Abstract
Background
The incidence of atherosclerosis is well correlated with the progression of type 2 diabetes mellitus. High plasma glucose in uncontrolled diabetic patients evokes many vascular complications such as atherosclerosis. Specifically, high glucose was reported to induce thrombospondin-1 (TSP-1), which activates matrix metalloproteinase-2 (MMP-2) and leads to the invasion of vascular smooth muscle cells (VSMCs) into the intima. Catechins with antioxidant effects are known to inhibit MMP-2 activity. Therefore, this study was aimed at revealing the effect of epicatechin, one of catechins, on high glucose-induced TSP-1 and the invasiveness of VSMCs.
Methods
VSMCs were primarily isolated from Sprague-Dawley rat aorta. The VSMCs were incubated with different doses (30, 100 and 300 µM) of epicatechin under high glucose concentration (30 mM). The TSP-1 protein and mRNA expressions were analyzed by performing Western blotting and Northern blot analyses, respectively. RT-PCR was performed to observe the MMP-2 mRNA expression. Gelatin zymography was performed for the measurement of MMP-2 activity. Invasion assays were performed to evaluate the invasiveness of VSMCs.
Figures and Tables
Fig. 1
The effects of high glucose on the expression of thrombospondin-1 (TSP-1) protein in rat aortic vascular smooth muscle cells (VSMCs). VSMCs were incubated with glucose (GLU, 5.6, 11.1, and 30 mM) for 48 h. (A) Total proteins (30 µg) of cellular lysate (cytosol) and medium were separated and analyzed on a 8% SDS-PAGE, and Western blot analysis was performed as described in Methods. Equal loading of cellular lysate was verified by probing the same blot for β-tubulin. (B) TSP-1 levels of cytosol and medium were determined by scanning densitometry of immunoblots. TSP-1 levels of cytosol and media under 5.6 mM glucose were set to 1. Data are expressed as mean ± SD from three independent experiments.
*P < 0.05 vs. 5.6 mM GLU.
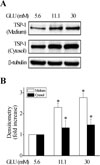
Fig. 2
The effects of epicatechin (EC) on high glucoseinduced thrombospondin-1 (TSP-1) protein in rat aortic vascular smooth muscle cells (VSMCs). VSMCs were incubated with glucose (GLU, 5.6 and 30 mM) and EC (30, 100, and 300 µM) for 48 h. (A) Total proteins (30 µg) of cellular lysate (cytosol) and medium were separated and analyzed on a 8% SDS-PAGE, and Western blot analysis was performed as described in Methods. Equal loading of cellular lysate was verified by probing the same blot for β-tubulin. (B) and (C) TSP-1 levels in cytosol (B) and medium (C) were determined by scanning densitometry of immunoblots. TSP-1 levels of cytosol and medium under 5.6 mM glucose without EC were set to 1. Data are expressed as mean ± SD from three independent experiments.
*P < 0.05 vs. 5.6 mM GLU without EC.
†P < 0.05 for 30 mM GLU with EC vs. 30 mM GLU.
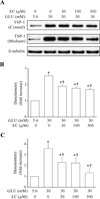
Fig. 3
The effects of epicatechin (EC) on high glucose-induced thrombospondin-1 (TSP-1) mRNA expression in rat aortic vascular smooth muscle cells (VSMCs). (A) VSMCs were incubated with glucose (GLU, 5.6 and 30 mM) or EC (30, 100, and 300 µM) for 24 h. The levels of TSP-1 mRNAs were measured by Northern blot analysis. Equal loading of sample (10 µg) was verified by the ribosomal 18S and 28S bands. (B) The levels of TSP-1 mRNAs expressed under 5.6 mM glucose without EC were set to 1. Data are expressed as mean ± SD from three independent experiments.
*P < 0.05 vs. 5.6 mM GLU without EC.
†P < 0.05 for 30 mM GLU with EC vs. 30 mM GLU.
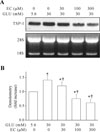
Fig. 4
The effects of epicatechin (EC) on the expression and activity of matrix metalloproteinase-2 (MMP-2) in rat aortic vascular smooth muscle cells (VSMCs). (A) VSMCs were incubated with glucose (GLU, 5.6 and 30 mM) with EC (30, 100, and 300 µM) for 24 h. The expressions of MMP-2 and GAPDH (as an internal control) mRNAs were measured by reverse transcription-polymerase chain reaction (RT-PCR). The PCR products were resolved on a 1% agarose gel and stained with ethidium bromide. (B) The activity of MMP-2 was measured by gelatin zymography. Medium (25 µg) were separated and analyzed on an 8% polyacrylamide gel including gelatin (1 g/L) as described in Methods. (C) By scanning densitometry, level of MMP-2 activity under 5.6 mM GLU without EC was set to 1. Data are expressed as mean ± SD from three independent experiments.
*, P < 0.05 vs. 5.6 mM GLU without EC.
†, P < 0.05 for 30 mM GLU with EC vs. 30 mM GLU.
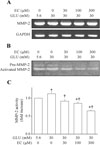
Fig. 5
The effects of epicatechin (EC) on the invasiveness of rat aortic vascular smooth muscle cells (VSMCs) using Matrigel-coated filters of Boyden chamber. VSMCs (2×105 cells/mL) were placed into the upper chamber of a Boyden chamber and were incubated with glucose (GLU, 5.6 and 30 mM) and EC (30, 100, and 300 µM) for 24 h. VSMCs migrated through Matrigel-coated filters to the lower surface were fixed with 2% paraformaldehyde and were stained with hematoxylin, and were counted for 5 high-power fields per well. The migrated VSMCs under 5.6 mM GLU without EC were set to 1. Data are expressed as mean ± SD from three independent experiments.
*, P < 0.05 vs. 5.6 mM GLU without EC.
†, P < 0.05 for 30 mM GLU with EC vs. 30 mM GLU.
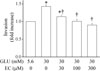
References
1. UK Prospective Diabetes Study (UKPDS) Group. Intensive blood-glucose control with sulfonylureas or insulin compared with conventional treatment and risk of complications in patients with type 2 diabetes (UKPDS 33). Lancet. 1998. 352:837–853.
2. Ruderman N, Haudenschild C. Diabetes as an atherogenic factor. Prog Cardiovasc Dis. 1984. 329:977–986.
3. Graier WF, Grubenthal I, Dittrich P, Wascher TC, Kostner GM. Intracellular mechanism of high D-glucose-induced modulation of vascular cell proliferation. Eur J Pharmacol. 1995. 294:221–229.
4. Pauly RR, Passaniti A, Bilato C, Monticone R, Cheng L, Papadopoulos N, Gluzband YA, Smith L, Weinstein C, Lakatta EG, Crow MT. Migration of cultured vascular smooth muscle cells through a basement membrane barrier requires type IV collagenase activity and is inhibited by cellular differentiation. Circ Res. 1994. 75:41–54.
5. Mason DP, Kenagy RD, Hasenstab D, Bowen-Pope DF, Seifert RA, Coats S, Hawkins SM, Clowes AW. Matrix metalloproteinase-9 overexpression enhances vascular smooth muscle cell migration and alters remodeling in the injured rat carotid artery. Circ Res. 1999. 85:1179–1185.
6. Hao F, Yu JD. High glucose enhance expression of matrix metalloproteinase-2 in smooth muscle cells. Acta Pharmacol Sin. 2003. 24:534–538.
7. Baenziger NL, Brodie GN, Majerus PW. A thrombin-sensitive protein of human platelet membranes. Proc Natl Acad Sci U S A. 1971. 68:240–243.
8. Lawler J. The structural and functional properties of thrombospondin. Blood. 1986. 67:1197–1209.
9. Clezardin P, Lawler J, Amiral J, Quentin G, Delmas P. Identification of cell adhesive sites in the N-terminal domain of thrombospondin-1. Biochem J. 1997. 321:819–827.
10. Poczatek MH, Hugo C, Darley-Usmar V, Murphy-Ullrich JE. Glucose stimulation of transforming growth factor-beta bioactivity in mesangial cells is mediated by thrombospondin-1. Am J Pathol. 2000. 157:1353–1363.
11. Wang S, Skorczewski J, Feng X, Mei L, Murphy-Ullrich JE. Glucose up-regulates thrombospondin 1 gene transcription and transforming growth factor-beta activity through antagonism of cGMP-dependent protein kinase repression via upstream stimulatory factor 2. J Biol Chem. 2004. 279:34311–34322.
12. Stenina OI, Krukovets I, Wang K, Zhou Z, Forudi F, Penn MS, Topol EJ, Plow EF. Increased expression of thrombospondin-1 in vessel wall of diabetic Zucker rat. Circulation. 2003. 107:3209–3215.
13. Hofmann CS, Sonenshein GE. Green tea polyphenol epigallocatechiin-3 gallate induces apoptosis of proliferating vascular smooth muscle cells via activation of p53. FASEB J. 2003. 17:702–704.
14. Galis ZS, Muszynski M, Sukhova GK, Simon-Morrissey E, Unemori EN, Lark MW, Amento E, Libby P. Cytokine-stimulated human vascular smooth muscle cells synthesize a complement of enzymes required for extracellular matrix digestion. Circ Res. 1994. 75:181–189.
15. Esparza J, Vilardell C, Calvo J, Juan M, Vives J, Urbano-Marquez A, Yague J, Cid MC. Fibronectin upregulates gelatinase B (MMP-9) and induces coordinated expression of gelatinase A (MMP-2) and its activator MT1-MMP (MMP-14) by human T lymphocyte cell lines. A process repressed through RAS/MAP kinase signaling pathways. Blood. 1999. 94:2754–2766.
16. Zempo N, Kenagy RD, Au YP, Bendeck M, Clowes MM, Reidy MA, Clowes AW. Matrix metalloproteinases of vascular wall cells are increased in balloon-injured rat carotid artery. J Vasc Surg. 1994. 20:209–217.
17. Sato H, Takino T, Okada Y, Cao J, Shinagawa A, Yamamoto E, Seiki M. A matrix metalloproteinase expressed on the surface of invasive tumour cells. Nature. 1994. 370:61–65.
18. Lee T, Esemuede N, Sumpio BE, Gahtan V. Thrombospondin-1 induces matrix metalloproteinase-2 activation in vascular smooth muscle cells. J Vasc Surg. 2003. 38:147–154.
19. da Silva EL, Abdalla DS, Terao J. Inhibitory effect of flavonoids on low-density lipoprotein peroxidation catalyzed by mammalian 15-lipoxygenase. IUBMB Life. 2000. 49:289–295.
20. Arts IC, Hollman PC, Feskens EJ, Bueno de Mesquita HB, Kromhout D. Catechin intake might explain the inverse relation between tea consumption and ischemic heart disease: the Zutphen Elderly Study. Am J Clin Nutr. 2001. 74:227–232.
21. Maeda K, Kuzuya M, Cheng XW, Asai T, Kanda S, Tamaya-Mori N, Sasaki T, Shibata T, Iguchi A. Green tea catechins inhibit the cultured smooth muscle cell invasion through the basement barrier. Atherosclerosis. 2003. 166:23–30.
22. Ryu GR, Kang JH, Jang HI, Ko SH, Jeong IK, Rhie DJ, Yoon SH, Hahn SJ, Jo YH, Kim MS, Kim MJ. The role of cAMP/PKA activation on exendin-4-induced cyclin D1 expression in INS-1 cell. J Kor Diabetes Assoc. 2005. 29:295–303.
23. Kim MJ, Ryu GR, Kang JH, Sim SS, Min do S, Rhie DJ, Yoon SH, Hahn SJ, Jeong IK, Hong KJ, Kim MS, Jo YH. Inhibitory effects of epicatechin on interleukin-1beta-induced inducible nitric oxide synthase expression in RINm5F cells and rat pancreatic islets by down-regulation of NF-kappaB activation. Biochem Pharmacol. 2004. 68:1775–1785.
24. Albini A, Iwamoto Y, Kleinman HK, Martin GR, Aaronson SA, Kozlowski JM, McEwan RN. A rapid in vitro assay for quantitating the invasive potential of tumor cells. Cancer Res. 1987. 47:3239–3245.
25. Craig WJ. Health-promoting properties of common herbs. Am J Clin Nutr. 1999. 70:suppl 3. S491–S499.
26. Guo Q, Zhao B, Shen S, Hou J, Hu J, Xin W. ESR study on the structure-antioxidant activity relationship of tea catechins and their epimers. Biochim Biophys Acta. 1999. 1427:13–23.
27. Bendeck MP, Conte M, Zhang M, Nili N, Strauss BH, Farwell SM. Doxycycline modulates smooth muscle cell growth, migration, and matrix remodeling after arterial injury. Am J Pathol. 2002. 160:1089–1095.
28. Taraboletti G, Morbidelli L, Donnini S, Parenti A, Granger HJ, Giavazzi R, Ziche M. The heparin binding 25 kDa fragment of thrombospondin-1 promotes angiogenesis and modulates gelatinase and TIMP-2 production in endothelial cells. FASEB J. 2000. 14:1674–1676.