Abstract
Since induced pluripotent stem cell (iPSC) was first in-troduced by Yamanaka in 2006, it took only six years to win a Nobel Prize for his pioneering work. It is unusual to win a Nobel Prize for such recent research with a short history. Many scientists and clinicians are interested in iPSC for its potential application. Significant progression in this field has been made, while there remain many hurdles to overcome for application of iPSC technique in real clinics. In this review, the concept of reprogramming and the basic techniques of iPSC generation will be discussed for the reader's convenience, followed by discussion of recent progress, followed by the topics of “disease modeling” and “cell therapy” with iPSC in the second half of this article. Several examples of rheumatologic application of iPSC will be provided in the main text. If rheumatologists could understand the merits and potentials of iPSC, opportunities for innovative research and therapy can be expanded.
References
1. Somorjai IM, Lohmann JU, Holstein TW, Zhao Z. Stem cells: a view from the roots. Biotechnol J. 2012; 7:704–22.


2. Schugar RC, Robbins PD, Deasy BM. Small molecules in stem cell self-renewal and differentiation. Gene Ther. 2008; 15:126–35.


3. Grskovic M, Javaherian A, Strulovici B, Daley GQ. Induced pluripotent stem cells–opportunities for disease modelling and drug discovery. Nat Rev Drug Discov. 2011; 10:915–29.
4. Murry CE, Keller G. Differentiation of embryonic stem cells to clinically relevant populations: lessons from embryonic development. Cell. 2008; 132:661–80.
5. Ambasudhan R, Talantova M, Coleman R, Yuan X, Zhu S, Lipton SA, et al. Direct reprogramming of adult human fibroblasts to functional neurons under defined conditions. Cell Stem Cell. 2011; 9:113–8.


7. Takahashi K, Tanabe K, Ohnuki M, Narita M, Ichisaka T, Tomoda K, et al. Induction of pluripotent stem cells from adult human fibroblasts by defined factors. Cell. 2007; 131:861–72.


8. Takahashi K, Yamanaka S. Induction of pluripotent stem cells from mouse embryonic and adult fibroblast cultures by defined factors. Cell. 2006; 126:663–76.


9. Lowry WE, Richter L, Yachechko R, Pyle AD, Tchieu J, Sridharan R, et al. Generation of human induced pluripotent stem cells from dermal fibroblasts. Proc Natl Acad Sci U S A. 2008; 105:2883–8.


10. Stadtfeld M, Hochedlinger K. Induced pluripotency: history, mechanisms, and applications. Genes Dev. 2010; 24:2239–63.


11. Maherali N, Sridharan R, Xie W, Utikal J, Eminli S, Arnold K, et al. Directly reprogrammed fibroblasts show global epigenetic remodeling and widespread tissue contribution. Cell Stem Cell. 2007; 1:55–70.
12. Wilmut I, Schnieke AE, McWhir J, Kind AJ, Campbell KH. Viable offspring derived from fetal and adult mam-malian cells. Nature. 1997; 385:810–3.


13. Wakayama T, Hayashi Y, Ogura A. Participation of the female pronucleus derived from the second polar body in full embryonic development of mice. J Reprod Fertil. 1997; 110:263–6.


14. Freberg CT, Dahl JA, Timoskainen S, Collas P. Epigenetic reprogramming of OCT4 and NANOG regulatory regions by embryonal carcinoma cell extract. Mol Biol Cell. 2007; 18:1543–53.
15. Taranger CK, Noer A, S⊘rensen AL, Håkelien AM, Boquest AC, Collas P. Induction of dedifferentiation, ge-nomewide transcriptional programming, and epigenetic reprogramming by extracts of carcinoma and embryonic stem cells. Mol Biol Cell. 2005; 16:5719–35.


16. Tada M, Morizane A, Kimura H, Kawasaki H, Ainscough JF, Sasai Y, et al. Pluripotency of reprogrammed somatic genomes in embryonic stem hybrid cells. Dev Dyn. 2003; 227:504–10.


17. Yu J, Vodyanik MA, He P, Slukvin II, Thomson JA. Human embryonic stem cells reprogram myeloid precursors following cell-cell fusion. Stem Cells. 2006; 24:168–76.


18. Okita K, Ichisaka T, Yamanaka S. Generation of germ-line-competent induced pluripotent stem cells. Nature. 2007; 448:313–7.


19. Yu J, Vodyanik MA, Smuga-Otto K, Antosiewicz-Bourget J, Frane JL, Tian S, et al. Induced pluripotent stem cell lines derived from human somatic cells. Science. 2007; 318:1917–20.


20. Lenardo MJ, Staudt L, Robbins P, Kuang A, Mulligan RC, Baltimore D. Repression of the IgH enhancer in ter-atocarcinoma cells associated with a novel octamer factor. Science. 1989; 243:544–6.


21. Okamoto K, Okazawa H, Okuda A, Sakai M, Muramatsu M, Hamada H. A novel octamer binding transcription factor is differentially expressed in mouse embryonic cells. Cell. 1990; 60:461–72.


22. Niwa H, Miyazaki J, Smith AG. Quantitative expression of Oct-3/4 defines differentiation, dedifferentiation or self-renewal of ES cells. Nat Genet. 2000; 24:372–6.


23. Wu da Y, Yao Z. Isolation and characterization of the murine Nanog gene promoter. Cell Res. 2005; 15:317–24.


24. Yuan H, Corbi N, Basilico C, Dailey L. Developmen-tal-specific activity of the FGF-4 enhancer requires the synergistic action of Sox2 and Oct-3. Genes Dev. 1995; 9:2635–45.


25. Tomioka M, Nishimoto M, Miyagi S, Katayanagi T, Fukui N, Niwa H, et al. Identification of Sox-2 regulatory region which is under the control of Oct-3/4-Sox-2 complex. Nucleic Acids Res. 2002; 30:3202–13.


26. Avilion AA, Nicolis SK, Pevny LH, Perez L, Vivian N, Lovell-Badge R. Multipotent cell lineages in early mouse development depend on SOX2 function. Genes Dev. 2003; 17:126–40.


27. Ivanova N, Dobrin R, Lu R, Kotenko I, Levorse J, DeCoste C, et al. Dissecting self-renewal in stem cells with RNA interference. Nature. 2006; 442:533–8.


28. Seoane J, Le HV, Massagué J. Myc suppression of the p21(Cip1) Cdk inhibitor influences the outcome of the p53 response to DNA damage. Nature. 2002; 419:729–34.


29. Lewitzky M, Yamanaka S. Reprogramming somatic cells towards pluripotency by defined factors. Curr Opin Biotechnol. 2007; 18:467–73.


30. Li Y, McClintick J, Zhong L, Edenberg HJ, Yoder MC, Chan RJ. Murine embryonic stem cell differentiation is promoted by SOCS-3 and inhibited by the zinc finger transcription factor Klf4. Blood. 2005; 105:635–7.


31. Nakagawa M, Koyanagi M, Tanabe K, Takahashi K, Ichisaka T, Aoi T, et al. Generation of induced pluripotent stem cells without Myc from mouse and human fibroblasts. Nat Biotechnol. 2008; 26:101–6.


32. Kim JB, Sebastiano V, Wu G, Araúzo-Bravo MJ, Sasse P, Gentile L, et al. Oct4-induced pluripotency in adult neural stem cells. Cell. 2009; 136:411–9.


33. Si-Tayeb K, Noto FK, Sepac A, Sedlic F, Bosnjak ZJ, Lough JW, et al. Generation of human induced pluripotent stem cells by simple transient transfection of plasmid DNA encoding reprogramming factors. BMC Dev Biol. 2010; 10:81.


34. Anokye-Danso F, Trivedi CM, Juhr D, Gupta M, Cui Z, Tian Y, et al. Highly efficient miRNA-mediated reprogramming of mouse and human somatic cells to pluripotency. Cell Stem Cell. 2011; 8:376–88.


35. Kim D, Kim CH, Moon JI, Chung YG, Chang MY, Han BS, et al. Generation of human induced pluripotent stem cells by direct delivery of reprogramming proteins. Cell Stem Cell. 2009; 4:472–6.


36. Park IH, Arora N, Huo H, Maherali N, Ahfeldt T, Shimamura A, et al. Disease-specific induced pluripotent stem cells. Cell. 2008; 134:877–86.


37. Tiscornia G, Vivas EL, Izpisúa Belmonte JC. Diseases in a dish: modeling human genetic disorders using induced pluripotent cells. Nat Med. 2011; 17:1570–6.


38. Moser HW. Adrenoleukodystrophy: phenotype, genetics, pathogenesis and therapy. Brain. 1997; 120:1485–508.


39. Urbach A, Bar-Nur O, Daley GQ, Benvenisty N. Differential modeling of fragile X syndrome by human embryonic stem cells and induced pluripotent stem cells. Cell Stem Cell. 2010; 6:407–11.


40. Saha K, Jaenisch R. Technical challenges in using human induced pluripotent stem cells to model disease. Cell Stem Cell. 2009; 5:584–95.


41. Lee G, Papapetrou EP, Kim H, Chambers SM, Tomishima MJ, Fasano CA, et al. Modelling pathogenesis and treatment of familial dysautonomia using patient-specific iPSCs. Nature. 2009; 461:402–6.


42. Soldner F, Hockemeyer D, Beard C, Gao Q, Bell GW, Cook EG, et al. Parkinson's disease patient-derived induced pluripotent stem cells free of viral reprogramming factors. Cell. 2009; 136:964–77.


43. Dimos JT, Rodolfa KT, Niakan KK, Weisenthal LM, Mitsumoto H, Chung W, et al. Induced pluripotent stem cells generated from patients with ALS can be differentiated into motor neurons. Science. 2008; 321:1218–21.


44. Itzhaki I, Maizels L, Huber I, Zwi-Dantsis L, Caspi O, Winterstern A, et al. Modelling the long QT syndrome with induced pluripotent stem cells. Nature. 2011; 471:225–9.


45. Ebert AD, Yu J, Rose FF Jr, Mattis VB, Lorson CL, Thomson JA, et al. Induced pluripotent stem cells from a spinal muscular atrophy patient. Nature. 2009; 457:277–80.


46. Carvajal-Vergara X, Sevilla A, D'Souza SL, Ang YS, Schaniel C, Lee DF, et al. Patient-specific induced pluripotent stem-cell-derived models of LEOPARD syndrome. Nature. 2010; 465:808–12.


47. Robinton DA, Daley GQ. The promise of induced pluripotent stem cells in research and therapy. Nature. 2012; 481:295–305.


48. Raya A, Rodríguez-Pizà I, Guenechea G, Vassena R, Navarro S, Barrero MJ, et al. Disease-corrected haemato-poietic progenitors from Fanconi anaemia induced pluripotent stem cells. Nature. 2009; 460:53–9.


49. Wernig M, Zhao JP, Pruszak J, Hedlund E, Fu D, Soldner F, et al. Neurons derived from reprogrammed fibroblasts functionally integrate into the fetal brain and improve symptoms of rats with Parkinson's disease. Proc Natl Acad Sci U S A. 2008; 105:5856–61.


50. Hanna J, Wernig M, Markoulaki S, Sun CW, Meissner A, Cassady JP, et al. Treatment of sickle cell anemia mouse model with iPS cells generated from autologous skin. Science. 2007; 318:1920–3.


51. Huangfu D, Osafune K, Maehr R, Guo W, Eijkelenboom A, Chen S, et al. Induction of pluripotent stem cells from primary human fibroblasts with only Oct4 and Sox2. Nat Biotechnol. 2008; 26:1269–75.


52. Lyssiotis CA, Foreman RK, Staerk J, Garcia M, Mathur D, Markoulaki S, et al. Reprogramming of murine fibroblasts to induced pluripotent stem cells with chemical complementation of Klf4. Proc Natl Acad Sci U S A. 2009; 106:8912–7.


53. Okita K, Nakagawa M, Hyenjong H, Ichisaka T, Yamanaka S. Generation of mouse induced pluripotent stem cells without viral vectors. Science. 2008; 322:949–53.


54. Lujan E, Chanda S, Ahlenius H, Südhof TC, Wernig M. Direct conversion of mouse fibroblasts to self-renewing, tripotent neural precursor cells. Proc Natl Acad Sci U S A. 2012; 109:2527–32.


55. Han DW, Tapia N, Hermann A, Hemmer K, Höing S, Araúzo-Bravo MJ, et al. Direct reprogramming of fibroblasts into neural stem cells by defined factors. Cell Stem Cell. 2012; 10:465–72.


56. Szabo E, Rampalli S, Risueño RM, Schnerch A, Mitchell R, Fiebig-Comyn A, et al. Direct conversion of human fibroblasts to multilineage blood progenitors. Nature. 2010; 468:521–6.


57. Vierbuchen T, Ostermeier A, Pang ZP, Kokubu Y, Südhof TC, Wernig M. Direct conversion of fibroblasts to functional neurons by defined factors. Nature. 2010; 463:1035–41.


58. Huangfu D, Maehr R, Guo W, Eijkelenboom A, Snitow M, Chen AE, et al. Induction of pluripotent stem cells by defined factors is greatly improved by small-molecule compounds. Nat Biotechnol. 2008; 26:795–7.


59. Ichida JK, Blanchard J, Lam K, Son EY, Chung JE, Egli D, et al. A small-molecule inhibitor of tgf-Beta signaling replaces sox2 in reprogramming by inducing nanog. Cell Stem Cell. 2009; 5:491–503.
60. Zhu S, Li W, Zhou H, Wei W, Ambasudhan R, Lin T, et al. Reprogramming of human primary somatic cells by OCT4 and chemical compounds. Cell Stem Cell. 2010; 7:651–5.


61. Hockemeyer D, Soldner F, Beard C, Gao Q, Mitalipova M, DeKelver RC, et al. Efficient targeting of expressed and silent genes in human ESCs and iPSCs using zinc-fin-ger nucleases. Nat Biotechnol. 2009; 27:851–7.


62. Mali P, Ye Z, Hommond HH, Yu X, Lin J, Chen G, et al. Improved efficiency and pace of generating induced pluripotent stem cells from human adult and fetal fibroblasts. Stem Cells. 2008; 26:1998–2005.


63. Sun N, Panetta NJ, Gupta DM, Wilson KD, Lee A, Jia F, et al. Feeder-free derivation of induced pluripotent stem cells from adult human adipose stem cells. Proc Natl Acad Sci U S A. 2009; 106:15720–5.


64. Lin C, Yu C, Ding S. Toward directed reprogramming through exogenous factors. Curr Opin Genet Dev. 2013; 23:519–25.


Figure 1.
Vector structure of 4-in-1 lentivirus. 4-in-1 lentiviral vector contains 4 Yamanaka factors of Oct4, Klf4, Sox2, and c-Myc.
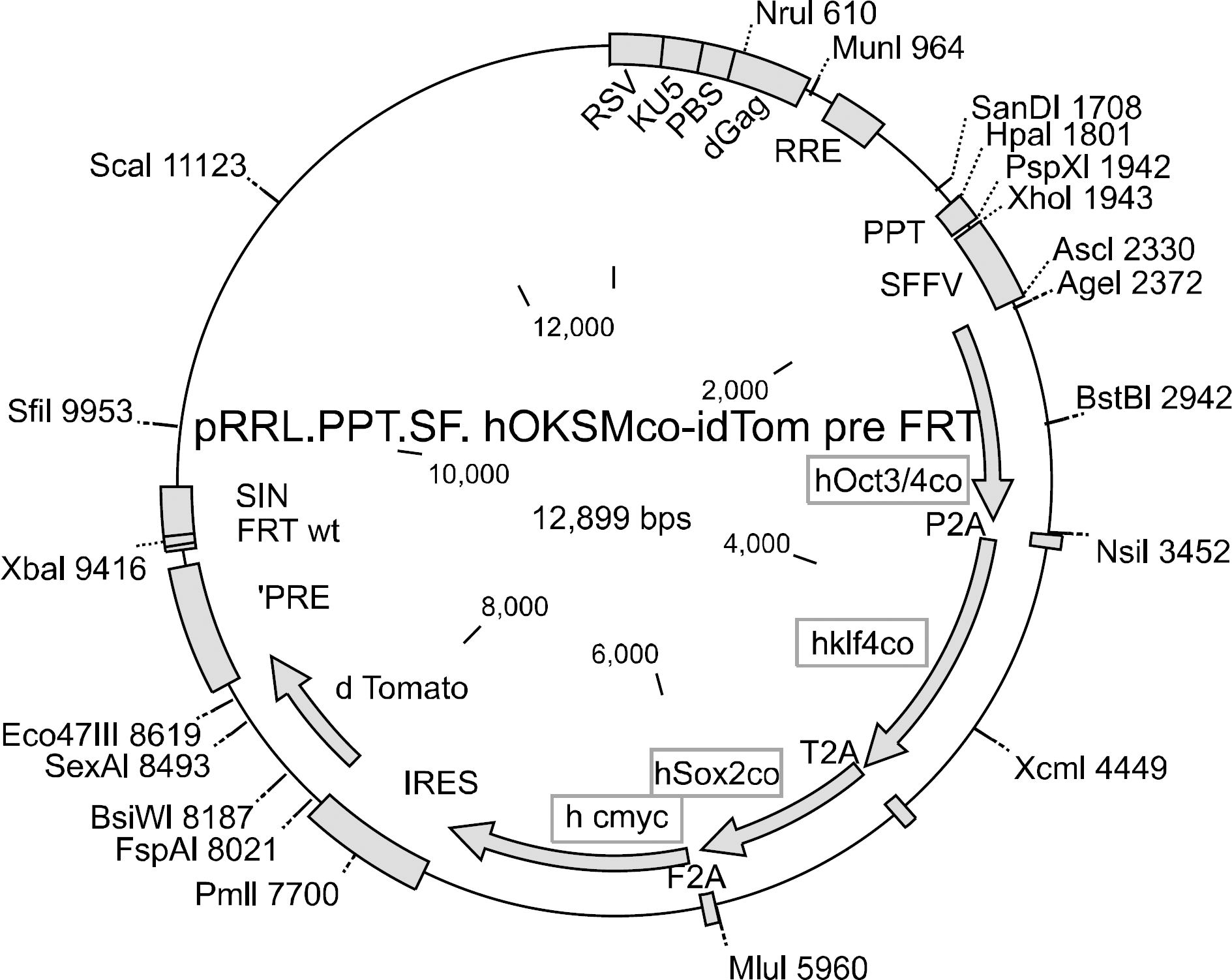
Figure 2.
Overall process of reprogramming. 293 T cells are used for production of 4-in-1 lentivirus. Lentivirus is transduced into dermal fibroblasts. After 1∼2 weeks, cells are transformed into stem cell like morphology. After 2∼3 weeks, stem cell colonies are formed and then picked up for cultivating stem cell lines.
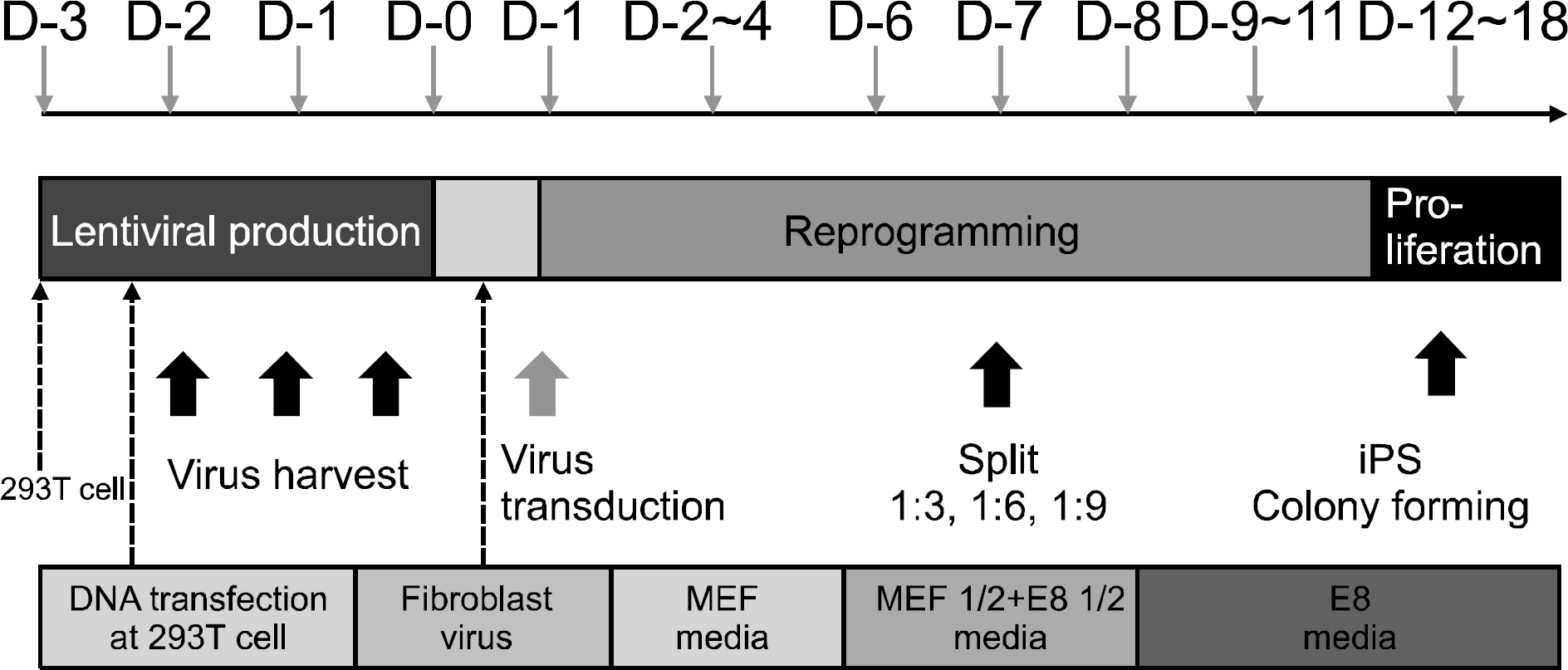
Figure 3.
Summarized images during the process of iPSC reprogramming. Fibroblast begins to be changed into the shapes of self-proliferating and colony-like feature. Background fibroblasts are not reprogrammed so they remain spindle shapes. Generated three colonies are positive for pluripotency markers.
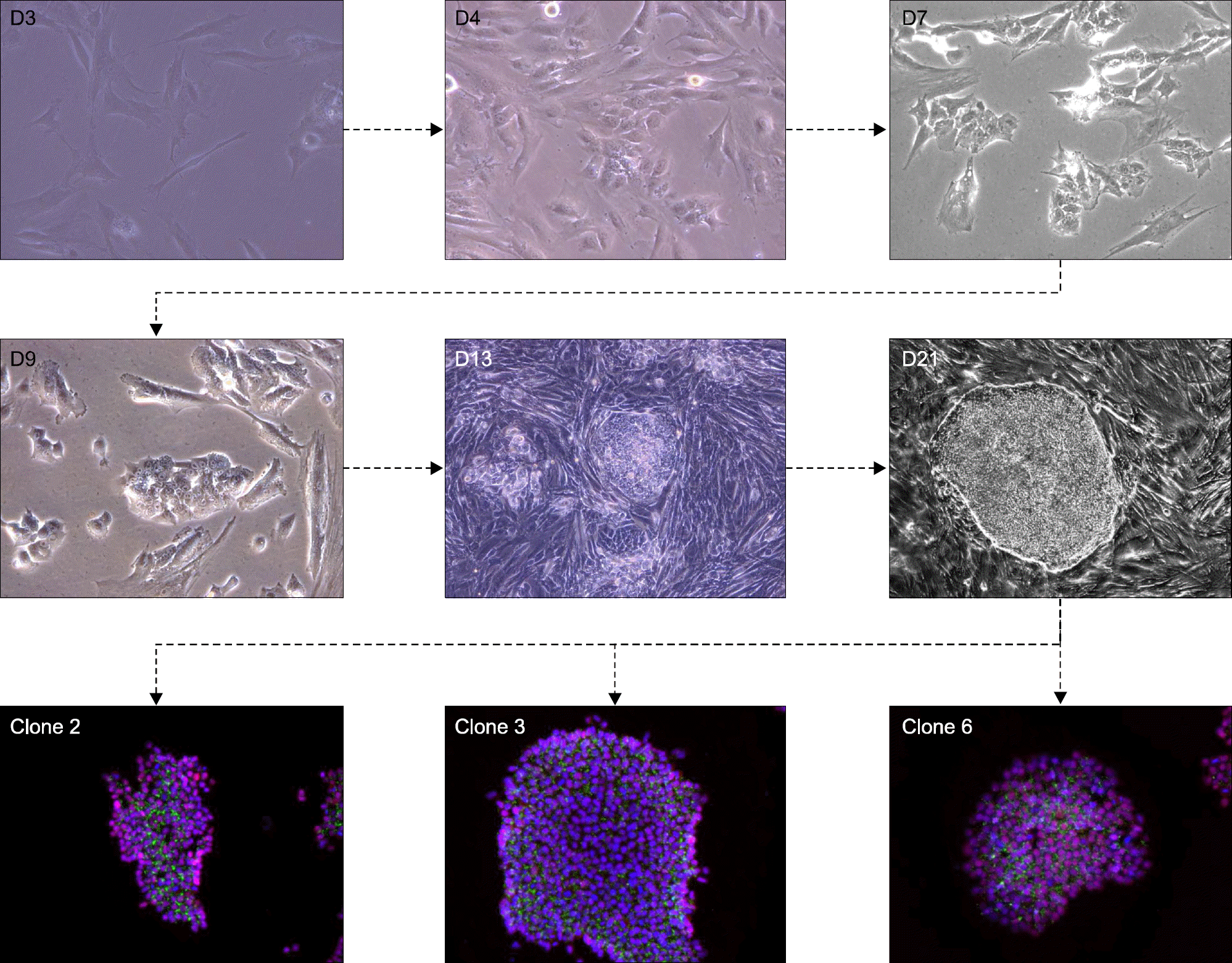
Figure 4.
Pluripotency markers are positive at generated clones. Immunofluorescence staining shows positive pluripotency markers such as Nanog, SSEA4, Sox2, Tra-1-81, Oct4, Tra-1-60. Embryonic stem cells and iPSC are alike in positivity of immunofluoresence study.
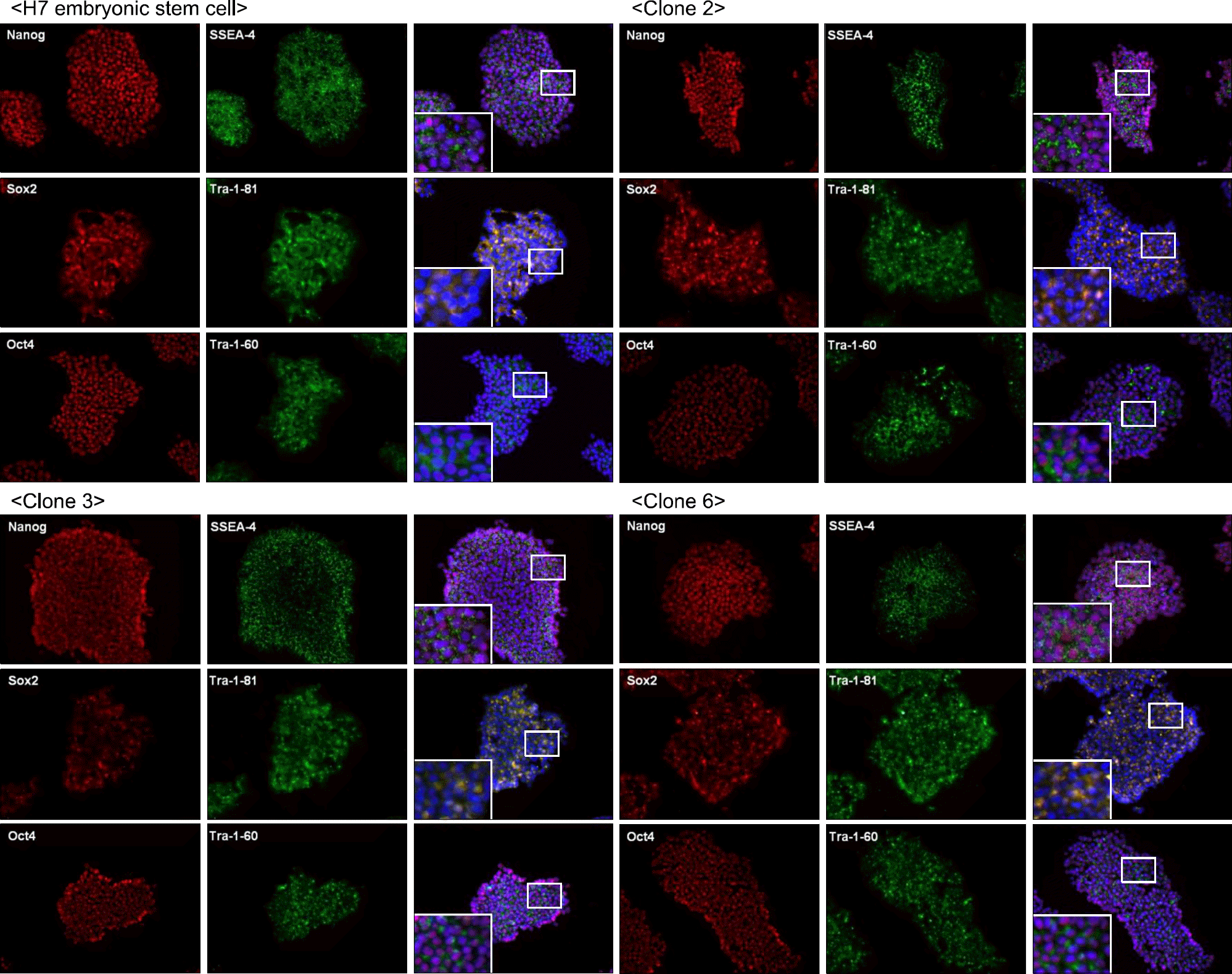
Figure 5.
Various levels of studies with disease-specific iPSC. OA FLS and RA FLS are compared with each other at fibroblast level. They are also studied when they are reprogrammed into iPSC clones. They can be differentiated into target disease cells such as chondrocytes and osteocytes. If we could find any differences in OA&RA iPSC-differentiated chondrocytes, it would be a promising methodology for exploring disease mechanism.
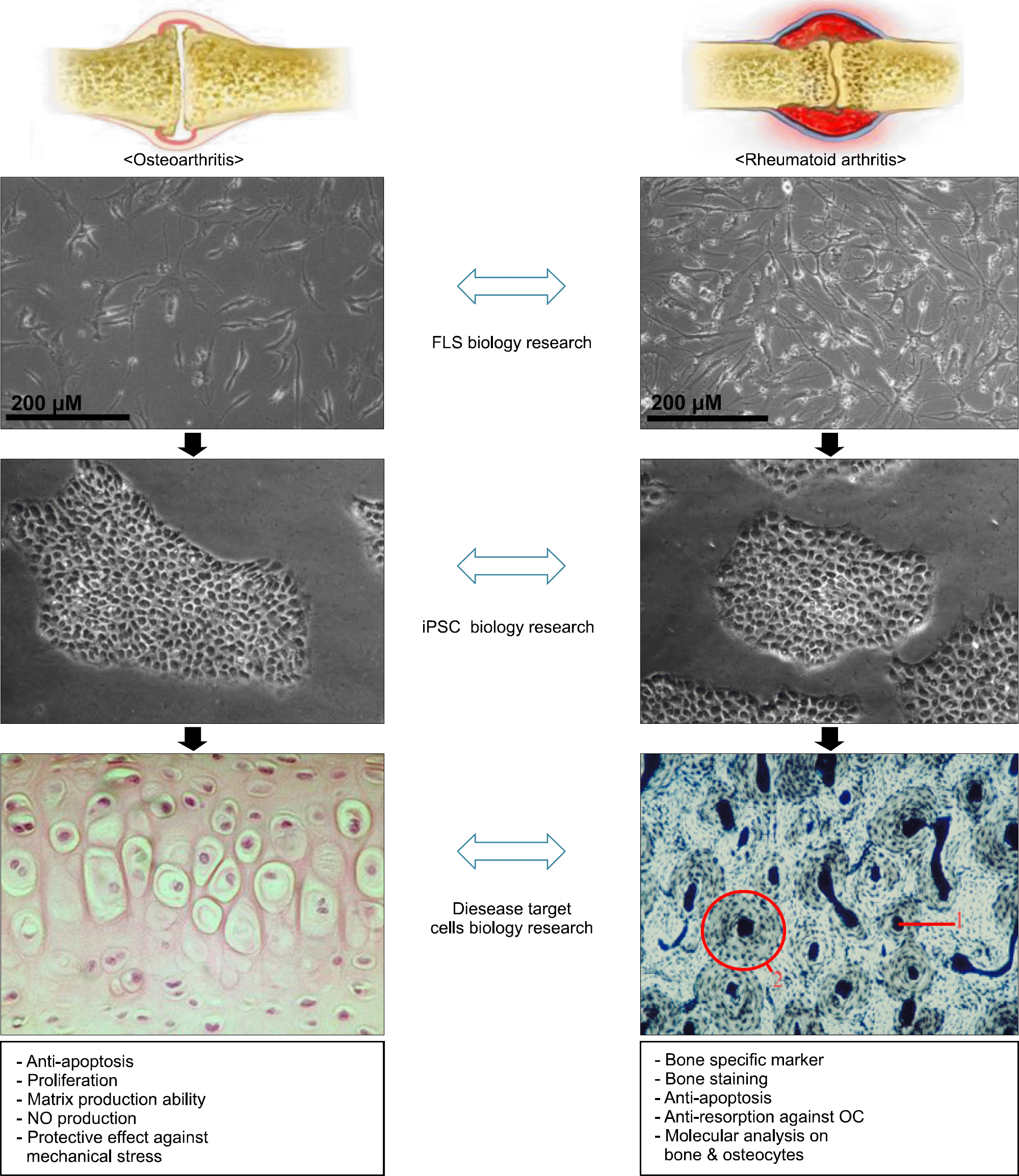
Figure 6.
An example of application of iPSC in disease modeling in future. iPSC from patients are differentiated into syno-viocytes, T cells, B cells and so on. After methotrexate is added in culture dishes, and then the effects of MTX on target cells could be studied.
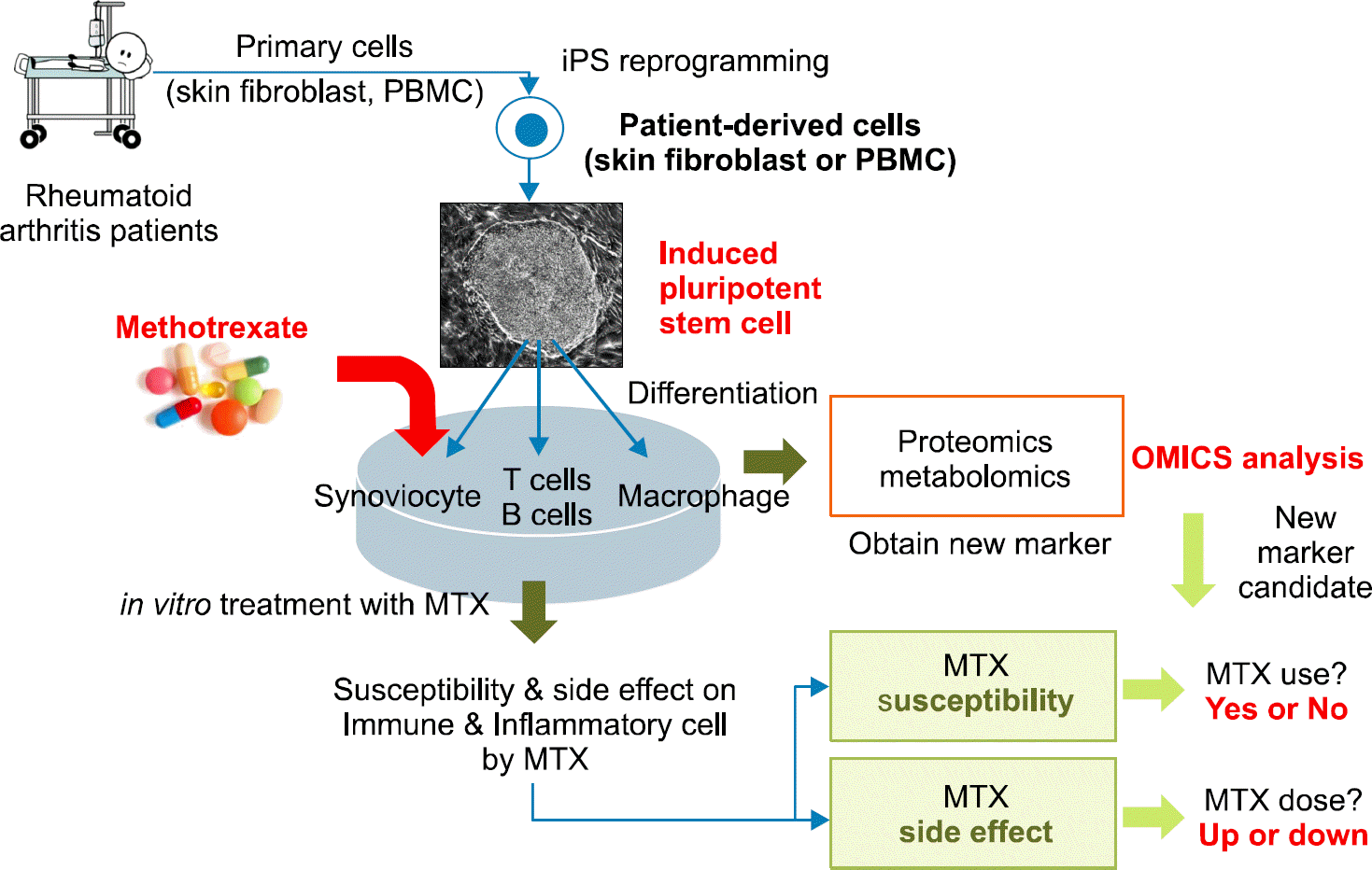
Table 1.
Application of iPSC technique in disease modelling