Abstract
Background
Glucolipotoxicity plays an important role in the progression of type 2 diabetes mellitus via inducing insulin secretory dysfunction. Expression of insulin gene in pancreatic beta cell might be regulated by AMP-activated protein kinase (AMPK), which is recognized as a key molecule of energy metabolism. We studied the effects of AMPK on glucolipotoxicity-induced β-cell dysfunction by suppression of PPAR-γ-coactivator-1 (PGC-1) in vitro and in vivo.
Method
Glucolipotoxicity was induced by 33.3 mM glucose and 0.6 mM (palmitate and oleate) for 3 days in isolated rat islets. Messenger RNA (mRNA) expressions of β-cell specific gene like insulin, BETA2/NeuroD and PGC-1 induced by glucolipotoxic condition and their changes with 5-aminoimidazole-4-carboxy-amide-1-D-ribofuranoside (AICAR) treatment were investigated using RT-PCR. We also examined glucose stimulated insulin secretion in same conditions. Furthermore, SD rats were submitted to a 90% partial pancreatectomy (Px) and randomized into two groups; Ad-GFP-infected Px rats (n = 3) and Ad-siPGC-1-infected Px rats (n = 3). Then, the Px rats were infected with Ad-GFP or Ad-siPGC-1 (1 × 109 pfu) via celiac artery. After 12 days of viral infection, we measured body weight and performed the intraperitoneal glucose tolerance test (IP-GTT).
Results
Glucolipotoxicity resulted in blunting of glucose-stimulated insulin secretion, which was recovered by the AICAR treatment in vitro. Suppression in their expressions of insulin and BETA2/NeuroD gene by glucolipotoxic condition were improved with AICAR treatment. However, PGC-1α expression was gradually increased by glucolipotoxicity, and suppressed by AICAR treatment. Overexpression of PGC-1 using an adenoviral vector in freshly isolated rat islets suppressed insulin gene expression. We also confirmed the function of PGC-1 using an Ad-siPGC-1 in vivo. Direct infection of Ad-siPGC-1 in 90% pancreatectomized rats significantly improved glucose tolerance and increased body weight.
Figures and Tables
Fig. 1
Change of insulin secretion by glucolipotoxicity and chronic AICAR treatment. Islets were isolated and subsequently cultured at the indicated glucolipotoxicity with or without AICAR treatment, prior to assay of insulin secretion. After incubation with each condition for 3 days, Cultured islets were washed in KRB washing buffer and incubated in KRB buffer containing 5.5 mM glucose for 1 h. And then, islets were stimulated for 1 h in KRB buffer containing 17.7 mM glucose. Results are expressed as means S.D. for five independent experiments (*P < 0.05).
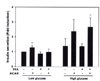
Fig. 2
Change of insulin gene expression by glucolipotoxicity and chronic AICAR treatment. A, islets were incubated with or without 0.6 mM FFA (a mixture of palmitate and oleate) in the presence of a high concentration of glucose (33.3 mM) for 3 days. Induction of glucolipotoxicity was confirmed by measuring insulin mRNA level using RT-PCR; B, The bar graphs show quantification of the results of PCR of rat islet extracts. Insulin gene expression was suppressed in a time-dependent manner and was notably increased by AICAR treatment at 3 day. Results are expressed as means S.D. for five independent experiments (*P < 0.05).
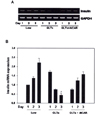
Fig. 3
Expressions of beta-cell specific gene by glucolipotoxicity and chronic AICAR treatment. A, rat islets were cultured in suspension for three days in 5 mM or 33.3 mM glucose and 0.6 mM FFA or in the presence of 400 µM AICAR. Insulin, BETA2 and PGC-1 were estimated by semi-quantitative RT-PCR; B, The bar graphs show quantification of the results of PCR of rat islet extracts. Expression of glucose-induced insulin and BETA2 genes were repressed by glucolipotoxicity but rescued by AICAR treatment. However, PGC-1 The mRNA levels of these genes were normalized to GAPDH mRNA, and the RT-PCR data from the three independent experiments were summarized as means standard errors relative to those of untreated islets (*P < 0.05, **P < 0.01).
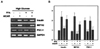
Fig. 4
Adenovirus-mediated expression of PGC-1 reduces expression of the insulin gene. A, After infection with the indicated adenovirus at 100 MOI and culture for 24 h at 11 mM glucose, isolated rat islets were infected with PGC-1-expressing adenovirus. After infection with the indicated adenovirus 100 MOI for 2 h, islets were cultured at low or high glucose levels with or without FFA, and/or AICAR treatment for 72 h. Overexpression of PGC-1 induced by the glucolipotoxic condition was clearly suppressed by AICAR co-treatment and the expression of the insulin gene was normalized. In contrast, overexpression of PGC-1 induced by the adenoviral vector was not suppressed by AICAR treatment. Results are expressed as means S.D. for five independent experiments (*P < 0.05).
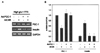
Fig. 5
Body weight and blood glucose change during the 12-day study period. Hyperglycemic Px rats were infected with a celiac artery of Ad-GFP or Ad-siPGC-1 (1 × 109 pfu/300 µL, n = 3) for 12 days. The morning nonfasting glucose values of the Ad-siPGC-1 group were significantly lower than those of the Ad-GFP group 6 days after Ad-siPGC-1 infection. A, Body weight did not differ between Ad-GFP and Ad-siPGC-1-infected Px rats; B, IPGTT was performed using a 20% glucose solution and a dose rate of 2 g/kg. The mean AUCg was elevated in the AICAR-treated Px rats. Data are expressed as means S.D., n = 6 (*P < 0.05). (*P < 0.05, **P < 0.01); C and D, PGC-1 was expressed in islets of pancreas tissue of Ad-GFP-infected (C) Px rat but PGC-1 was remarkably decreased in islet of Ad-siPGC-1-infected Px rat (D). (400X).
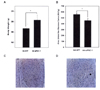
References
2. Roche E, Maestre I, Martin F, Fuentes E, Casero J, Reig JA, Soria B. Nutrient toxicity in pancreatic beta-cell dysfunction. J Physiol Biochem. 2000. 56(2):119–128.
3. Prentki M, Joly E, El-Assaad W, Roduit R. Malonyl-CoA signaling, lipid partitioning, and glucolipotoxicity: role in beta-cell adaptation and failure in the etiology of diabetes. Diabetes. 2002. 51:Suppl 3. S405–SS13.
4. Pick A, Clark J, Kubstrup C, Levisetti M, Pugh W, Bonner-Weir S, Polonsky K. Role of apoptosis in failure of β-cell mass compensation for insulin resistance and β-cell defects in the male Zucker diabetes fatty rat. Diabetes. 1998. 47:358–364.
5. Cnop M, Hannaert JC, Hoorens A, Eizirik DL, Pipeleers DG. Inverse relationship between cytotoxicity of free fatty acids in pancreatic islet cells and cellular triglyceride accumulation. Diabetes. 2001. 50:1771–1777.
6. Rutter GA, da SilvaXavier G, Leclerc I. Roles of 5'-AMP-activated protein kinase(AMPK) in mammalian glucose homoeostasis. Biochem J. 2003. 375:1–16.
7. Kemp BE, Mitchelhill KI, Stapleton D, Michell BJ, Chen ZP, Witters LA. Dealing with energy demand: the AMP-activated protein kinase. Trends Biochem Sci. 1999. 24(1):22–25.
8. Hardie DG. The AMP-activated protein kinase pathway-new players upstream and downstream. J Cell Sci. 2004. 11723(Pt 23):5479–5487.
9. Salt IP, Johnson G, Ashcroft SJ, Hardie DG. AMP-activated protein kinase is activated by low glucose in cell lines derived from pancreatic beta cells, and may regulate insulin release. Biochem J. 1998. 335:533–539.
10. da SilvaXavier G, Leclerc I, Varadi A, Tsuboi T, Moule SK, Rutter GA. Role for AMP-activated protein kinase in glucose-stimulated insulin secretion and preproinsulin gene expression. Biochem J. 2003. 371:761–774.
11. Diraison F, Motakis E, Parton LE, Nason GP, Leclerc I, Rutter GA. Impact of adenoviral transduction with SREBP1c or AMPK on pancreatic islet gene expression profile: analysis with oligonucleotide microarrays. Diabetes 53. 2004. 53:Suppl 3. S84–S91.
12. Leclerc I, Woltersdorf WW, Da Silva Xavier G, Rowe RL, Cross SE, Korbutt GS, Rajotte RV, Smith R, Rutter GA. Metformin, but not leptin, regulates AMP-activated protein kinase in pancreatic islets: impact on glucose-stimulated insulin secretion. Am J Physiol Endocrinol Metab. 2004. 286:E1023–E1031.
13. Pold R, Jensen LS, Jessen N, Buhl ES, Schmitz O, Flyvbjerg A, Fujii N, Goodyear LJ, Gotfredsen CF, Brand CL, Lund S. Long-term AICAR administration and exercise prevents diabetes in ZDF rats. Diabetes. 2005. 54(4):928–934.
14. Diraison F, Parton L, Ferre P, Foufelle F, Briscoe CP, Leclerc I, Rutter GA. Over-expression of sterol-regulatory-element-binding protein-1c(SREBP1c) in rat pancreatic islets induces lipogenesis and decreases glucose-stimulated insulin release: modulation by 5-aminoimidazole-4-carboxamide ribonucleoside(AICAR). Biochem J. 2004. 378:769–778.
15. Puigserver P, Spiegelman BM. Peroxisome proliferator-activated receptor-gamma coactivator 1 alpha(PGC-1 alpha): transcriptional coactivator and metabolic regulator. Endocr Rev. 2003. 24:78–90.
16. Yoon JC, Xu G, Deeney JT, Yang SN, Rhee J, Puigserver P, Levens AR, Yang R, Zhang CY, Lowell BB, Berggren PO, Newgard CB, Bonner-Weir S, Weir G, Spiegelman BM. Suppression of beta cell energy metabolism and insulin release by PGC-1alpha. Dev Cell. 2003. 5:73–83.
17. Bonner-Weir S, Trent DF, Weir GC. Partial pancreatectomy in the rat and subsequent defect in glucose-induced insulin release. J Clin Invest. 1983. 71:1544–1553.
18. Ko SH, Suh SH, Kim BJ, Ahn YB, Song KH, Yoo SJ, Son HS, Cha BY, Lee KW, Son HY, Kang SK, Bonner-Weir S, Weir GC, Yoon KH, Park CG. Expression of the intermediate filament vimentin in proliferating duct cells as a marker of pancreatic precursor cells. Pancreas. 2004. 228(2):121–128.
19. Zhou G, Myers R, Li Y, Chen Y, Shen X, Fenyk-Melody J, Wu M, Ventre J, Doebber T, Fujii N, Musi N, Hirshman MF, Goodyear LJ, Moller DE. Role of AMP-activated protein kinase in mechanism of metformin action. J Clin Invest. 2001. 108:1167–1174.
20. Kefas BA, Cai Y, Kerckhofs K, Ling Z, Martens G, Heimberg H, Pipeleers D, Van de Casteele M. Metformin-induced stimulation of AMP-activated protein kinase in beta-cells impairs their glucose responsiveness and can lead to apoptosis. Biochem Pharmacol. 2004. 68:409–416.
21. Fiedler M, Zierath JR, Selen G, Wallberg-Henriksson H, Liang Y, Sakariassen KS. 5-aminoimidazole-4-carboxy-amide-1-beta-D-ribofuranoside treatment ameliorates hyperglycaemia and hyperinsulinaemia but not dyslipidaemia in KKAy-CETP mice. Diabetologia. 2001. 44:2180–2186.
22. Halseth AE, Ensor NJ, White TA, Ross SA, Gulve EA. Acute and chronic treatment of ob/ob and db/db mice with AICAR decreases blood glucose concentrations. Biochem Biophys Res Commun. 2002. 294:798–805.
23. Song XM, Fiedler M, Galuska D, Ryder JW, Fernstrom M, Chibalin AV, Wallberg-Henriksson H, Zierath JR. 5-Aminoimidazole-4-carboxamide ribonucleoside treatment improves glucose homeostasis in insulin-resistant diabetic(ob/ob) mice. Diabetologia. 2002. 45(1):56–65.
24. Puigserver P, Wu Z, Park CW, Graves R, Wright M, Spiegelman BM. A cold-inducible coactivator of nuclear receptors linked to adaptive thermogenesis. Cell. 1998. 92:829–839.
25. Knutti D, Kaul A, Kralli A. A tissue-specific coactivator of steroid receptors, identified in a functional genetic screen. Mol Cell Biol. 2000. 20:2411–2422.
26. Delerive P, Wu Y, Burris TP, Chin WW, Suen CS. PGC-1 functions as a transcriptional coactivator for the retinoid X receptors. J Biol Chem. 2002. 277:3913–3917.
27. Tcherepanova I, Puigserver P, Norris JD, Spiegelman BM, McDonnell DP. Modulation of estrogen receptor-alpha transcriptional activity by the coactivator PGC-1. J Biol Chem. 2000. 275:16302–16308.
28. Yoon JC, Puigserver P, Chen G, Donovan J, Wu Z, Rhee J, Adelmant G, Stafford J, Kahn CR, Granner DK, Newgard CB, Spiegelman BM. Control of hepatic gluconeogenesis through the transcriptional coactivator PGC-1. Nature. 2001. 413:131–138.