Abstract
In a search for novel treatments for diabetic complications from natural resources, we found that the ethyl acetate-soluble fraction from the 80% ethanol extract of the leaves of Homonoia riparia has a considerable inhibitory effect on advanced glycation end product (AGE) formation. Bioassay-guided isolation of this fraction resulted in identification of 15 phenolic compounds (1 – 15). These compounds were evaluated in vitro for inhibitory activity against the formation of AGE. The majority of tested compounds, excluding ethyl gallate (15), markedly inhibited AGE formation, with IC50 values of 2.2 – 89.9 µM, compared with that of the positive control, aminoguanidine (IC50 = 962.3 µM). In addition, the effects of active isolates on the dilation of hyaloid-retinal vessels induced by high glucose (HG) in larval zebrafish was investigated; (–)-epigallocatechin-3-O-gallate (6), corilagin (7), and desmanthine-2 (11) significantly decreased HG-induced dilation of hyaloid–retinal vessels compared with the HG-treated control group.
Natural products have been a source of medicinal agents for thousands of years, and numerous currently prescribed drugs are derived either directly or indirectly from natural products.12 These products exhibit enormous structural and chemical diversity, exceeding those of synthetic libraries. Approximately 40% of chemical scaffolds found in natural products are absent in the current medicinal chemistry repertoire. Thus, natural products represent an important source of new structural leads, which could provide drugs for all major disease areas. The major classes of plant phytochemicals include phenolics, terpenoids and essential oils, alkaloids, and polypeptides.3 Among these, phenolic compounds are the most numerous and structurally diverse plant phytochemicals that exist in nature.
Homonoia riparia Lour. (Euphorbiaceae) is a small genus of shrubs or small trees with a widespread distribution from India to China, Taiwan, and throughout Malesia to New Guinea, and it is found commonly in groups along riverbanks. The leaves and fruits of the plant have depurative and antiseptic properties and are used for the treatment of wounds, ulcers, and skin diseases.4 The roots exhibit laxative, diuretic, refrigerant, depurative, and emetic properties, and a decoction of the roots is used to treat bladder stones, chest pain, gonorrhea, hemorrhoids, syphilis, and thirst.4 Earlier phytochemical investigation of H. riparia afforded sterols, fatty acids, triterpenoids, and flavonoids.567 Recently, six new cycloartane-type triterpenes purified from this plant were shown to exhibit inhibitory activity against VEGF-induced angiogenesis.8
In our continuing efforts to identify effective, naturally sourced therapeutic agents for diabetic complications, we found that the ethyl acetate (EtOAc)-soluble fraction from the 80% ethanol (EtOH) extract of the leaves of H. riparia exhibits a considerable inhibitory effect on advanced glycation end product (AGE) formation. Bioassay-guided isolation of this fraction resulted in the isolation of 15 phenolic compounds (1 – 15). This report describes the isolation and structural elucidation of these phenolic compounds, as well as the characterization of their inhibitory effects on AGE formation. We further investigated the effects of active isolates on the dilation of high glucose (HG)-induced hyaloid-retinal vessels in larval zebrafish.
1H-NMR (300MHz) and 13C-NMR (75MHz) spectra were obtained using the Bruker DRX-300 spectrometer with tetramethylsilane as an internal standard. ESI-MS was performed using the Shimadzu LCMS-IT-TOF spectrometer. Column chromatography was performed using silica gel (70 – 230 mesh and 230 – 400 mesh, Merck), YMC-gel ODS-A (S-75 µm, YMC), and Sephadex LH-20 (Amersham Pharmacia Biotech). Thin-layer chromatography was performed on pre-coated silica gel 60 F254 (0.25 mm, Merck) and RP-18 F254s plates (0.25 mm, Merck). Spots were detected by UV light (254 nm) and spraying 10% H2SO4 followed by heating.
The leaves of H. riparia were collected in Hanoi, Vietnam, in June 2012 and identified by Prof. J.-H. Kim, Gachon University, Republic of Korea. A voucher specimen (no. VN-118) has been deposited in the Herbarium of Korea Institute of Oriental Medicine, Republic of Korea.
Air-dried H. riparia leaves (5.0 kg) were extracted using 80% EtOH (3 × 50 L) at room temperature for 7 days by maceration, after which they were filtered and concentrated to yield an 80% EtOH extract (650 g). This extract (300 g) was suspended in H2O (5 L) and then partitioned successively using n-hexane (3 × 5 L), EtOAc (3 × 5 L), and n-BuOH (3 × 5 L) to afford n-hexane- (10.1 g), EtOAc- (78.0 g), and n-BuOH-soluble fractions (50.2 g), respectively. The EtOAcsoluble fraction (50 g), which significantly inhibited AGE formation, was subjected to silica gel column chromatography (70 – 230 µm, 60 × 15 cm) and eluted using a CH2Cl2-MeOH solvent gradient (50:1 → 0:1) to generate five fractions (A–E).
Fraction A (5.5 g) was subjected to YMC RP-18 column chromatography (50 × 5 cm) and eluted using a MeOH-H2O gradient (1:10 → 10:1) to afford three subfractions (A1–A3). Subfraction A2 (0.9 g) was subjected to further chromatography using a Sephadex LH-20 column (60 × 3 cm) and a MeOH–H2O gradient (1:5 → 2:1) to obtain 1 (30 mg), 2 (18 mg), and 3 (8 mg). Chromatography of fraction B (13.5 g) on a silica gel column (70 – 230 mesh, 50 × 10 cm), eluting using a CH2Cl2–MeOH gradient (50:1 → 0:1), yielded three subfractions (B1–B3). Subfraction B1 (3.2 g) was further purified on a Sephadex LH-20 column (60 × 3 cm) using a MeOH–H2O gradient (1:2 → 2:1) to obtain 4 (18 mg) and 5 (45 mg). Chromatography of fraction B2 (5.5 g) on a YMC RP-18 column (50 × 5 cm) using a MeOH–H2O gradient (1:1 → 4:1) afforded 6 (85 mg), 7 (10 mg), 8 (15 mg), and 9 (80 mg). Fraction C (8.8 g) was subjected to silica gel column chromatography (70 – 230 mesh, 50 × 10 cm) using a CH2Cl2–MeOH gradient (50:1 → 0:1) to afford three fractions (C1–C3). Subfraction C2 (2.0 g) was chromatographed separately on a Sephadex LH-20 column (60 × 3 cm), eluting using a MeOH–H2O gradient (1:2 → 2:1) to yield 10 (10 mg), 11 (9 mg), and 12 (7 mg). Chromatography of fraction E (3.5 g) on a YMC RP-18 column (50 × 5 cm) using a MeOH–H2O gradient (1:10 → 10:1) afforded four subfractions (E1–E4), of which E2 (0.8 g) was further chromatographed on a Sephadex LH-20 column (60 × 3 cm), eluting using a MeOH–H2O gradient (1:4 → 2:1) to obtain 13 (40 mg), 14 (13 mg), and 15 (15 mg).
Yellow powder; ESI-MS m/z : 539 [M+H]+; 1H-NMR (300 MHz, DMSO-d6): δ 8.01 (1H, d, J = 2.0 Hz, H-6‴), 7.99 (1H, d, J = 2.0 Hz, H-2‴), 7.58 (2H, d, J = 8.0 Hz, H-2′/6′), 7.15 (1H, d, J = 8.0 Hz, H-‴), 6.82 (1H, s, H-3″), 6.78 (1H, s, H-3), 6.72 (2H, d, J = 8.0 Hz, H-3′/5′), 6.45 (1H, d, J = 2.0 Hz, H-8″), 6.39 (1H, s, H-6), 6.18 (1H, d, J = 2.0 Hz, H-6″); 13C-NMR (75 MHz, DMSO-d6): δ 182.2 (C-4), 181.8 (C-4″), 164.2 (C-2″), 163.9 (C-2), 163.9 (C-7″), 161.9 (C-7), 161.6 (C-4′), 161.1 (C-5″), 160.6 (C-5), 160.6 (C-4‴), 159.6 (C-9″), 154.6 (C-9), 131.5 (C-6‴), 128.3 (C-2′/6′), 127.9 (C-2″), 121.5 (C-3‴), 121.1 (C-1‴), 120.1 (C-1′), 116.3 (C-5‴), 115.9 (C-3′/5′), 103.8 (C-10), 103.8 (C-10″), 103.6 (C-8), 103.1 (C-3″), 102.7 (C-3), 98.9 (C-6), 98.7 (C-6″), 94.1 (C-8″).
Yellow powder; ESI-MS m/z : 446 [M–H]−; 1H-NMR (300 MHz, DMSO-d6): δ 8.00 (2H, d, J = 8.5 Hz, H-2′/6′), 6.85 (2H, J = 9.0 Hz, H-3′/5′), 6.18 (1H, d, J = 2.2 Hz, H-8), 5.99 (1H, d, J = 2.2 Hz, H-6), 5.40 (1H, d, J = 7.0 Hz, H-1″), 3.57 (1H, d, J = 11.0 Hz, H-6a″), 3.36 (1H, dd, J = 12.0, 4.5 Hz, H-6b″), 3.23–3.06 (4H, m, H-2″/3″/4″/5″); 13C-NMR (75 MHz, DMSO-d6): δ 176.4 (C-4), 161.0 (C-7), 161.1 (C-5), 160.2 (C-4′), 156.9 (C-9), 155.1 (C-2), 132.8 (C-3), 130.6 (C-2′/6′), 120.8 (C-1′), 115.1 (C-3′/5′), 105.0 (C-10′), 101.3 (C-1″), 100.3 (C-6), 94.3 (C-8), 77.3 (C-3″), 76.5 (C-5″), 74.2 (C-2″), 69.8 (C-4″), 61.8 (C-6″).
Yellow powder; ESI-MS m/z : 435 [M+H]+; 1H-NMR (300 MHz, CD3OD): δ 7.52 (1H, d, J = 2.0 Hz, H-2′), 7.50 (1H, dd, J = 8.5, 2.0 Hz, H-6′), 6.90 (1H, d, J = 8.5 Hz, H-5′), 6.39 (1H, d, J = 2.0 Hz, H-8), 6.21 (1H, d, J = 2.0, H-6), 5.47 (1H, d, J = 1.0 Hz, H-1″), 4.33 (1H, J = 2.0 Hz, H-2″), 3.92–3.86 (2H, m, H-3″/4″), 3.51–3.49 (2H, m, H-5″); 13C-NMR (75 MHz, CD3OD): δ 180.1 (C-4), 166.3 (C-7), 163.2 (C-5), 159.4 (C-2), 158.7 (C-9), 150.0 (C-4′), 146.5 (C-3′), 135.0 (C-3), 123.2 (C-6′), 123.1 (C-1′), 116.9 (C-5′), 116.5 (C-2′), 109.6 (C-1″), 105.7 (C-10), 100.0 (C-6), 94.9 (C-8), 88.1 (C-4″), 83.4 (C-2″), 78.8 (C-3″), 62.7 (C-5″).
Yellow powder; ESI-MS m/z : 471 [M+Na]+; δ 1H-NMR (300 MHz, CD3OD): δ 7.33 (1H, d, J = 2.0 Hz, H-2′), 7.30 (1H, dd, J = 8.0, 2.0 Hz, H-6′), 6.90 (1H, d, J = 8.0 Hz, H-5′), 6.34 (1H, d, J = 2.1 Hz, H-8), 6.20 (1H, d, J = 2.1 Hz, H-6), 5.34 (1H, d, J = 1.5 Hz, H-1″), 4.22 (1H, dd, J = 3.0, 1.7 Hz, H-2″), 3.44–3.30 (3H, m, H-3″/4″/5″), 0.94 (3H, d, J = 6.0 Hz, H-6″); 13CNMR (75 MHz, CD3OD): δ 179.5 (C-4), 165.7 (C-7), 163.1 (C-5), 159.2 (C-2), 158.4 (C-9), 149.7 (C-4′), 146.3 (C-3′), 136.2 (C-3), 122.9 (C-6′), 122.8 (C-1′), 116.9 (C-2′), 116.3 (C-5′), 105.8 (C-10), 103.5 (C-1″), 99.7 (C-6), 94.7 (C-8), 73.2 (C-4″), 72.1 (C-2″), 72.0 (C-3″), 71.8 (C-5″), 17.6 (C-6″).
Yellow powder; ESI-MS m/z : 465 [M+H]+; 1H-NMR (500 MHz, DMSO-d6): δ 7.66 (1H, dd, J = 8.4, 2.1 Hz, H-6′), 7.54 (1H, d, J = 2.1 Hz, H-2′), 6.82 (1H, d, J = 8.4 Hz, H-5′), 6.39 (1H, d, J = 1.8 Hz, H-8), 6.18 (1H, d, J = 1.8 Hz, H-6), 5.35 (1H, d, J = 7.8 Hz, H-1″), 3.27–3.66 (5H, m, H-2″/3″/4″/5″/6″); 13C NMR (125 MHz, DMSO-d6): δ 177.5 (C-4), 164.2 (C-7), 161.3 (C-5), 156.34 (C-2), 156.2 (C-9), 148.5 (C-4′), 144.9 (C-3′), 133.5 (C-3), 122.0 (C-6′), 121.1 (C-1′), 116.0 (C-5′), 115.2 (C-2′), 104.0 (C-10), 101.8 (C-1″), 98.7 (C-6), 93.5 (C-8), 75.9 (C-5″), 73.2 (C-3″), 71.3 (C-2″), 68.0 (C-4″), 60.2 (C-6″).
Yellow powder; ESI-MS m/z : 459 [M+H]+; 1H-NMR (300 MHz, acetone-d6): δ 7.02 (2H, s, H-2″/6″), 6.60 (2H, s, H-2′/6′), 6.10 (1H, d, J = 1.8 Hz, H-8), 6.01 (1H, d, J = 1.8 Hz, H-6), 5.53 (1H, m, H-3), 5.02 (1H, d, J = 7.5 Hz, H-2), 3.00 (dd, J = 16.8, 4.4 Hz, H-4ax), 2.90 (dd, J = 16.8, 3.5 Hz, H-4eq); 13C-NMR (75 MHz, CD3OD): δ 165.6 (COO), 157.3 (C-9), 156.3 (C-7), 156.1 (C-5), 146.5 (C-3″/5″), 145.8 (C-3′/5′), 139.1 (C-4″), 133.1 (C-4′), 128.9 (C-1′), 119.3 (C-1″), 109.0 (C-2″/6″), 105.3 (C-2′/6′), 97.7 (C-10), 95.7 (C-8), 94.3 (C-6), 77.3 (C-2), 69.3 (C-3), 26.8 (C-4).
Yellowish powder; ESI-MS m/z : 633 [M–H]−; 1H-NMR (300 MHz, acetone-d6): δ 7.07 (2H, s, galloyl-H), 6.70, 6.84 (each 1H, s, hexahydroxydiphenoyl-H), 6.38 (1H, d, J = 2.0 Hz, H-1), 5.0 (2H, m, H-6), 4.55 – 4.00 (4H, m, H-2/3/4/6); 13C-NMR (75MHz, acetone-d6): δ 170.2/168.7/166.8 (ester carbonyl C), 146.5/146.1/145.7/145.6/145.5/140.5 (hydroxylated carbon atoms), 126.2/125.8/122.1 (the ester link carbons on the aromatic rings), 120.7/117.4 (C atoms linking the diphenyl rings of the diphenoyl), 111.1 (galloyl-C), 110.3/108.4 (hexahydroxydiphenoyl-C), 95.1 (C-1), 76.3 (C-5), 71.7 (C-3), 69.6 (C-2), 65.1 (C-6), 62.6 (C-4).
Yellow powder; ESIMS m/z : 481 [M+H]+; 1H-NMR (300 MHz, CD3OD): δ 7.31 (2H, s, H-2′/6′), 6.37 (1H, d, J = 2.1 Hz, H-8), 6.18 (1H, d, J = 2.1 Hz, H-6), 5.18 (1H, d, J = 6.8 Hz, H-1″), 3.57 (1H, d, J = 11.0 Hz, H-6a″), 3.36 (1H, dd, J = 12.0, 4.5 Hz, H-6b″), 3.63–3.06 (4H, m, H-2″/3″/4″/5″); 13CNMR (75 MHz, CD3OD): δ 179.5 (C-4), 166.1 (C-7), 163.3 (C-5), 159.7 (C-2), 158.8 (C-9), 146.9 (C-3′/5′'), 138.1 (C-4′), 136.5 (C-3), 122.2 (C-1′), 109.8 (C-2′/6′), 109.6 (C-1″), 105.9 (C-10), 99.9 (C-6), 94.8 (C-8), 88.1 (C-4″), 83.4 (C-2″), 78.8 (C-3″), 62.7 (C-5″).
Yellow powder; ESI-MS m/z : 465 [M+H]+; 1H-NMR (300 MHz, CD3OD): δ 6.92 (2H, s, H-2′/6′), 6.32 (1H, d, J = 2.1 Hz, H-8), 6.16 (1H, d, J = 2.1 Hz, H-6), 5.29 (1H, d, J = 1.1 Hz, H-1″), 4.19 – 3.30 (4H, m, H-2″/3″/4″/5″), 0.94 (3H, d, J = 6.3 Hz, H-6″); 13CNMR (75 MHz, CD3OD): δ 179.7 (C-4), 166.0 (C-7), 163.2 (C-5), 159.5 (C-2), 158.6 (C-9), 146.9 (C-3′/5′), 138.0 (C-4′), 136.4 (C-3), 122.0 (C-1′), 109.7 (C-2′/6′), 105.9 (C-10), 103.7 (C-1″), 99.9 (C-6), 94.8 (C-8), 73.4 (C-4″), 72.2 (C-3″), 72.1 (C-5″), 72.0 (C-2″), 17.7 (C-6″).
Yellow powder; ESI-MS m/z : 617 [M+H]+; 1H-NMR (300 MHz, CD3OD): δ 6.96 (2H, s, galloyl-H), 6.93 (2H, s, H-2′/6′), 6.34 (1H, d, J = 2.1 Hz, H-8), 6.18 (1H, d, J = 2.1 Hz, H-6), 5.30 (1H, d, J = 1.1 Hz, H-1″), 4.21–3.30 (4H, m, H-2″/3″/4″/5″), 0.94 (3H, d, J = 6.3 Hz, H-6″); 13C-NMR (75 MHz, CD3OD): δ 178.7 (C-4), 166.7 (COO), 165.2 (C-7), 162.5 (C-5), 159.7 (C-2), 157.8 (C-9), 146.2 (C-3′/5′), 145.7 (C-3‴/5‴), 139.3 (C-4‴), 137.4 (C-4′), 135.4 (C-3), 121.4 (C-1′), 121.2 (C-1‴), 109.9 (C-2′/6′), 109.1 (C-2‴/6‴), 105.4 (C-10), 99.5 (C-6), 99.4 (C-1″), 94.2 (C-8), 73.4 (C-4″), 72.8 (C-2″), 71.7 (C-5″), 70.2 (C-3″), 17.1 (C-6″).
Yellow powder; ESI-MS m/z : 617 [M+H]+; 1H-NMR (300 MHz, CD3OD): δ 6.98 (2H, s, galloyl-H), 6.94 (2H, s, H-2′/6′), 6.35 (1H, d, J = 2.1 Hz, H-8), 6.17 (1H, d, J = 2.1 Hz, H-6), 5.31 (1H, s, H-1″), 4.21–3.30 (4H, m, H-2″/3″/4″/5″), 0.94 (3H, d, J = 6.3 Hz, H-6″); 13C-NMR (75 MHz, CD3OD): δ 179.2 (C-4), 168.2 (COO), 165.4 (C-7), 162.8 (C-5), 159.1 (C-2), 158.2 (C-9), 146.4 (C-3′/5∼), 146.1 (C-3‴/5‴), 139.5 (C-4‴), 137.5 (C-4′), 136.2 (C-3), 121.7 (C-1‴), 121.4 (C-1′), 109.9 (C-2′/6′), 109.5 (C-2‴/6‴), 105.6 (C-10), 103.2 (C-1″), 99.5 (C-6), 94.4 (C-8), 75.4 (C-4″), 71.8 (C-2″), 70.7 (C-3″), 69.4 (C-5″), 17.1 (C-6″).
Yellow powder; ESI-MS m/z : 433 [M+H]+; 1H-NMR (300 MHz, CD3OD): δ 7.75 (2H, d, J = 8.1 Hz, H-2′/6′), 6.93 (2H, d, J = 8.1 Hz, H-3′/5′), 5.38 (1H, brs, H-1″), 4.24 (1H, brs, H-2″), 3.72 (1H, brs, H-3″), 3.33 (2H, m, H-4″/5″), 0.93 (3H, s, H-6″); 13C-NMR (75 MHz, CD3OD): δ 179.5 (C-4), 163.1 (C-7), 161.6 (C-5), 159.2 (C-2/4′), 159.6 (C-9), 136.2 (C-3), 131.9 (C-2′/6′), 122.7 (C-1′), 116.6 (C-3′/5′), 105.6 (C-10), 103.6 (C-1″), 99.9 (C-6), 95.2 (C-8), 73.3 (C-4″), 72.2 (C-3″), 72.1 (C-5″), 72.0 (C-2″), 17.7 (C-6″).
Yellow powder; ESI-MS m/z : 443 [M+H]+; 1H-NMR (300 MHz, CD3OD): δ 7.04 (1H, d, J = 1.8 Hz, H-2′), 7.01 (2H, s, H-2″/6″), 6.90 (1H, dd, J = 7.8, 1.8 Hz, H-2′), 6.02 (1H, d, J = 1.8 Hz, H-6), 6.06 (1H, d, J = 1.8 Hz, H-8), 5.52 (1H, m, H-3), 5.10 (1H, d, J = 7.5 Hz, H-2), 3.02 (1H, dd, J = 16.2, 4.0 Hz, H-4ax), 2.91 (1H, dd, J = 16.4, 3.4 Hz, H-4eq); 13CNMR (75 MHz, CD3OD): δ 166.4 (COO), 157.8 (C-9), 157.5 (C-7), 156.8 (C-5), 146.2 (C-3″/5″), 145.8 (C-4′), 145.5 (C-3′), 139.0 (C-4″), 131.4 (C-1′), 121.3 (C-1″), 119.2 (C-5′), 115.3 (C-3′), 114.8 (C-2′), 109.4 (C-2″/6″), 98.7 (C-10), 96.7 (C-8), 95.3 (C-6), 77.8 (C-2), 69.3 (C-3), 26.8 (C-4).
Yellow powder; ESI-MS m/z : 601 [M+H]+; 1H-NMR (300 MHz, DMSO-d6) δ 1H-NMR (300 MHz, CD3OD): 7.38 (1H, d, J = 2.0 Hz, H-2′), 7.32 (1H, dd, J = 2.0, 8.0 Hz, H-6′), 7.10 (2H, s, H-2‴/6‴), 6.94 (1H, d, J = 8.0 Hz, H-5′), 6.34 (1H, d, J = 2.1 Hz, H-8), 6.22 (1H, d, J = 2.1 Hz, H-6), 5.64 (1H, d, J = 1.5 Hz, H-1″), 5.52 (1H, t, J = 2.0 Hz, H-2″), 4.02–3.40 (3H, m, H-3″, 4″, 5″), 0.98 (3H, d, J = 6.0 Hz, H-6″); 13C-NMR (75 MHz, DMSO-d6): δ 179.5 (C-4), 167.5 (C-7), 166.0 (C-7‴), 163.3 (C-5), 159.4 (C-9), 158.6 (C-2), 150.0 (C-4′), 146.6 (C-3‴/5‴), 146.5 (C-3′), 140.1 (C-4‴), 135.8 (C-3), 123.0 (C-6′), 122.9 (C-1′), 121.4 (C-1‴), 117.0 (C-5′), 116.6 (C-2′), 110.5 (C-2‴/6‴), 106.0 (C-10), 100.6 (C-6′), 100.0 (C-1″), 94.8 (C-8), 73.9 (C-4″), 73.6 (C-2″), 72.3 (C-5″), 70.8 (C-3″), 17,9 (C-6″).
White powder; ESI-MS m/z : 199 [M+H]+; 1H-NMR (300 MHz, CD3OD): δ 6.89 (2H, s, H-2/6), 4.14 (2H, q, J = 7.3 Hz, H-8), 1.21 (3H, t, J = 7.3 Hz, H-9); 13C-NMR (75 MHz, CD3OD): δ 168.7 (COO), 146.6 (C-3/5), 139.7 (C-4), 121.8 (C-1), 110.1 (C-2/6), 61.8 (C-8), 14.8 (C-9).
In accordance with a well-established method, a reaction mixture of bovine serum albumin (10 mg/mL, 700 µL; Sigma) in 50mM phosphate buffer (pH 7.4) with 0.02% sodium azide was added to 0.2M fructose and glucose (100 µL). In screw-cap tubes (1.5 mL), the reaction mixture was then mixed with 200 µL of serially diluted compounds or AG (99% purity; Sigma). After incubating at 37 ℃ for 7 days, the fluorescent reaction products (200 µL) were transferred to 96-well plates and assayed on a spectrofluorometric detector (Synergy HT; excitation wavelength, 350 nm, emission wavelength, 450 nm). The AGE assay was performed in triplicate. The IC50 values were obtained by regression analysis using the GraphPad 5.0 Prism software.
All experimental protocols for animal care and use were approved by the local ethics board (South Korean Institute of Oriental Medicine Animal Care and Use Committee) Committee, and animal husbandry and procedures were performed in accordance with institutional guidelines. Adult zebrafish were maintained under standard conditions at 28.5 ℃ under a 14 h light/10 h dark cycle. Embryos were obtained from crosses between flk:EGFP transgenic fish and raised in egg water (sea salt, 0.06 g/L). One-dayold flk:EGFP embryos were placed in a 24-well plate (five embryos per well) and maintained in 2 mL egg water containing 130 mM glucose. HG-induced embryos were treated with 6, 7, and 11 (10 or 20 µM) from 1 to 6 days post-fertilization (dpf). At 6 dpf, HG-induced embryos were fixed with 4% paraformaldehyde, and the lens containing the hyaloid-retinal vessels was isolated. Fluorescence was visualized using the Olympus SZX16 stereomicroscope, and diameters were measured using ImageJ software. Experiments were performed in triplicate.
EtOH extracts of leaves of H. riparia showed greater than 70% inhibition on AGE formation in vitro at a concentration of 10 µg/mL. Thus, this extract was further fractionated using n-hexane, EtOAc, and n-BuOH to isolate components showing strong inhibitory effects on AGE formation. These fractions were tested for their inhibitory effects on AGE formation in vitro; the EtOAcsoluble fraction showed the most potent inhibitory activity on AGE formation, with an IC50 value of 4.3 µg/mL, followed by the n-BuOH fraction (IC50 = 5.8 µg/mL), water fraction (IC50 = 8.5 µg/mL), and n-hexane fraction (IC50 > 50 µg/mL), respectively. Thus, the EtOAc-soluble fraction was subjected to a series of chromatographic separation steps guided by the inhibitory activity on AGE formation, leading to isolation of compounds 1 – 15. Based on a comparison of the spectral data of these compounds with those of compounds in the literature, the following isolates were identified: amentoflavone (1),9 astragalin (2),10 avicularin (3),11 quercitrin (4),12 hyperoside (5),13 (−)-epigallocatechin-3-O-gallate (6),14 corilagin (7),15 myricetin-3-O-glucoside (8),16 myricitrin (9),17 desmanthin-1 (10),18 desmanthine-2 (11),18 afzelin (12),19 (−)-epicatechin-3-O-gallate (13),14 quercitrin gallate (14),20 and ethyl gallate (15).21 All of these isolates are phenolic compounds, a class of chemicals consisting of a hydroxyl group bonded directly to an aromatic hydrocarbon group. These phenolic compounds are classified into a simple phenol [ethyl gallate (15)], flavonoids [amentoflavone (1), astragalin (2), avicularin (3), quercitrin (4), hyperoside (5), myricetin-3-O-glucoside (8), myricitrin (9), desmanthin-1 (10), desmanthine-2 (11), afzelin (12), and quercitrin gallate (14)], a condensed tannin [corilagin (7)], and hydrolysable tannins [(−)-epigallocatechin-3-O-gallate (6) and (−)-epicatechin-3-O-gallate (13)].
The inhibitory effects of the isolated compounds (1 – 15) on AGE formation in vitro were examined, and the results are presented in Table 1. The majority of these compounds, excluding ethyl gallate (15), markedly inhibited AGE formation, with IC50 values of 2.2 – 89.9 µM, compared with the positive control aminoguanidine (AG, IC50 = 962.3 µM), which was the first AGE inhibitor used for the treatment of diabetic nephropathy [22]. Of the compounds evaluated, a condensed tannin, corilagin (7), showed the strongest inhibitory activity against AGE formation, with an IC50 value of 2.2 ± 0.2 µM. Two hydrolysable tannins, (−)-epigallocatechin-3-O-gallate (6) and (−)-epicatechin-3-O-gallate (13), also showed significant AGE formation inhibition, with IC50 values of 15.1 ± 1.1 and 23.1 ± 1.3 µM, respectively. Flavonoids with the catechol or pyrogallol moiety in the B-ring, such as avicularin (3), quercitrin (4), hyperoside (5), myricetin-3-O-glucoside (8), myricitrin (9), desmanthin-1 (10), desmanthine-2 (11), and quercitrin gallate (14), showed considerable AGE formation inhibition, with IC50 values of 5.0 – 34.4 µM, while the other flavonoids, amentoflavone (1), astragalin (2), and afzelin (12), which do not contain a catechol or pyrogallol moiety in the B-ring showed less potent activities, with IC50 values of 58.9 – 89.9 µM. Since the presence of catechol or pyrogallol unit(s) in the B-ring of flavonoids is essential to induce significant antioxidant activity, our finding is consistent with previous reports that the inhibitory activity against AGE formation of several flavonoids were closely related to their antioxidant capacities.2324
The accumulation of AGEs in retinal blood vessels is one of the major etiological factors contributing to diabetic retinopathy. Previous animal studies have shown that exposure to high levels of AGEs contribute to vascular complications.2526 The zebrafish is emerging as an excellent vertebrate model for studying embryonic vascular development. Thus, the hydrolysable tannin (−)-epigallocatechin-3-O-gallate (6), the condensed tannin corilagin (7), and the flavonoid desmanthine-2 (11), which potently inhibit AGE formation, were examined for their effects on retinopathy in vivo in a diabetic zebrafish model. The change in hyaloid-retinal vessel dilation was assessed in transgenic zebrafish embryos expressing EGFP in the vasculature (flk:EGFP) under HG conditions. Based on a toxicity test conducted in zebrafish, none of the compounds evaluated were toxic at concentrations up to 20 µM (data not shown). Thus, to evaluate the effects of compounds 6, 7, and 11 on HG-induced dilation of hyaloid-retinal vessels, flk:EGFP transgenic zebrafish embryos were treated with 10 and 20 µM of these compounds. HG-induced hyaloid-retinal vessels treated with 6, 7, and 11 were significantly thinner than those of the HG-treated control group (Fig. 2.B–2.H). These compounds showed decreases in HG-induced hyaloid-retinal vessel diameter of 62.4 – 82.9% at 10 ìM and 84.1 – 94.2% at 20 µM compared with the HG-treated control group (Fig. 2.I).
The formation of AGEs, the products of non-enzymatic glycation and oxidation of proteins and lipids, has been considered an important pathophysiological mechanism in the development of diabetic complications. AGEs accumulate in various tissues at accelerated rates under diabetic conditions27 and have been implicated in the development and progression of numerous complications associated with diabetes, such as neuropathy, nephropathy, angiopathy, and retinopathy.28 Because of the potential role of AGEs in vascular complications, the development and investigation of AGE inhibitors, especially natural anti-AGE agents without adverse effects, may provide a therapeutic approach for delaying or preventing diabetic complications.29 In the current study on effective agents for the treatment of diabetic complications derived from H. riparia leaves, 15 phenolic compounds were isolated. In the AGE assay, compounds 3 – 11, 13, and 14 significantly inhibited AGE formation, with IC50 values of 4.9 – 34.4 µM. In addition, the hydrolysable tannin (−)-epigallocatechin-3-O-gallate (6), the condensed tannin corilagin (7), and the flavonoid desmanthine-2 (11), significantly decreased the dilation of HG-induced hyaloidretinal vessels in a diabetic zebrafish model. These results suggest that H. riparia and its active compounds have beneficial uses in the development of therapeutic and/or preventative agents for diabetic vascular complications and other related diseases.
Figures and Tables
Fig. 2
Effects of compounds 6, 7, and 11 on dilation of hyaloidretinal vessels in a high-glucose (HG)-induced diabetic retinopathy model. Hyaloid-retinal vessels of flk:EGFP transgenic zebrafish from the following groups: (A) untreated normal (NOR); (B) HG-treated control (HG); HG-treated specimens receiving (C) 10 µM, and (D) 20 µM compound 6; HG-treated specimens receiving (E) 10 µM, and (F) 20 µM compound 7; HG-treated specimens receiving (G) 10 mM, and (H) 20 mM compound 11. (I) Data are displayed as mean artificial units (AU) for vessel diameters. The diameters of hyaloid-retinal vessels were measured at locations proximal to the optic disc (red circle). Scale bar = 50 µm. The hyaloid vessel diameter of each lens was measured three times and the experiment was performed in triplicate. ###p < 0.001 vs. NOR, *p < 0.05 vs. HG, **p < 0.01 vs. HG, ***p < 0.001 vs. HG.
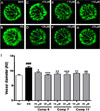
Table 1
Inhibitory effects of compounds 1 – 15 from the leaves of H. riparia against AGE formationa
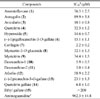
Acknowledgments
This research was supported by the Korea Institute of Planning and Evaluation for Technology in Food, Agriculture, Forestry and Fisheries (IPET) funded by the Ministry of Agriculture, Food and Rural Affairs (316023-05-2-CG000) and the Korea Institute of Oriental Medicine (K17270). The NMR and MS experiments were performed by the Korea Basic Science Institute (KBSI).
References
1. Harvey A. IDrugs. 2010; 13:70–72.
2. Carlson EE. ACS Chem Biol. 2010; 5:639–653.
3. Siddiqui S, Verma A, Rather AA, Jabeen F, Meghavansi MK. Adv Biol Res. 2009; 3:188–195.
4. Warrier PK, Nambiar VPK, Ramankutty C. Indian Medicinal Plants: A compendium of 500 species, vol. 3. Chennai: Orient Longman Ltd.;1994. p. 172.
5. Parveen N, Singh MP, Khan NU. J Indian Chem Soc. 1988; 65:815–816.
6. Viswanadh GS, Atchuta Ramaiah P, Laatsch H, Maskey R. J Trop Med Plant. 2006; 7:267–273.
7. Yang SM, Liu XK, Qing C, Wu DG, Zhu DY. Acta Pharm Sin. 2007; 42:292–296.
8. Lee I, Kim J, Kim YS, Yoo NH, Kim CS, Jo K, Kim JH, Bach TT, Kim JS. J Nat Prod. 2012; 75:1312–1318.
9. Shin DI, Kim J. Korean J Pharmacogn. 1991; 22:207–210.
10. Dai D, He J, Sun R, Zhang R, Aisa H, Abliz Z. Anal Chim Acta. 2009; 632:221–228.
11. Kim HJ, Woo ER, Park H. J Nat Prod. 1994; 57:581–586.
12. Kim YK, Kim YS, Choi SU, Ryu SY. Arch Pharm Res. 2004; 27:44–47.
13. Zhou T, Chen B, Fan G, Chai Y, Wu Y. J Chromatogr A. 2006; 1116:97–101.
14. Lee SH, Kim SY, Kim JJ, Jang TS, Chung SR. Korean J Pharmacogn. 1999; 30:397–403.
15. Okuda T, Yoshida T, Nayeshiro H. Chem Pharm Bull. 1977; 25:1862–1869.
16. Sotnikova OM, Litvinenko VI. Chem Nat Compd. 1968; 4:42–43.
17. Chung SK, Kim YC, Takaya Y, Terashima K, Niwa M. J Agric Food Chem. 2004; 52:4664–4668.
18. Nicollier G, Thompson AC. Flavonoids of Desmanthus illinoensis. J Nat Prod. 1983; 46:112–117. http://pubs.acs.org/doi/pdf/10.1021/np50025a011.


19. Fukunaga T, Nishiya K, Kajikawa I, Watanabe Y, Suzuki N, Takeya K, Itokawa H. Chem Pham Bull. 1988; 36:1180–1184.
20. Park WY. Korean J Pharmacogn. 1996; 27:212–218.
21. Sato Y, Oketani H, Singyouchi K, Ohtsubo T, Kihara M, Shibata H, Higuti T. Biol Pharm Bull. 1997; 20:401–404.
22. Brownlee M, Vlassara H, Kooney A, Ulrich P, Cerami A. Science. 1986; 232:1629–1632.
23. Morimitsu Y, Yoshida K, Esaki S, Hirota A. Biosci Biotechnol Biochem. 1995; 59:2018–2021.
25. Stitt A, Gardiner TA, Alderson NL, Canning P, Frizzell N, Duffy N, Boyle C, Januszewski AS, Chachich M, Baynes JW, Thorpe SR. Diabetes. 2002; 51:2826–2832.
26. Hammes HP, Weiss A, Fuhrer D, Krämer HJ, Papavassilis C, Grimminger F. Diabetologia. 1996; 39:251–255.
27. Brownlee M. Nature. 2001; 414:813–820.
28. Takenaka K, Yamagishi S, Matsui T, Nakamura K, Imaizumi T. Curr Neurovasc Res. 2006; 3:73–77.
29. Reddy VP, Beyaz A. Drug Discov Today. 2006; 11:646–654.