Abstract
Background
The aim of this study is to evaluate the hepatic mechanism of fenofibrate that has the diabetes protective action in rats.
Methods
We chose OLETF rats and divided them into three groups. Fenofibrate (DF) group was fed with diet and fenofibrate (300 mg/kg/day). Paired feeding (Dd) group and free diet (DD) group were fed with diet. After 36 weeks of treatment, all the rats were sacrificed.
Results
The fasting blood glucose level of DF group (8.5 ± 0.9 mmol/L) showed normal. The fasting blood glucose level of Dd group (22.4 ± 3.0 mmol/L) and DD group (16.9 ± 3.7 mmol/L) showed significantly increased than that of DF group (P < 0.01, respectively). The body weight, visceral adipose tissue and subcutaneous adipose tissue of DF group were significantly decreased compared to those of Dd and DD groups (P <0.01, P <0.05, P <0.05). DF group showed significantly increased state-3 respiration rate, ATP synthetic activity, state-4 respiration rate and their blood β-keton body levels than those of control groups (P <0.01, respectively). DF group showed normal morphology of hepatocytes but DD and Dd groups showed hepatic steatosis with mitochondrial swellings.
Conclusion
Chronic fenofibrate treatment prevents the development of diabetes in OLETF rats with inhibiting gain of body weight and abdominal adiposity. The hepatic mechanism for reducing visceral adiposity is that fenofibrate leads to increasing oxidative phosphorylation, uncoupling and ketogenesis as well as increasing beta-oxidation of fatty acids. Moreover, fenofibrate treatment prevents the development of hepatic steatosis.
Figures and Tables
Fig. 1
The changes of body weight during the entire experiment. All groups showed the increasing tendency of body weight. The weight gain of fenofibrate group is significantly decreased than that of paired feed group and free diet group.
*P < 0.0001, versus paired feed group or free diet group, respectively.
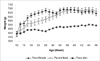
Fig. 2
Light microscopic findings of the liver in fenofibrate treated group and control groups. H&E stain, original magnification ×200.
A, The liver of DF group shows normal morphology. The hepatocytes have increased amount of eosinophilic cytoplasm. However, there is no evidence of hepatitis on entire sections of all cases; B, The liver of Dd group shows fatty change of the hepatocytes; C, The liver of DD group shows marked fatty change of the hepatocytes. This fatty change is severer than that of Dd group.
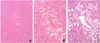
Fig. 3
Electron microscopical findings of the hepatocyte in DD and DF groups.
A, DD group. Most mitochondria (M) are swollen. Their cristae show varying degrees of disorientation, shortening and reduction in numbers. Lipid droplets (L) are large, and sometimes aggregated (two lipid droplets in the upper left corner). A close contact between the lipid droplet and mitochondria is demonstrated (arrows); B, Fenofibrate group. Most mitochondria appeared normal and only few small lipid droplets were scattered in the cytoplasm. (Bar, 2 µM. N: nucleus).
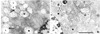
Fig. 4
Oxygram of isolated mitochondria from the liver in each group of OLETF rats.
In fenofibrate group, the oxygen consumption was sharply increased after addition of ADP (state-3 respiration) but this change was not observed in paired feeding and free diet groups. This meant that both group had marked deteriorations of ADP phosphorylation in their mitochondria. The state-3 respiration was terminated when oligomycin, the inhibitor of oxidative phosphorylation, was added. After that uncoupling process (the state-4 respiration) was induced. The state-4 respiration also decreased in both groups than in fenofibrate treated group.
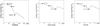
Fig. 5
The summary of possible hepatic mechanisms for prevention of diabetes by fenofibrate in OLETF rat.
Fenofibrate reduces fat burden on liver via increasing β-oxidation of fatty acid and promoting catabolism of its metabolites at multiple subsidiary sites, where oxidative phosphorylation, uncoupling and ketogenesis are increased. Fenofibrate also prevents hepatocyte injury, such as mitochondrial damage (ballooning) and steatosis. The changes of adipocytokines (adiponectin and leptin) induced by fenofibrate also do beneficial actions on fat burden and injury of the liver. (The findings in this study were presented as bold character) mit, mitochondria, BW, body weight
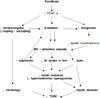
Table 1
The Comparison of Blood Glucose, Insulin, β-ketone Body Levels and HOMA IR Index between Fenofibrate, Paired Feeding and Free Diet Groups

Table 2
The Comparison of Body Weight, Fat Tissue Areas, Adiponectin, Leptin and FFA Levels between Fenofibrate, Paired Feeding and Free Diet Groups
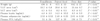
References
1. Roden M, Price TB, Perseghin G, Petersen KF, Rothman DL, Cline GW, Shulman GI. Mechanism of free fatty acid-induced insulin resistance in humans. J Clin Invest. 1996. 97:2859–2865.
2. Kelley DE, Goodpaster B, Wing RR, Simoneau JA. Skeletal muscle fatty acid metabolism in association with insulin resistance, obesity, and weight loss. Am J Physiol. 1999. 277:E1130–E1141.
3. Boden G, Chen X, Ruiz J, White JV, Rossetti L. Mechanisms of fatty acid-induced inhibition of glucose uptake. J Clin Invest. 1994. 93:2438–2446.
4. Tiikkainen Mirja, Tamminen Marjo, Hkkinen Anna-Maija, Bergholm Robert, Vehkavaara Satu, Halavaara Juha, Teramo Kari, Rissanen Aila, Yki-Jrvinen Hannele. Liver-Fat Accumulation and Insulin Resistance in Obese Women with Previous Gestational Diabetes. Obes Res. 2002. 10:859–867.
5. Chen MT, Kaufman LN, Spennetta T, Shrago E. High fat-feeding to rats on the interrelationship of body weight, plasma insulin, and fatty acyl-coenzyme A esters in liver and skeletal muscle. Metabolism. 1992. 41:564–569.
6. Lam TK, Yoshii H, Haber CA, Bogdanovic E, Lam L, Fantus IG, Giacca A. Free fatty acid-induced hepatic insulin resistance: a potential role for protein kinase C-delta. Am J Physiol Endocrinol Metab. 2002. 283:E682–E691.
7. Petersen KF, Dufour S, Befroy D, Garcia R, Shulman GI. Impaired mitochondrial activity in the insulin-resistant offspring of patients with type 2 diabetes. N Engl J Med. 2004. 350:664–671.
8. Kelley DE, He J, Menshikova EV, Ritov VB. Dysfunction of mitochondria in human skeletal muscle in type 2 diabetes. Diabetes. 2002. 51:2944–2950.
9. Schrauwen P, Hesselink MK, Blaak EE, Borghouts LB, Schaart G, Saris WH, Keizer HA. Uncoupling protein 3 content is decreased in skeletal muscle of patients with type 2diabetes. Diabetes. 2001. 50:2870–2873.
10. Schrauwen P, Xia J, Bogardus C, Pratley RE, Ravussin E. Skeletal muscle uncoupling protein 3 expression is a determinant of energy expenditure in Pima Indians. Diabetes. 1999. 48:146–149.
11. Schrauwen P, Hesselink MK. Oxidative capacity, lipotoxicity, and mitochondrial damage in type 2 diabetes. Diabetes. 2004. 53:1412–1417.
12. Guerre-Millo M, Gervois P, Raspe E, Madsen L, Poulain P, Derudas B, Herbert JM, Winegar DA, Willson TM, Fruchart JC, Berge RK, Staels B. Peroxisome Proliferator-activated Receptor Activators Improve Insulin Sensitivity and Reduce Adiposity. J Biol Chem. 2002. 275:16638–16642.
13. Lee HJ, Choi SS, Park MK, An YJ, Seo SY, Kim MC, Hong SH, Hwang TH, Kang DY, Garber AJ, Kim DK. Fenofibrate lowers abdominal andskeletal adiposity and improves insulin sensitivity in OLETF rats. Biochem Biophys Res Commun. 2002. 296:293–299.
14. Koh EH, Kim MS, Park JY, Kim HS, Youn JY, Park HS, Youn JH, Lee KU. Peroxisome proliferator-activated receptor (PPAR)-alpha activation prevents diabetes in OLETF rats: comparison with PPAR-gamma activation. Diabetes. 2003. 52:2331–2337.
15. Lanni A, Mancin IF, Sabatino L. De novo expression of uncoupling protein 3 is associated to enhanced mitochondrial thioesterase expression and fatty acid metabolism in liver of fenofibrate-treated rats. FEBS Letters. 2002. 525:7–12.
16. Matthews DR, Hosker JP, Rudenski AS, Naylor BA, Treacher DF, Turner RC. Homeostasis model assessment: insulin resistance and cell function from fasting plasma glucose and insulin concentrations in man. Diabetologia. 1985. 28:412–419.
17. Tomas E, Tsao TS, Saha AK, Murrey HE, Zhang Cc C, Itani SI, Lodish HF, Ruderman NB. Enhanced muscle fat oxidation and glucose transport by ACRP30 globular domain: acetyl-CoA carboxylase inhibition and AMP-activated protein kinase activation. Proc Natl Acad Sci U S A. 2002. 99:16309–16313.
18. Guo Q, Wang PR, Milot DP, Ippolito MC, Hernandez M, Burton CA, Wright SD, Chao Y. Regulation of lipid metabolism and gene expression by fenofibrate in hamsters. Biochim Biophys Acta. 2001. 1533:220–232.
19. Bacha F, Saad R, Gungor N, Arslanian SA. Adiponectin in youth: relationship to visceral adiposity, insulin sensitivity, and beta-cell function. Diabetes Care. 2004. 27:547–552.
20. Hotta K, Funahashi T, Arita Y, Takahashi M, Matsuda M, Okamoto Y, Iwahashi H, Kuriyama H, Ouchi N, Maeda K, Nishida M, Kihara S, Sakai N, Nakajima T, Hasegawa K, Muraguchi M, Ohmoto Y, Nakamura T, Yamashita S, Hanafusa T, Matsuzawa Y. Plasma concentrations of a novel, adipose-specific protein, adiponectin, in type 2 diabetic patients. Arterioscler Thromb Vasc Biol. 2000. 20:1595–1599.
21. Cornwell PD, De Souza AT, Ulrich RG. Profiling of hepatic gene expression in rats treated with fibric acid analogs. Mutat Res. 2004. 549:131–145.
22. Acin A, Rodriguez M, Rique H, Canet E, Boutin JA, Galizzi JP. Cloning and characterization of the 5' flanking region of the human uncoupling protein 3 (UCP3) gene. Biochem Biophys Res Commun. 1999. 258:278–283.
23. Brun S, Carmona MC, Mampel T, Vinas O, Giralt M, Iglesias R, Villarroya F. Activators of peroxisome proliferator-activated receptor-alpha induce the expression of the uncoupling protein-3 gene in skeletal muscle: a potential mechanism for the lipid intake-dependent activation of uncoupling protein-3 gene expression at birth. Diabetes. 1999. 48:1217–1222.
24. Yamamoto K, Fukuda N, Zhang L, Sakai T. Altered heptic metabolism of fatty acids in rats fed a hypolipidaemic drug, fenofibrate. Pharmacological research. 1996. 33:337–342.
25. Larsen PJ, Jensen PB, Sorensen RV, Larsen LK, Vrang N, Wulff EM, Wassermann K. Differential Influences of Peroxisome ProliferatorActivated Receptors gamma and alpha on food Intake and energy homeostasis. Diabetes. 2003. 52:2249–2259.
26. Kyosuke Y, Nobuhiro F, Zhang L, Takahiro S. Altered heptic metabolism of fatty acids in rats fed a hypolipidaemic drug, fenofibrate. Pharmacological research. 1996. 33:337–342.
27. Larsen PJ, Jensen PB, Sørensen RV, Larsen LK, Vrang N, Wulff EM, Wassermann K. Differential Influences of Peroxisome ProliferatorActivated Receptors γ and -α on food Intake and energy homeostasis. Diabetes. 2003. 52:2249–2259.
28. Meertens LM, Miyata KS, Cechetto JD, Rachubinski RA, Capone JP. A mitochondrial ketogenic enzyme regulates its gene expression by association with the nuclear hormone receptor PPARα. EMBO J. 1998. 17:6972–6978.
29. Arase K, Fisler JS, Shargill NS, York DA, Bray GA. Intracerebroventricular infusions of 3-OHB and insulin in a rat model of dietary obesity. Am J Physiol. 1988. 255:R974–R981.
30. Sherlock S. Patterns of hepatocyte injury in man. Lancet. 1982. 1:782–786.
31. Hall JC, Sordahl LA, Stefko PL. The effect of insulin on oxidative phosphorylation in normal and diabetic mitochondria. J Biol Chem. 1960. 235:1536–1539.
32. Ghadially FN. Ultrastructual pathology of the cell and matrix. 1988. London: Butterworths;240–249.
33. Marchesini G, Brizi M, Bianchi G, Tomassetti S, Bugianesi E, Lenzi M, McCullough AJ, Natale S, Forlani G, Melchionda N. Nonalcoholic fatty liver disease: a feature of the metabolic syndrome. Diabetes. 2001. 50:1844–1850.
34. Browning JD, Horton JD. Molecular mediators of hepatic steatosis and liver injury. J Clin Invest. 2004. 114:147–152.
35. Seppala-Lindroos A, Vehkavaara S, Hakkinen AM, Goto T, Westerbacka J, Sovijarvi A, Halavaara J, Yki-Jarvinen H. Fat accumulation in the live is associated with defects in insulin suppression of glucose production and serum free fatty acids independent of obesity in normal men. J Clin Endocrinol Metab. 2002. 87:3023–3028.
36. Ryysy L, Hakkinen AM, Goto T, Vehkavaara S, Westerbacka J, Halavaara J, Yki-Jarvinen H. Hepatic fat content and insulin action on free fatty acids and glucose metabolism rather than insulin absorption are associated with insulin requirements during insulin therapy in type 2 diabetic patients. Diabetes. 2002. 49:749–758.
37. Bhargava R, Senior PA, Ackerman TE, Ryan EA, Paty BW, Lakey JR, Shapiro AM. Prevalence of hepatic steatosis after islet transplantation and its relation to graft function. Diabetes. 2004. 53:1311–1317.
38. Weyer C, Funahashi T, Tanaka S, Hotta K, Matsuzawa Y, Pratley RE, Tataranni PA. Hypoadiponectinemia in obesity and type 2 diabetes: close association with insulin resistance and hyperinsulinemia. J Clin Endocrinol Metab. 2001. 86:1930–1935.
39. Silha JV, Krsek M, Skrha JV, Sucharda P, Nyomba BL, Murphy LJ. Plasma resistin, adiponectin and leptin levels in lean and obese subjects: correlations with insulin resistance. Eur J Endocrinol. 2003. 149:331–335.
40. Chitturi S, Farrell G, Frost L, Kriketos A, Lin R, Fung C, Liddle C, Samarasinghe D, George J. Serum leptin in NASH correlates with hepatic steatosis but not fibrosis: a manifestation of lipotoxicity? Hepatolog. 2002. 36:403–409.
41. Chalasani N, Crabb DW, Cummings OW, Kwo PY, Asghar A, Pandya PK. Does leptin play a role in the pathogenesis of human nonalcoholic steatohepatitis. Am J Gastroenterol. 2003. 98:2771–2776.
42. Hays T, Rusyn I, Burns A, Kennett M, Ward J, Gonzalez F, Peters J. Role of peroxisome proliferator-activated receptor-α (PPARα) in bezafibrate-induced hepatocarcinogenesis and cholestasis. Carcinogenesis. 2005. 26:219–227.
43. Chevalier S, Roberts R. Perturbation of rodent hepatocyte growth control by nongenotoxic hepatocarcinogens: mechanisms and lack of relevance for human health. Oncol Rep. 1988. 5:1319–1327.