 22:2821–2829.
23. Arneja S, Leith J. Review article: Validity of the KT-1000 knee ligament arthrometer. J Orthop Surg (Hong Kong). 2009; 17:77–79.