Abstract
Pulegone is a naturally occurring organic compound obtained from essential oils from a variety of plants. The aim of this study was to investigate the anti-inflammatory effects through the inhibitory mechanism of inducible nitric oxide synthase (iNOS), cyclooxygenase (COX-2), nuclear factor kappa B (NF-κB), mitogen-activated protein kinases (MAPK) pathways and the activation of nuclear factor erythroid 2-related factor 2 (Nrf2)/ heme oxygenase (HO)-1 pathways in lipopolysaccharide (LPS)-stimulated RAW264.7 cells. Results revealed that pulegone significantly inhibited NO production as well as iNOS and COX-2 expressions. Meanwhile, western blot analysis showed that pulegone down-regulated LPS-induced NF-κB and MAPKs activation in RAW 264.7 cells. Furthermore, the selected compound suppressed LPS-induced intracellular ROS production in RAW 264.7 cells, while the expression of stress response gene, HO-1, and its transcriptional activator, Nrf-2 was upregulated upon pulegone treatment. Taking together, these findings provided that pulegone inhibited the LPS-induced expression of inflammatory mediators via the down-regulation iNOS, COX-2, NF-κB, and MAPKs signaling pathways as well as up-regulation of Nrf-2/HO-1 indicating that pulegone has a potential therapeutic and preventive application in various inflammatory diseases.
Inflammation is an essential biological response of the body to various harmful stimuli which leads to the generation of pro-inflammatory cytokines and mediators. It is the result of several contributing factors such as microbial pathogen, chemicals, irritant and immunological disorders. Although, inflammation is a beneficial host-response upon tissue injury, but upon long persistence, it may result in chronic conditions such as cancer, cardiovascular disease, diabetes, pulmonary disorders, neurological disease, and arthritis.1 The treatment of inflammatory diseases focuses on the suppression of inflammatory mediators such as NO as well as the inhibition of the complex network of signaling pathways including, inducible nitric oxide synthase (iNOS), cyclooxygenase (COX-2), nuclear factor κB (NF-κB), mitogen-activated protein kinase (MAPK), reactive oxygen species (ROS), and proinflammatory cytokines (TNF-α, IL-6, and IL-1β).2
NF-κB is a transcription factor which plays a fundamental role in the inflammatory and acute inflammatory responses.3 In normal cellular homeostatic conditions, NF-κB subunits are inactive and bound with IêB inside cytoplasm. Phosphorylation and desecration of IêB activates NF-κB to translocate toward nucleus and where it begins the transcription of several of key inflammatory genes.4 Furthermore, NF-κB is also involve in the regulation of proinflammatory gene expression which mediates the synthesis of cytokines such as TNF-α, IL-1β, IL-6 and IL-8.5 It also regulates the transcription of COX-2 or iNOS.5 MAPKs, extracellular signal-regulated kinase (ERK), c-Jun N-terminal kinase (JNK) and p38 MAPK, have been associated with the transcriptional regulation of inflammatory genes via NF-κB activation.6
Heme oxygenase-1 (HO-1) is a key anti-oxidant enzyme that catalyzes the degradation of heme and produces biliverdin, ferrous iron, and carbon monoxide. The depletion of heme and generation of biliverdin together with ferrous iron, and carbon monoxide contribute to the anti-inflammatory effects of HO-1.7 Recent studies showed that HO-1 inhibits the excessive production of proinflammatory cytokines as well as reactive oxygen species (ROS) in LPS-stimulated RAW 264.7 cells.8 At the transcription level, HO-1 induction is regulated by a transcription factor called nuclear transcription factor-E2-related factor 2 (Nrf-2). Activation of Nrf-2 regulates the expression of HO-1 and enhances the anti-oxidative ability of cells and therefore, becomes a drug target for the treatment of inflammatory diseases.9
Essential oils are volatile, concentrated hydrophobic, natural, complex compounds mainly extracted from aromatic plants. They are characterized by strong odor. Essential oils were first developed in Middle Ages by Arabs and known for their antiseptic abilities such as antibacterial, anti-fungal, and anti-viral, and medicinal properties and their smell.10 Essential oils have been used in embalmment, preservation of foods and as antimicrobial, analgesic, sedative, anti-inflammatory, spasmolytic and for anesthetic purposes.10 Pulegone (Fig. 1), a naturally occurring organic compound obtained from the essential oils several of plants such as Nepeta cataria (catnip), Mentha piperita, and pennyroyal.1011 Previously, we identified that pulegone essential oil from Korean herb Agastache rugosa through the gas chromatographic analysis.12 Pulegone classified as a monoterpene with characteristic colorless oily liquid and delivers a pleasant odor similar to pennyroyal, peppermint, and camphor. It is used in flavoring agents, in perfumery, and in aromatherapy.13 Earlier reports showed that pulegone exhibit multiple biological activities including antioxidant,14 antimicrobial,14 insecticidal,14 and anticholinesterase activity.15 Although pulegone showed various biological activities, however, the mechanism of its anti-inflammatory actions remain to clarify yet. Therefore, the aim of this study was to focus on the anti-inflammatory activities of pulegone through the inhibition of iNOS, COX-2, NF-κB, and MAPKs expression and the induction of Nrf2/HO-1 expression in LPS-stimulated RAW 264.7 cells.
Pulegone, LPS from Escherichia coli, Griess reagent, 3-[4,5-dimethylthiazol-2-yl]-2,5-di-phenyl tetrazolium bromide (MTT), dimethyl sulfoxide (DMSO), 2 7-dichlorodihydrofluorescein diacetate (DCFH-DA), 2-amino-5,6-dihydro-6-methyl-4H-1,3-thiazine hydrochloride (AMT), 6-hydroxy-2,5,7,8-tetramethylchroman-2-carboxylic acid (Trolox), phenylmethylsulfonyl fluoride (PMSF), fetal bovine serum (FBS) and antibiotics were purchased from Sigma-Aldrich Co. (St. Louis, MO, USA), and Dulbecco's modified Eagle's medium (DMEM) from Hyclone (Logan, UT, USA). Various primary antibodies (iNOS, COX-2, NF-κB (p65), p-ERK, ERK, p-JNK, JNK, p-p38, p38, HO-1, Nrf-2 and β-actin) and secondary antibodies were obtained from Cell signaling Technology Inc. (Beverly, MA, USA) and Santa Cruz Biotechnology Inc. (Santa Cruz, CA, USA) and diluted 1:1000. Polyvinylchloride fluoride (PVDF) membrane (Immobilon-P) was obtained from Millipore Co. (Billerica, MA, USA). Super-signal® West Pico Chemiluminescent Substrate was obtained from Pierce Biotechnology, Inc. (Rockford, IL, USA). All other chemicals and solvents were purchased from Sigma-Aldrich Co., unless stated otherwise.
Marine RAW 264.7 cells were obtained from the American Type Culture Collection (ATCC Rockville, MD, USA). RAW 264.7 cells were cultured in DMEM supplemented with 10% heat-inactivated FBS, and 1% penicillin-streptomycin and 0.1% amphotericin B. The cells were incubated in humidified atmosphere with 5% CO2 at 37 ℃.
Cell viability was assessed using the MTT assay. Briefly, RAW 264.7 cells were seeded into 96-well plates at a density of 1 × 104 cells per well and incubated at 37 ℃ for 24 h. The cells were then treated with the pulegone at various concentrations. After incubation for an additional 24 h at 37 ℃, 100 µl MTT (0.5 mg/ml in PBS) was added to each well and the incubation continued for another 2 hours. The resulting color was assayed at 540 nm using a microplate spectrophotometer (Molecular Devices, Sunnyvale, CA, USA).
The nitrite concentration in the medium was measured using Griess reagent as an indicator of NO production. Briefly, RAW 264.7 cells (2 × 104 cells/well in a 24-well plate with 500 µl culture medium) were pretreated with various concentration of samples for 2 hours and incubated for 18 hours with LPS (1 µg/ml). After incubation, the nitrate concentration of the supernatants (100 µl/well) was measured by adding 100 µl Griess reagent. The absorbance values of mixtures were determined using a micro-plate spectrophotometer (Molecular devices) at 540 nm. The iNOS inhibitor, AMT was used as a positive control.
The intracellular ROS scavenging activity of pulegone was measured using fluorescent probe DCFH-DA. Cells plated in a black 96-well plate at a density of 1 × 104 cells/well were co-treated with various concentration of the pulegone and LPS (1.0 µg/ml) for 2 h. Cells were treated with 20 µM DCFH-DA for 30 min at 37 ℃. The fluorescence intensity was measured at excitation wavelength of 485 nm and an emission wavelength of 528 nm using of fluorescence microplate reader (Dual Scanning SPECTRAmax, Molecular Devices).
Western blotting was used to measure the protein expression of iNOS, COX-2, MAPKs, HO-1, and Nrf-2 according to the procedure of Jung et al. (2017).16
Data were expressed as the means ± standard deviations (SDs) of at least three independent experiments unless otherwise indicated. Data were compared using one-way ANOVA. P values < 0.05, 0.01, and 0.001 were considered statistically significant. All analyzes were performed using SPSS for windows, version 23 (SPSS Inc., Chicago, IL, USA).
Cytotoxicity test was conducted using range of concentrations of pulegone in RAW 264.7 cells. As represented in Fig. 2, incubation of the RAW 264.7 cell with pulegone (6.25 – 50 µg/mL) did not induced any cytotoxic effects when compared with the control. Additionally, cell viability was more than 95% at most of the concentrations. Therefore, pulegone upto 50 µg/mL seemed to be not toxic to RAW 264.7 cells and these non-toxic concentrations were used for respective anti-inflammatory assays.
LPS-stimulated NO generation is important part of inflammatory signaling pathway. In this study, NO production was measured with non-toxic concentrations of pulegone in LPS-activated RAW 264.7 cells. As shown in Fig. 3, LPS induction greatly enhanced NO production. However, pulegone pretreatment at the indicated concentrations dose-dependently inhibited NO production. Moreover, pulegone particularly at 12.5 – 50 µg/mL significantly suppressed NO production compared to LPS-treated cells. AMT, positive control, significantly inhibited LPS-induced NO release from RAW 264.7 cells (Fig. 3).
To further investigate the whether the anti-inflammatory potential of pulegone was due to the inhibition of NO, Western blot analysis was performed. According to Fig. 4, LPS exposure significantly up-regulated iNOS and COX-2 expression compared to non-treated cells. However, pulegone treatment in a dose response manner down-regulated iNOS and COX-2 expressions. Interestingly, higher concentration exhibited promising down-regulatory effects against iNOS and COX-2 protein expression.
To determine whether pulegone affects LPS-induced activation and translocation of NF-κB, the phosphorylation of p65 subunit was examined by immunoblotting. Fig. 5 clearly shown that LPS treatment sharply enhanced phosphorylation of p65. Whereas, pulegone exhibited moderate down-regulatory effects on translocation of p65 (NF-κB).
The phosphorylation levels of MAPKs were analyzed in LPS-treated RAW 264.7 cells by Western blotting. MAPKs including ERK, JNK, and p38 signaling also play a vital part in regulating the LPS-induced inflammatory process. Furthermore, phosphorylation of MAPKs is also closely linked to the regulation of NF-κB activation. As Fig. 6 depicted that the inhibitory activities of pulegone on ERK, JNK, and p38 phosphorylation after 2 h of LPS stimulation in RAW 264.7 cells. These results demonstrated that the tested compound significantly down-regulated phosphorylation of ERK, JNK, and p38 in LPS-treated RAW 264.7 cells. The inhibition of the phosphorylation of JNK and p38 for pulegone are higher than the inhibition of the phosphorylation of ERK.
It has been proposed that inflammation is accompanied by cellular oxidative stress. Therefore, in order to understand that anti-inflammatory mechanic of pulegone was associated with scavenging ROS generation. Inhibitory effects of pulegone on LPS-induced ROS generation in RAW 264.7 cells was investigated. LPS-exposure significantly enhanced ROS generation from RAW 264.7 cells. However, overproduced ROS was profoundly scavenged dose-dependently by pulegone, particularly at 25 and 50 µg/ml pulegone exhibited promising ROS inhibitory effects (Fig. 7). Positive control, trolox, also shown to significantly suppressed ROS generation in LPS-induced RAW 264.7 cells.
HO-1 is a key anti-oxidant enzyme provides protection against oxidative stress. Down-regulation of HO-1 in cells indicates the cells experience oxidative stress. HO-1 expression upon LPS-treatment to RAW 264.7 cells begins to deplete. Whereas, pretreatment of pulegone upto 50 µg/ml strongly up-regulated HO-1 expression compared to LPS-treated cells. As expected, pulegone treatment led to a significant increase in HO-1 protein expression in LPS-induced RAW 264.7 cells (Fig. 8A). Nrf-2 is a transcription factor which regulates the expression of HO-1 to protect cells against oxidative stress. Accordingly, Nrf-2 modulating potential of pulegone were investigated by Western blot in LPS-induced RAW 264.7 cells. As shown in results (Fig. 8B), pulegone significantly up-regulated Nrf-2 expression compared to the non-treated group, which indicated that the protective effects of pulegone against oxidative stress was associated with down-regulation of Nrf-2 protein expression.
Pulegone is naturally occurring essential oil obtained from various aromatic plants. The plants containing essential oils are usually located in warm countries like Mediterranean and tropical countries.10 Essential oils are lipophilic in nature and highly volatile secondary plant metabolites. Essential oils have been reported to greatly use in perfumery, cosmetics, feed, food, and beverage industries. Despite their wide use as fragrances, it is important to focus on their mode of biological action for the application in human health. Like other essential oils, pulegone is found in many plants like peppermint, pennyroyal and blue mint bush. In alternative medicine practices, peppermint oil is used in aromatherapy and has been claimed to have beneficial effects in the treatment of mental diseases and has also been found to improve cognitive performance and increase alterness.1718 Moreover, it has recently reported that a near-fatal case due to ingestion of a toxic dose of peppermint oil.19 In order to treat dizziness, pennyroyal has been used as an anticonvulsive and as a sedative and is still sometimes used as an abortifacient.20 Blue mint bush has been used as a sedative. In cooking, blue mint has been also used to prevent food decay.21 Even though pennyroyal is used in traditional medicine, it is known to be extremely hepatotoxic and neurotoxic. In laboratory animals, pulegone causes extensive liver damage,22 and human intoxication with pennyroyal oil (or with a mint tea containing this oil) is associated with the loss of renal function, hepatotoxicity, dizziness, epileptic encephalopathy and in severe cases, death.23 Even if the hepatotoxic effects of pulegone have been extensively demonstrated and studied, the behavioral effects of this terpenic compound have only recently gained attention in the field.24 It has reported that pulegone increases mouse locomotor activity, and this effect was sensitive to dopamine receptor antagonists, suggesting that dopamine was involved in the ambulation promoted by pulegone.25
The present work was carried out to understand the molecular mechanism of essential oil derived compound, pulegone, towards the inhibition of the inflammatory reactions. It was previously reported that pulegone inhibited the lens protein-induced inflammation by 58% when 50 µl of 0.5% pulegone was instilled into the cul de sac of the rabbit eyes.26 Recently, Schizonepeta volatile oil, comprising of menthone (46.67 %) and pulegone (33.92 %) has been found to reduce the inflammatory cytokines level in LPS-induced exotoxin-poisoned mice.27 We investigated the effects of pulegone on NF-κB and Nrf-2 signaling in LPS-stimulated RAW 264.7 cells. Our results indicated that pulegone effectively inhibited the production of NO through suppressing iNOS, COX-2, NF-κB, and MAPKs signaling as well as up-regulating Nrf-2/HO-1 signaling. These data indicate that pulegone may be applied as a pharmaceutical agent for handling or preventing inflammatory disease.
The overproduction of NO is associated with the inflammatory process. Numerous studies have shown that the overproduction of NO can lead the inflammatory reactions.2829 Therefore, inhibition of NO production together with down-regulation of iNOS and COX-2 protein expression might have crucial therapeutic value for the prevention of inflammatory diseases. Our study showed that pulegone inhibited NO production in a dose-dependent manner in LPS-treated RAW 264.7 cells without showing any signs of cell toxicity upto 50 µg/ml. Furthermore, pulegone found to be significantly down-regulated iNOS and COX-2 protein expression.
Recent investigations revealed that LPS-induced inflammation is highly associated with diversified intracellular signaling pathways, such as NF-κB and MAPKs. NF-κB is largely involved in the expression of number of proinflammatory genes such as iNOS, COX-2, TNF-α, IL-1β, and IL-6 in LPS-induced inflammation.303132 Predominantly, in normal state NF-κB resides in the cytoplasm in inactive form, however, upon stimulation such as LPS endotoxin it activates and start translocation towards nucleus and promotes the transcription process of target inflammatory genes. Therefore, modulation of NF-κB could provide an effective therapeutic target for treating inflammatory diseases. Similarly, current study signifies that pulegone dose-dependently down-regulated NF-κB protein expression levels in LPS-induced RAW 264.7 cells. The MAPK families consist of ERK1/2, JNK, and p38 that control cellular signal transduction in response to inflammation.33
The activation of MAPKs signaling cascade are also associated with the activation of NF-κB. Therefore, the mechanism of NF-κB inactivation is also related to the down-regulatory expression of phosphorylation of ERK1/2, JNK, and p38. Western blot analysis demonstrated that pulegone significantly suppressed the phosphorylation of JNK and p38 in LPS-stimulated RAW 264.7 cells. Moreover, among MAPKs, pulegone strongly down-stream the signaling of p38 compared to ERK and JNK protein expressions.
Overproduction of free radicals can damage cellular components like cellular macromolecules, DNA, lipids and proteins. ROS-derived oxidative stress is a major factor in the progression of inflammatory reactions34 and that LPS can facilitate the cellular ROS generation which is also related to the expression of inflammatory signaling pathways mediated by NF-κB.35 Recent study revealed that oxidative stress strongly affects the activation of NF-κB.36 Moerover, antioxidant molecules can inhibit the production of proinflammatory mediators.37 In the present study, pretreatment of pulegone significantly scavenged LPS-stimulated ROS overproduction in RAW 264.7 cells. Particularly, ROS scavenging effects were prominent at 25 and 50 µg/ml. These results indicated that pulegone induced significant anti-oxidative effects by scavenging ROS generation.
It is currently believed that the inducible HO-1 is a stress responsive protein and has anti-inflammatory activities.38 HO-1 degrades heme through catalyzation process and produces iron, carbon monoxide, and biliverdin. Later, biliverdin is converted into bilirubin, which is a strong antioxidant. Various studies indicate that the induction of HO-1 inhibits LPS-induced inflammatory response activated by Nrf-2 pathways.3940 Transcription factor Nrf-2 activation is the primary defense against cellular oxidative stress. Nrf-2 translocates in the nucleus after the dissociation from keap1 and binds to antioxidant response element (ARE) and regulates the transcription of HO-1 protein in LPS-induced RAW 264.7 cells.39 Inducible HO-1 inhibits the excessive production of TNF-α and IL-1β through Nrf-2 activation in response to LPS-induced RAW 264.7 cells.41 Therefore, while considering the anti-oxidative potential of pulegone, the expression of key anti-oxidative enzyme, HO-1, and the expression of its transcriptional activator protein, Nrf-2, has been evaluated and results showed very strong anti-oxidative effects of pulegone at the indicated concentrations. The expression of both HO-1 and Nrf-2 was up-regulated in response to pulegone exposure in LPS-induced RAW264.7 cells, signifying the anti-oxidative modulatory mechanism of pulegone.
Although pulegone exhibited potent in vitro anti-inflammatory activity, but its overall function in vivo has yet to be clarified. Plugenol toxicity was conducted in mice and rats over the period of 2 years and results demonstrate that under the conditions of 2-year gavage studies, there was no signs of carcinogenic activity of pulegone in male F344/N rats at dose of 18.75, 37.5, or 75mg/kg. However, pulegone in male and female B6C3F1 mice as well as in female F344/N rats was found to be carcinogenic. Moreover, pulegone administration was also linked with the incidence of nonneoplastic lesions in liver and nose of rats and mice and in the forestomach of male and female mice and male rats.42
In conclusion, these findings demonstrated that pulegone significantly inhibits the production of inflammatory mediators and up-regulate antioxidant protein expression in LPS-stimulated RAW 264.7 cells. Moreover, the inhibitory actions of pulegone are likely associated with the regulation of NF-κB, MAPKs, and HO-1/Nrf-2 signaling pathways. Verification of anti-inflammatory action of pulegone and justifying relative mechanisms in in vivo models will be beneficial for application of pulegone as therapeutic agents for inflammatory diseases.
Figures and Tables
Fig. 2
Cell viability of pulegone measured by the MTT assay. Values represent the mean ± SD of three independent experiments.
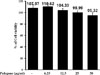
Fig. 3
Inhibitory effects of pulegone on the production of NO in LPS-stimulated RAW264.7 cells. Cells were pretreated with different concentrations of pulegone for 2 h and then stimulated with LPS (1.0 µg/ml) for 24 h. The culture media were used to measure the amount of nitrite to determine NO production. Data are presented as mean ± SD of three independent experiments. ###p < 0.001 indicates significant difference from the control group. *p < 0.05, **p < 0.01, and ***p < 0.001 indicate significant difference from the LPS-treated group.
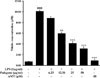
Fig. 4
Inhibitory effects of pulegone on the expression of iNOS and COX-2 in LPS-stimulated RAW264.7 cells. Cells were pretreated with the indicated concentration of pulegone for 2 h and stimulated with LPS (1.0 µg/ml) for 18 h. The expression of iNOS, COX-2, and β-actin was detected by Western blot using corresponding antibodies. The results presented are representative of three independent experiments. #p < 0.05 indicates a significant difference from the control group. *p < 0.05 indicates a significant difference from the LPS-treated group.
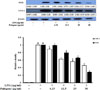
Fig. 5
Inhibitory effects of pulegone on the expression of NF-κB in LPS-stimulated RAW264.7 cells. Cells were pretreated with the indicated concentration of pulegone for 2 h and stimulated with LPS (1.0 µg/ml) for 18 h. The expression of NF-κB and β-actin was detected by Western blot using corresponding antibodies. The results presented are representative of three independent experiments. #p < 0.05 indicates a significant difference from the control group. *p < 0.05 indicates a significant difference from the LPS-treated group.
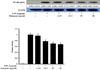
Fig. 6
Inhibitory effects of pulegone on the expression of MAPKs in LPS-stimulated RAW264.7 cells. Cells were pretreated with the indicated concentration of pulegone for 2 h and stimulated with LPS (1.0 µg/ml) for 18 h. The expression of MAPKs was detected by Western blot using corresponding antibodies. The results presented are representative of three independent experiments. #p < 0.05 indicates a significant difference from the control group. *p < 0.05 indicate a significant difference from the LPS-treated group.
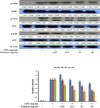
Fig. 7
Inhibitory effects of pulegone on the production of ROS in LPS-stimulated RAW264.7 cells. Cells pretreated with different concentration of pulegone for 2 h and stimulated with LPS (1.0 µg/ml) for 24 h. ROS levels were measured by fluorescence analysis of DCFH-DA. Data are presented as mean ± SD of three independent experiments. ###p < 0.01 indicates a significant difference from the control group. *p < 0.05, **p < 0.01, and ***p < 0.001 indicate significant differences from the LPS-treated group.
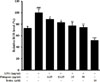
Fig. 8
Inhibitory effects of pulegone on the expression of HO-1 (A) and Nrf2 (B) in LPS-stimulated RAW264.7 cells. Cells were pretreated with indicated concentration of pulegone for 2 h and stimulated with LPS (1.0 µg/ml) for 18 h. The expression of HO-1 and Nrf2 was detected by Western blot using corresponding antibodies. The results presented are representative of three independent experiments. #p < 0.05 indicates a significant difference from the control group. *p < 0.05 indicates a significant difference from the LPS-treated group.
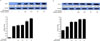
Acknowledgements
This research was supported by the Basic Science Research Program through the National Research Foundation of Korea (NRF), funded by the Ministry of Education (2012R1A6A1028677).
References
1. Choudhari AS, Raina P, Deshpande MM, Wali AG, Zanwar A, Bodhankar SL, Kaul-Ghanekar R. J Ethnopharmacol. 2013; 150:215–222.
2. Joung EJ, Lee B, Gwon WG, Shin T, Jung BM, Yoon NY, Choi JS, Oh CW, Kim HR. Int Immunopharmacol. 2015; 29:693–700.
3. Giuliani C, Napolitano G, Bucci I, Montani V, Monaco F. Clin Ter. 2001; 152:249–253.
4. May MJ, Ghosh S. Immunol Today. 1998; 19:80–88.
5. Tak PP, Firestein GS. J Clin Invest. 2001; 107:7–11.
6. Kim AR, Lee MS, Shin TS, Hua H, Jang BC, Choi JS, Byun DS, Utsuki T, Ingram D, Kim HR. Toxicol in Vitro. 2011; 25:1789–1795.
7. Pae HO, Chung HT. Immune Netw. 2009; 9:12–19.
8. Lee IS, Lim J, Gal J, Kang JC, Kim HJ, Kang BY, Choi HJ. Neurochem Int. 2011; 58:153–160.
9. Taha R, Blaise G. Funct Food Health Dis. 2014; 4:510–523.
10. Bakkali F, Averbeck S, Averbeck D, Idaomar M. Food Chem Toxicol. 2008; 46:446–475.
11. Sullivan JB, Rumack BH, Thomas H, Peterson RG, Bryson P. J Am Med Assoc. 1979; 242:2873–2874.
12. Choi JS, Song BM, Park HJ. Kor J Pharmacogn. 2016; 47:192–196.
13. Kumar P, Mishra S, Malik A, Satya S. Ind Crops Prod. 2011; 34:802–817.
14. Brahmi F, Abdenour A, Bruno M, Silvia P, Alessandra P, Danilo F, Drifa YG, Fahmi EM, Khodir M, Mohamed C. Ind Crops Prod. 2016; 88:96–105.
15. Ertas A, Gören AC, Hasimi N, Tolan V, Kolak U. Rec Nat Prod. 2015; 9:105–115.
16. Jung HA, Roy A, Abdul QA, Kim HR, Park HJ, Choi JS. Nat Prod Sci. 2017; 23:183–191.
17. Yim VWC, Ng AKY, Tsang HWH, Leung AY. J Altern Complement Med. 2009; 15:187–195.
18. Moss M, Hewitt S, Moss L, Wesnes K. Int J Neurosci. 2008; 118:59–77.
19. Nath SS, Pandey C, Roy D. Indian J Anaesth. 2012; 56:582–584.
20. Di Stasi LC, Oliveira GP, Carvalhaes MA, Queiroz-Junior M, Tien OS, Kakinami SH, Reis MS. Fitoterapia. 2002; 73:69–91.
21. Beikmohammadi M. World Appl Sci J. 2011; 12:1635–1638.
22. McClanahan RH, Thomassen D, Slattery JT, Nelson SD. Chem Res Toxicol. 1989; 2:349–355.
23. Bakerink JA, Gospe SM Jr, Dimand RJ, Eldridge MW. Pediatrics. 1996; 98:944–947.
24. de Sousa DP, Nóbrega FFF, de Lima MRV, de Almeida RN. Naturforsch C. 2011; 66:353–359.
25. Umezu T. Pharmacol Biochem Behav. 2010; 94:497–502.
26. Yao QS, Chiou GC. Zhongguo Yao Li Xue Bao. 1993; 14:13–17.
27. Wen TQ, Sang WT, Xu F, Wang F, Zeng N. Zhongguo Zhong Yao Za Zhi. 2016; 41:4642–4647.
28. Meda L, Cassatella MA, Szendrei GI, Otvos L Jr, Baron P, Villalba M, Ferrari D, Rossi F. Nature. 1995; 374:647–650.
29. Dandona P, Chaudhuri A, Dhindsa S. Diabetes Care. 2010; 33:1686–1687.
30. Marks-Konczalik J, Chu SC, Moss J. J Biol Chem. 1998; 273:22201–22208.
31. Islam MN, Choi RJ, Jin SE, Kim YS, Ahn BR, Zhao D, Jung HA, Choi JS. J Ethnopharmacol. 2012; 144:175–181.
32. Chen JJ, Huang WC, Chen CC. Mol Biol Cell. 2005; 16:5579–5591.
33. Kaminska B. Biochim Biophys Acta. 2005; 1754:253–262.
34. Hancock JT, Desikan R, Neill S. J Biochem Soc Trans. 2001; 29:345–349.
35. Choi SY, Hwang JH, Ko HC, Park JG, Kim SJ. J Ethnopharmacol. 2007; 113:149–155.
36. Siomek A. Acta Biochem Pol. 2012; 59:323–331.
37. Ryan KA, Smith MF Jr, Sanders MK, Ernst PB. Infect Immun. 2004; 72:2123–2130.
38. Kim JH, Choo YY, Tae N, Min BS, Lee JH. Int Immunopharmacol. 2014; 22:420–426.
39. Lee MY, Lee JA, Seo CS, Ha H, Lee H, Son JK, Shin HK. Food Chem Toxicol. 2011; 49:1047–1055.
40. Paine A, Eiz-Vesper B, Blasczyk R, Immenschuh S. Biochem Pharmacol. 2010; 80:1895–1903.
41. Tsoyi K, Lee TY, Lee YS, Kim HJ, Seo HG, Lee JH, Chang KC. Mol Pharmacol. 2009; 76:173–182.
42. Auerbach SS, Elmore SA, Bishop JB, Bucher JR, Chan PC, Chhabra RS, Foster PM, Herbert RA, Hooth MJ, King-Herbert AP, Kissling GE, Malarkey DE, Roycroft JH, Sanders JM, Smith CS, Travlos GS, Walker NJ, Witt KL, Hejtmancik MR, Sells DM, Skowronek AJ, Toft JD II, Hamlin MH II, Brix AE, Hard GC, Kolenda-Roberts HM, Peckham JC, Wolfe GW, Seung HS, Brecher S, Iyer S, Tharakan VS, Morrison JP, Elmore SA, Flake GP, Hard GC, Kolenda-Roberts HM, Malarkey DE, Maronpot RR, Peckham JC, Morrison JP, Belpoggi F, Brix AE, Elmore SA, Flake GP, Hard GC, Herbert RA, Malarkey DE, Maronpot RR, Wakamatsu N, Crockett PW, Betz LJ, McGowan KP, Gunnels SR, Coker KK, Harper LM, Serbus DC. Natl Toxicol Program Tech Rep Ser. 2011; 563:1–201.