Abstract
Background
Human metapneumovirus (hMPV) and respiratory syncytial virus (RSV) share some epidemiological and clinical characteristics; however, few studies have examined the mechanisms by which these viruses induce airway inflammation.
Objective
This study was undertaken to compare cytokine profiles in hMPV and RSV patients to investigate possible differences in inflammatory pathways.
Methods
Nasopharyngeal aspirate specimens were collected from 1,008 pediatric patients hospitalized for acute lower respiratory tract infection with wheezing and 20 normal healthy controls. Patients were tested for 7 common respiratory viruses then divided into hMPV (n = 35) and RSV groups (n = 67). T helper (Th) 1 (interferon [IFN]-γ), Th2 (interleukin [IL]-4, eotaxin) and Th17 (IL-1β, IL-6) cytokine profiles were analyzed in the 3 groups.
Results
IFN-γ and IL-2 levels were significantly increased in the hMPV and RSV groups compared to the control group (p < 0.0001 and p < 0.0001, respectively). IL-4 levels were significantly higher in the RSV group compared to the hMPV and control groups (p = 0.0003 and p < 0.0001, respectively). Eotaxin levels showed a tendency to be higher in the RSV group compared to the hMPV group (p = 0.0580), and significantly higher compared to the control group (p < 0.0001). IL-1β levels were significantly higher in the hMPV compared to the RSV group (p < 0.0001), and IL-6 levels were significantly higher in the hMPV group compared to the control group (p < 0.0001).
Human metapneumovirus (hMPV) is a recently discovered Paramyxoviridae, which causes infection in the upper or lower airway and induces respiratory symptoms such as coughing and wheezing [12]. Respiratory syncytial virus (RSV) induces acute wheezing and is well known as a representative and causative virus of bronchiolitis. It may also lead to asthma thereafter [34].
Clinical and epidemiological similarities have been noted [5]. They both cause bronchiolitis and reactive airway disease, and exacerbate bronchial asthma resulting in wheezing. However, there are differences between the 2 viruses. Those infected with hMPV are older than those infected with RSV. In addition, the incidence of RSV infection is highest in winter, whereas hMPV is prevalent mainly in spring. In addition, blood eosinophil levels were higher in the RSV group compared to the hMPV group [5].
Airway inflammatory reactions caused by viral infection may have a different mechanism depending on the causative virus, and studies on such mechanisms and associations related to the progression of asthma are in progress. Regarding the association with asthma, there have been many studies performed on RSV to date, but in the case of hMPV, which was first discovered in 2001, few studies have examined the mechanisms by which this virus induces airway inflammation in spite of its epidemiological and clinical importance [6].
This study aimed to compare nasal cytokine profiles in hMPV and RSV patients to investigate their inflammatory pathways.
Nasopharyngeal aspirate specimens was collected from 1,008 pediatric patients hospitalized with acute respiratory tract infection with wheezing at Inje University Sanggye Paik Hospital from December 2003 to April 2008 and tested for 7 common respiratory viruses. Conditions classified as wheezing illness were bronchiolitis, reactive airways disease (RAD), and bronchial asthma [7]. Acute bronchiolitis was defined as a first occurrence of expiratory wheezing with or without tachypnea, air trapping, and substernal retraction in children <2 years of age. RAD was defined as recurrent wheezing <3 times, and bronchial asthma was defined as recurrent wheezing ≥3 times in any child.
The control group included 20 pediatric patients in the same age range who did not have any respiratory symptoms at the time of the study and had no past history of allergic diseases at the time of visiting hospital for their pediatric health examination and vaccination.
Collection of nasopharyngeal aspirates was conducted immediately after hospitalization. A disposable catheter was inserted into the nose to aspirate the nasal fluid, and 2 mL of saline solution was injected into the tube for collection. Collected specimens were centrifuged and suspended matter in the supernatant layer was retrieved and stored at -70℃ until analysis.
The study was approved by Inje University Sanggye Paik Hospital's Institutional Review Board (approval number: IJ 06-34) and written permission was obtained from all patient's parents/caregivers.
Seven common types of respiratory viruses (adenovirus, RSV, influenza A, influenza B, and parainfluenza 1–3) were detected through the immunofluorescent-antibody test (IFA) (DAKO, Cambridgeshire, UK) from nasopharyngeal aspirate specimens collected between December 2003 and December 2006. From January 2007 to study completion, 7 kinds of respiratory viruses (adenovirus, RSV, influenza A, influenza B, parainfluenza 1–3) were detected through reverse transcriptase-polymerase chain reaction (RT-PCR) (Seegene Inc., Seoul, Korea). hMPV was detected separately through the multiplex RT-PCR.
Cytokines interferon (IFN)-γ, interleukin (IL)-1β, IL-4, IL-6, IL-13 and chemokine eotaxin were measured by amplifying to a 20-fold concentration using a commercial enzyme-linked immunosorbent assay (Endogen, Woburn, MA, USA), with a minimum detection limit of 2 pg/mL. All analyses were conducted twice for quality purposes.
Sex differences between the 3 groups were compared using a chi-square test. Cytokine levels in nasal fluid were expressed per milliliter. Because most of the data were not normally distributed, differences between the 3 subject groups were screened with the nonparametric Kruskal-Wallis test. When this test indicated a significant difference, the difference between the 2 groups was tested for with the Mann-Whitney U-test. Correlations were analyzed by the nonparametric Spearman correlation test. All normally distributed data were expressed as mean ± standard deviation, while nonnormally distributed data were expressed as median. All analyses were performed using SAS Deployment Wizard ver. 9.3 (SAS Institute Inc., Cary, NC, USA), and a p value of less than 0.05 was considered significant.
Out of the 1,008 pediatric patients who underwent viral testing, 35 subjects were hMPV positive (hMPV group) and 67 were RSV positive (RSV group). Twenty normal healthy controls (control group) were used for comparison. Of these virus-positive cases, 46.2% of HMPV-infected and 16.9% of RSV-infected patients were classified as asthma exacerbation (data not shown). There were no significant differences in age and gender among the 3 groups, and there were also no significant differences in white blood cell counts or blood eosinophil fraction ratio between the hMPV and RSV groups (Table 1).
The representative T helper (Th) 1 cytokine, IFN-γ, was significantly higher in both the hMPV (mean ± standard deviation; 11.9 ± 23.3 pg/mL) and RSV group (12.6 ± 15.8 pg/mL) compared to the control group (0.3 ± 0.7 pg/mL) (p < 0.0001 and p < 0.0001, respectively), but there were no significant differences between the 2 groups (p = 0.0874) (Fig. 1A). IL-2 was significantly higher in both the hMPV (87.9 ± 114.3 pg/mL) and RSV group (14.0 ± 16.6 pg/mL) compared to the control group (3.3 ± 8.2 pg/mL) (p < 0.0001 and p < 0.0001, respectively), and IL-2 levels were higher in the hMPV group compared to the RSV group (p < 0.0001) (Fig. 1B).
The representative Th2 cytokine, IL-4, was measured under the reference value in many cases (the value under the reference value was defined as the sensitivity value), but analysis showed it was significantly higher in the RSV group (1.5 ± 2.4 pg/mL) compared to the hMPV (0.7±0.7 pg/mL) and control group (0.0 ± 0.0 pg/mL) (p = 0.0003 and p < 0.0001, respectively) (Fig. 2A). Th2 eosinophilic chemokine, eotaxin, levels also tended to be higher in the RSV group (14.7 ± 18.7 pg/mL) compared to the hMPV group (18.5 ± 25.2 pg/mL) (p = 0.0580), and both groups had significantly higher levels than the control group (0.5 ± 1.1 pg/mL) (p < 0.0001 and p < 0.0001, respectively) (Fig. 2B).
Meanwhile, levels of the Th17 cytokine, IL-1β, were significantly higher in the hMPV group (745.0 ± 558.3 pg/mL) compared to the RSV (184.8 ± 145.1 pg/mL) and control group (36.8 ± 80.6 pg/mL) (p < 0.0001 and p < 0.0001, respectively) (Fig 3A). IL-6 levels were significantly higher in hMPV (844.9 ± 1797.6 pg/mL) and RSV groups (567.6 ± 772.3 pg/mL) compared to the control group (6.8 ± 11.9 pg/mL) (p < 0.0001 and p < 0.0001, respectively) (Fig. 3B)
We investigated mechanisms of airway inflammation due to respiratory virus infections by comparing nasal cytokine profiles. Each mechanism with respect to Th1, Th2, and Th17 cytokines in patients infected with RSV and hMPV were studied. New findings included: Th1 mechanism involvement in both the RSV and hMPV groups resuled in higher levels when compared to the control group. In addition, more dominant Th2 mechanisms were shown in the RSV infection group through increases of IL-4 and eotaxin levels, while more dominant Th17 mechanisms of airway inflammation were demonstrated in the hMPV infection group through increases of IL-1β and IL-6.
The primitive T cell differentiates into Th1, Th2, Th17, and regulatory T cells according to the body's needs and for each mechanism inflammatory markers, such as IFN-γ, IL-4, IL-5, IL-17, and IL-10, act as important mediating cytokines. In addition to the Th2 mechanism, which is well known in allergic diseases including bronchial asthma, the Th17 mechanism has also been found to play an important role in asthma as it increases the bronchial sensitivity. In particular, it was reported that Th17 is involved in neutrophil-mediated asthma that presents as severe irreversible airway obstruction [891011]. According to a recent study, when eosinophils are activated via the Th17 pathway, expression of IL-1β increases and ultimately IL-17 releases freely from CD4+ T cells increasing in level, as well [12]. Therefore, the Th17-based airway inflammation mechanism in patients infected by hMPV in this study may be an important outcome that could explain the potential of progression to post-HMPV infection asthma in addition to results from previous studies.
IL-1β, along with IL-18, is also involved in inflammasomes, protein complexes of the innate immune response that can be activated by viral infection [13]. Upregulation of associated genes in hMPV-infected children were recently found in a study by Malmo et al. [14], with greater upregulation demonstrated in children with more severe disease. It is important to note that the patients in our study all experienced infection severe enough to be hospitalized, just as in Malmo's study. Laham et al. [15] presented in their study on human nasal lavage fluid from patients with upper and lower airway infections that all cytokines, not only IL-1β and IL-6, are important in the Th17 pathway. Not only were these cytokine levels higher in the RSV group than in the hMPV group, but also IL-8, IL-10, IL-12, and TNF-α. However, our study involved inpatients with severe lower airway infections, and IL-1β and IL-6 levels were higher in the hMPV group, unlike in study of Laham et al. [15]. hMPV infection in mice has been shown to induce increased levels of IL-6 compared to those infected with RSV [16]. A study by Guerrero-Plata et al. [17] found human dendritic cells exposed to hMPV and RSV produced increased levels of IL-6 and IL-1B. This also implies that the airway inflammatory reaction to the virus depends on severity and the Th17 mechanism may play an important role in it.
In a laboratory study in rats conducted by Guerrero-Plata et al. [1718], proinflammatory cytokines IL-1α, IL-1β, IL-6, and TNF-α; regulatory cytokine IL-10; and chemokines CCL-3 macrophage inflammatory protein-1alpha and CCL-5 RANTES (regulated on activation, normal T cell expressed and secreted) were higher in the bronchoalveolar lavage fluid of rats infected by RSV than in those infected by hMPV. This finding implies that inflammatory mechanisms in animal models are different from the human subjects in our study.
Among recent hypotheses that describe the onset and aggravation of asthma, studies on the association of the Th17 mechanism have been reported. Neutrophils and IL-17 have been found in lung tissue in patients with severe asthma or steroid-resistant asthma and their involvement in bronchial fibrosis has also been reported [192021]. Memory CD4+ T cells produce both IL-4 and IL-17A and as the inflammation occurs repeatedly, it can be converted from the Th2 to the Th17 pathway [22]. Eosinophils increase the production of IL-17 in CD4+ T cells and also increase the expression of IL-17 mRNA by freeing IL-1β [12]. Thus, as this study shows, IL-1β increases IL-17 and activates the Th17 mechanism, demonstrating the possibility that IL-1β can cause progression to bronchial asthma in the hMPV group. The above findings show that it is clearly distinguishable from the Th2 mechanism that creates asthma progression through increase of IL-4 and eotaxin in the RSV group.
It has been demonstrated many times that the incidence of Th2 type immunodeviation results from the onset of asthma, and the results of our study were similar to those of previous studies [23]. In a previous study by our research group, analysis of inflammatory cells in bronchoalveolar lavage fluid found elevated neutrophil levels in RSV-induced bronchiolitis patients. However, in patients with an allergy, eosinophil levels also rose [24]. When patients were divided into eosinophil positive and eosinophil negative subgroups, only Th2 cytokine IL-5 levels were elevated. Thus, it was reported that during RSV infection, Th2 cytokines acted strongly in some patients, which led to eosinophilic inflammation [2526]. Since this study was conducted only in severely ill and hospitalized patients, there was a high likelihood that allergy contributed to disease severity. Therefore, it cannot be ruled out that the eosinophil positive subgroup of the severe RSV infection group might have driven the entire study's results (i.e., the increase in eotaxin).
As for study limitations, nasopharyngeal aspirate specimens were easy to collect but were likely an insufficient reflection of lower airway inflammation. Pringle et al. [27] compared the culture broths of upper and lower airway cells collected from patients infected by hMPV or RSV and reported contradictory results (e.g., varying levels of IFN), suggesting that inflammatory reactions of the upper and lower airways may not be the same. However, McDougall et al. [28] reported that inflammatory mediators of nasal epithelial and bronchial epithelial cells were released at nearly the same extent. The number of enrolled subjects was relatively small, so further study with a much larger sample population is needed in the future. The final limitation of our study was the use of 2 different virus detection methods (i.e., IFA and RT-PCR). However, we feel that the gap in accuracy between them is minor and should not significantly affect the results.
Our study demonstrates that despite having similar epidemio-logical and clinical characteristics, RSV and hMPV infections result in different inflammatory mechanisms: RSV acts through the Th2 pathway, whereas hMPV acts through the Th17 pathway by releasing IL-1β and IL-6.
Figures and Tables
Fig. 1
Comparison of T helper 1 cytokine interferon (IFN)-γ (A) and interleukin (IL)-2 (B) in nasal secretions of patients infected with either human metapneumovirus (hMPV) (n = 35) or respiratory syncytial virus (RSV) (n = 67), and normal controls (n = 20).
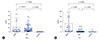
Fig. 2
Comparison of T helper 2 cytokine interleukin (IL)-4 (A) and chemokine eotaxin (B) in nasal secretions of patients infected with either human metapneumovirus (hMPV) (n = 35) or respiratory syncytial virus (RSV) (n = 67), and normal controls (n = 20).
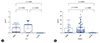
References
1. Peret TC, Boivin G, Li Y, Couillard M, Humphrey C, Osterhaus AD, Erdman DD, Anderson LJ. Characterization of human metapneumoviruses isolated from patients in North America. J Infect Dis. 2002; 185:1660–1663.


2. Jartti T, van den Hoogen B, Garofalo RP, Osterhaus AD, Ruuskanen O. Metapneumovirus and acute wheezing in children. Lancet. 2002; 360:1393–1394.


3. Glezen P, Denny FW. Epidemiology of acute lower respiratory disease in children. N Engl J Med. 1973; 288:498–505.


4. Sigurs N, Bjarnason R, Sigurbergsson F, Kjellman B. Respiratory syncytial virus bronchiolitis in infancy is an important risk factor for asthma and allergy at age 7. Am J Respir Crit Care Med. 2000; 161:1501–1507.


5. Kim CK, Choi J, Callaway Z, Kim HB, Chung JY, Koh YY, Shin BM. Clinical and epidemiological comparison of human metapneumovirus and respiratory syncytial virus in seoul, Korea, 2003-2008. J Korean Med Sci. 2010; 25:342–347.


6. van den Hoogen BG, de Jong JC, Groen J, Kuiken T, de Groot R, Fouchier RA, Osterhaus AD. A newly discovered human pneumovirus isolated from young children with respiratory tract disease. Nat Med. 2001; 7:719–724.


7. Asthma: a follow up statement from an international paediatric asthma consensus group. Arch Dis Child. 1992; 67:240–248.
8. Barczyk A, Pierzchala W, Sozañska E. Interleukin-17 in sputum correlates with airway hyperresponsiveness to methacholine. Respir Med. 2003; 97:726–733.


9. Laan M, Cui ZH, Hoshino H, Lötvall J, Sjöstrand M, Gruenert DC, Skoogh BE, Lindén A. Neutrophil recruitment by human IL-17 via C-X-C chemokine release in the airways. J Immunol. 1999; 162:2347–2352.
10. Doe C, Bafadhel M, Siddiqui S, Desai D, Mistry V, Rugman P, McCormick M, Woods J, May R, Sleeman MA, Anderson IK, Brightling CE. Expression of the T helper 17-associated cytokines IL-17A and IL-17F in asthma and COPD. Chest. 2010; 138:1140–1147.


11. McKinley L, Alcorn JF, Peterson A, Dupont RB, Kapadia S, Logar A, Henry A, Irvin CG, Piganelli JD, Ray A, Kolls JK. TH17 cells mediate steroid-resistant airway inflammation and airway hyperresponsiveness in mice. J Immunol. 2008; 181:4089–4097.


12. Esnault S, Kelly EA, Nettenstrom LM, Cook EB, Seroogy CM, Jarjour NN. Human eosinophils release IL-1β and increase expression of IL-17A in activated CD4+ T lymphocytes. Clin Exp Allergy. 2012; 42:1756–1764.
13. Rathinam VA, Vanaja SK, Fitzgerald KA. Regulation of inflammasome signaling. Nat Immunol. 2012; 13:333–342.


14. Malmo J, Moe N, Krokstad S, Ryan L, Loevenich S, Johnsen IB, Espevik T, Nordbø SA, Døllner H, Anthonsen MW. Cytokine profiles in human metapneumovirus infected children: identification of genes involved in the antiviral response and pathogenesis. PLoS One. 2016; 11:e0155484.


15. Laham FR, Israele V, Casellas JM, Garcia AM, Lac Prugent CM, Hoffman SJ, Hauer D, Thumar B, Name MI, Pascual A, Taratutto N, Ishida MT, Balduzzi M, Maccarone M, Jackli S, Passarino R, Gaivironsky RA, Karron RA, Polack NR, Polack FP. Differential production of inflammatory cytokines in primary infection with human metapneumovirus and with other common respiratory viruses of infancy. J Infect Dis. 2004; 189:2047–2056.


16. Cheemarla NR, Guerrero-Plata A. Immune response to human metapneumovirus infection: what we have learned from the mouse model. Pathogens. 2015; 4:682–696.


17. Guerrero-Plata A, Casola A, Suarez G, Yu X, Spetch L, Peeples ME, Garofalo RP. Differential response of dendritic cells to human metapneumovirus and respiratory syncytial virus. Am J Respir Cell Mol Biol. 2006; 34:320–329.


18. Guerrero-Plata A, Casola A, Garofalo RP. Human metapneumovirus induces a profile of lung cytokines distinct from that of respiratory syncytial virus. J Virol. 2005; 79:14992–14997.


19. Mukherjee S, Lindell DM, Berlin AA, Morris SB, Shanley TP, Hershenson MB, Lukacs NW. IL-17-induced pulmonary pathogenesis during respiratory viral infection and exacerbation of allergic disease. Am J Pathol. 2011; 179:248–258.


20. Stoppelenburg AJ, Salimi V, Hennus M, Plantinga M, Huis in, Walk J, Meerding J, Coenjaerts F, Bont L, Boes M. Local IL-17A potentiates early neutrophil recruitment to the respiratory tract during severe RSV infection. PLoS One. 2013; 8:e78461.


21. Molet S, Hamid Q, Davoine F, Nutku E, Taha R, Pagé N, Olivenstein R, Elias J, Chakir J. IL-17 is increased in asthmatic airways and induces human bronchial fibroblasts to produce cytokines. J Allergy Clin Immunol. 2001; 108:430–438.


22. Cosmi L, Maggi L, Santarlasci V, Capone M, Cardilicchia E, Frosali F, Querci V, Angeli R, Matucci A, Fambrini M, Liotta F, Parronchi P, Maggi E, Romagnani S, Annunziato F. Identification of a novel subset of human circulating memory CD4(+) T cells that produce both IL-17A and IL-4. J Allergy Clin Immunol. 2010; 125:222–230. 230.e1–230.e4.


23. Sly PD, Boner AL, Björksten B, Bush A, Custovic A, Eigenmann PA, Gern JE, Gerritsen J, Hamelmann E, Helms PJ, Lemanske RF, Martinez F, Pedersen S, Renz H, Sampson H, von Mutius E, Wahn U, Holt PG. Early identification of atopy in the prediction of persistent asthma in children. Lancet. 2008; 372:1100–1106.


24. Kim CK, Chung CY, Choi SJ, Kim DK, Park Y, Koh YY. Bronchoalveolar lavage cellular composition in acute asthma and acute bronchiolitis. J Pediatr. 2000; 137:517–522.


25. Kim CK, Kim SW, Park CS, Kim BI, Kang H, Koh YY. Bronchoalveolar lavage cytokine profiles in acute asthma and acute bronchiolitis. J Allergy Clin Immunol. 2003; 112:64–71.


26. Pifferi M, Ragazzo V, Caramella D, Baldini G. Eosinophil cationic protein in infants with respiratory syncytial virus bronchiolitis: predictive value for subsequent development of persistent wheezing. Pediatr Pulmonol. 2001; 31:419–424.

