Abstract
Luteolin 5-O-glucoside is the major flavonoid from Korean thistle, Cirsium maackii. We previously reported the anti-inflammatory activities of luteolin 5-O-glucoside in lipopolysaccharide (LPS)-stimulated RAW 264.7 cells. In this study, we determined the anti-inflammatory mechanisms of luteolin 5-O-glucoside through the inhibition of nitric oxide (NO) production in vitro and in vivo. Results revealed that luteolin 5-O-glucoside dose-dependently inhibited NO production and expression of iNOS and COX-2 in LPS-induced RAW 264.7 cells. Luteolin 5-O-glucoside also significantly inhibited the translocation of NF-κB, the activation of MAPKs, and ROS generation in LPS-induced RAW 264.7 cells. In addition, protein expressions of Nrf-2 and HO-1 were also upregulated by luteolin 5-O-glucoside treatment. Moreover, luteolin 5-O-glucoside inhibited λ-carrageenan-induced mouse paw edema by 65.34% and 48.31% at doses of 50 and 100 mg/kg body weight, respectively. These findings indicate potential anti-inflammatory effect of luteolin 5-O-glucoside particularly by downregulating NF-κB and upregulating HO-1/Nrf-2 pathway.
Inflammation is an essential biological response of the body to many stimuli and leads to the release of proinflammatory cytokines and mediators. Various factors such as microbial infections, chemicals, and immunological reactions are associated with inflammation. Although inflammation is a beneficial host response to tissue injury, it may result in chronic conditions such as cancer, cardiovascular disease, diabetes, pulmonary disorders, neurological disease, and arthritis.1 The suppression of inflammatory mediators or signaling pathways including nitric oxide (NO), inducible nitric oxide synthase (iNOS), cyclooxygenase (COX-2), prostaglandin E2 (PGE2), nuclear factor kappa B (NF-κB), mitogen-activated protein kinase (MAPK), reactive oxygen species (ROS), and proinflammatory cytokines (tumor necrosis factor [TNF]-α, interleukin [IL]-6, and IL-1β) are the treatment focus points of inflammatory diseases.2 The transcription factor NF-κB plays a vital role in inflammatory and acute response.3 In general, NF-κB subunits are inactive and bound to IκB inside the cytoplasm. Phosphorylation of IκB releases NF-κB, allowing it to enter the nucleus and activate gene expression.4 NF-κB is a central regulator of proinflammatory gene expression and mediates the synthesis of cytokines such as TNF-α, IL-1β, IL-6, and IL-8.5 It also controls the transcription of other inflammatory mediators such as COX-2 and iNOS.5 Activation of NF-κB is also controlled by cellular kinases such as MAPKs and phosphatidylinositol 3-kinase (PI3K)/protein kinase B (Akt) signaling pathways. MAPKs, extracellular signal-regulated kinase (ERK), c-Jun N-terminal kinase (JNK), and p38 MAPK have been associated with the transcriptional regulation of inflammatory genes via NF-κB activation.6 Heme oxygenase-1 (HO-1), an inducible and essential enzyme in heme catabolism, degrades heme and produces biliverdin, ferrous iron, and carbon monoxide. These products contribute to the anti-inflammatory effects of HO-1.7 Recent studies showed that HO-1 inhibits the excessive production of proinflammatory cytokines as well as ROS in LPS-stimulated RAW264.7 cells.8 HO-1 induction is regulated at the transcriptional level by a transcription factor called nuclear transcription factor-E2-related factor 2 (Nrf-2). Nrf-2 contributes to the anti-inflammatory process through regulation of HO-1 gene expression; therefore, it has become a drug target for the treatment of inflammatory diseases.9
Cirsium is a genus of perennial and biennial flowering plants commonly known as thistles. Cirsium maackii is a perennial thistle and a member of the Asteraceae family that grows abundantly in Korea. Traditionally, the roots or whole plants of Cirsium species have been used as a folk medicine in the treatment of hemorrhage, inflammation of the liver and kidney, and a variety of abdominal and intestinal disorders.10 Luteolin 5-O-glucoside was isolated previously from C. magofukui and C. sieboldii, and considered to be a rare flavonoid due primarily to its stereochemical hindrance between the hydroxyl group at the 5-position and the adjacent carbonyl group at the 4-position.11 Interestingly, Luteolin 5-O-glucoside is a flavonoid that can be isolated from botanical sources such as C. maackii in large quantity.12 We have previously reported that luteolin 5-O-glucoside exhibits antiinflammatory activity through the inhibition of NO production, iNOS, and COX-2 protein expression in LPS-treated RAW 264.7 cells.13 Although these effects of luteolin 5-O-glucoside have been evaluated, the complete regulation mechanism of inflammatory signaling pathways in vitro and the anti-inflammatory effects of luteolin 5-O-glucoside in vivo remain to be evaluated. Therefore, the objective of this study was to evaluate the effects of luteolin 5-O-glucoside on the downregulation of inflammatory mediators such as NO, iNOS, COX-2, NF-κB, and MAPKs, and upregulation of HO-1 and Nrf-2 protein expression in vitro and the in vivo anti-inflammatory activity using a carrageenan-induced mouse paw edema model.
Male ICR mice (male, 25 - 30 g, 10 weeks old) were purchased from the Samtako Bio Korea (Gyeonggido, Korea). All animal experiments were conducted according to experimental protocols and procedures approved by the Animal Ethics Committee of Pukyong National University (approval number: 2013–05). All animals were acclimated for at least one week, caged as seven per group, and fed a diet of animal chow and water ad libitum. The animals were housed at 23 ± 0.5 ℃ and 10% humidity with a 12-h light-dark cycle.
LPS from Escherichia coli, Griess reagent, 3-[4,5-dimethylthiazol-2-yl]-2,5-di-phenyl tetrazolium bromide (MTT), dimethyl sulfoxide (DMSO), 2′,7′-dichlorodihydrofluorescein diacetate (DCFH-DA), 2-amino-5,6-dihydro-6-methyl-4H-1,3-thiazine hydrochloride (AMT), 6-hydroxy-2,5,7,8-tetramethylchroman-2-carboxylic acid (Trolox), phenylmethylsulfonyl fluoride (PMSF), fetal bovine serum (FBS) and antibiotics were purchased from Sigma-Aldrich Co. (St. Louis, MO, USA) and Dulbecco's modified Eagle's medium (DMEM) was from Hyclone (Logan, UT, USA). Various primary antibodies (against iNOS, COX-2, NF-κB [p65], p-ERK, ERK, p-JNK, JNK, p-p38, p38, HO-1, Nrf-2, and β-actin) and secondary antibodies were obtained from Cell signaling Technology Inc. (Beverly, MA, USA) and Santa Cruz Biotechnology, Inc., Santa Cruz, CA, USA) and diluted 1:1,000. Polyvinylchloride fluoride (PVDF) membrane (Immobilon-P) was from Millipore Co. (Billerica, MA, USA). Super-signal® West Pico Chemiluminescent Substrate was from Pierce Biotechnology, Inc. (Rockford, IL, USA). All other chemicals and solvents were purchased from Sigma-Aldrich Co., unless stated otherwise.
We isolated luteolin 5-O-glucoside from C. maakii leaves following the method described by Jung et al.13 The chemical structure of luteolin 5-O-glucoside was elucidated on the basis of spectroscopic evidence including 1H and 13CNMR and comparison with published spectra data, and purity was determined by an HPLC chromatogram.13
Murine RAW 264.7 macrophage cells were obtained from the American Type Culture Collection (ATCC Rockville, MD, USA). RAW 264.7 cells were cultured in DMEM supplemented with 10% heat-inactivated FBS, 1% penicillin-streptomycin, and 0.1% amphotericin B. The cells were incubated in a humidified atmosphere with 5% CO2 at 37 ℃.
Cell viability was assessed using the MTT assay. Briefly, RAW 264.7 cells were seeded into 96-well plates at a density of 1 × 105 cells per well and incubated at 37 ℃ for 24 h. The cells were then treated with the test sample at various concentrations. After incubation for an additional 24 h at 37 ℃, 100 µL MTT (0.5 mg/ml in PBS) was added to each well and incubation continued for another 2 h. The resulting color was assayed at 540 nm using a microplate spectrophotometer (Molecular Devices, Sunnyvale, CA, USA).
The nitrite concentration in the medium was measured using Griess reagent as an indicator of NO production. Briefly, RAW 264.7 cells (2 × 105 cells/well in a 24-well plate with 500 µL culture medium) were pretreated with various concentrations of the test sample for 2 h and then incubated for 18 h with LPS (1 µg/mL). After incubation, the nitrate concentration of the supernatants (100 µL/well) was measured by addition of 100 µL Griess reagent. The absorbance values of mixtures were determined using a microplate spectrophotometer (Molecular Devices) at 540 nm. The iNOS inhibitor, AMT was used as a positive control.
The intracellular ROS scavenging activity of luteolin 5-O-glucoside was measured using the fluorescent probe DCFH-DA. Cells were plated in a black 96-well plate at a density of 1 × 105 cells/well and co-treated with various concentrations of the compound and LPS (1 µg/mL) for 2 h. Cells were treated with 20 µM DCFH-DA for 30 min at 37 ℃. The fluorescence intensity was measured at an excitation wavelength of 485 nm and an emission wavelength of 528 nm using a fluorescence microplate reader (Dual Scanning SPECTRAmax, Molecular Devices).
Western blotting was performed to measure the protein expression of iNOS, COX-2, MAPKs, HO-1, and Nrf-2. First, RAW 264.7 cells (5 × 105 cells/mL) were cultured in 100-mm culture dishes in the presence or absence of LPS (1.0 µg/mL) and with or without test sample for 18 h. After treatment, the cells were washed twice with ice-cold PBS and lysed with cell lysis buffer (50mM Tris-HCl, 150 mM NaCl, 1% Nonidet P-40, 1% Tween 20, 0.1% SDS, 1 mM Na3VO4, 10 µg/mL leupeptin, 50 mM NaF, and 1 mM PMSF, pH 7.5) on ice for 30min. Cell extracts were obtained by centrifugation at 14,000 × g at 4 ℃ for 20 min. Protein amount was determined by Protein Bradford assay. Proteins were separated by SDS-PAGE and transferred to PVDF membranes. Membranes were immediately blocked with nonfat dry milk (50 g/L) in Tris-buffered saline containing 0.1% Tween-20 (pH 7.4) (TBST) buffer at room temperature for 1 h. The membranes were then washed three times (10 min each) in TBST buffer and incubated with primary antibody diluted 1:1,000 in nonfat dry milk (50 g/L) in TBST buffer at 4 ℃ overnight. After three washes with TBST buffer (10min), the membranes were incubated with a horseradish peroxidase (HRP)-conjugated secondary antibody diluted 1:2,000 in nonfat dry milk (50 g/L) in TBST buffer at room temperature for 2 h. After three washes in TBST buffer (10 min), antibody labeling was visualized with the Supersignal West Pico Chemiluminescent Substrate (Pierce, Rockford, IL, USA) according to the manufacturer's instructions and exposed to X-ray film (GE Healthcare Ltd., Amersham, United Kingdom). Prestained blue markers were used for molecular weight determination. Bands were quantified by densitometry analysis using an ATTO CS analyzer.
The in vivo anti-inflammatory activity of luteolin 5-O-glucoside was determined using the λ-carrageenan-induced edema test in the hind paws of mice.14 Male ICR mice (seven per group, 25 - 30 g) fasted for 12 h before the experiment with free access to water. A 1% suspension of carrageenan in saline was prepared 30 min before each experiment and 50 µL was injected into the plantar side of the right hind paws of mice. Luteolin 5-O-glucoside and indomethacin were suspended in Tween-80 plus 0.9% (w/v) saline solution. The final concentration of Tween-80 did not exceed 5% and did not cause any detectable inflammation. Luteolin 5-O-glucoside at doses of 50 and 100 mg/kg body weight was administered orally 1 h before carrageenan treatment. Indomethacin was administered orally at a dose of 10 mg/kg 1 h before the carrageenan treatment. The paw volume was measured immediately after carrageenan injection and at 2, 5, and 7 h intervals after the administration of the edematogenic agent using a plenthysmometer (model 7159, Ugo Basile, Varese, Italy). The degree of induced swelling was evaluated using the equation (a-b), where ‘a’ was the volume of the right hind paw after carrageenan treatment and ‘b’ was the volume of the right hind paw before carrageenan treatment. Indomethacin was used as a positive control.
Data were expressed as the mean ± standard deviation (SD) of at least three independent experiments unless otherwise indicated. Data were compared using one-way ANOVA. P values < 0.05 were considered statistically significant. All analyze were performed using SPSS for windows, version 23 (SPSS Inc., Chicago, IL, USA).
The MTT cell viability assay was used to determine the effects of luteolin 5-O-glucoside on RAW 264.7 cells. Cell viability was tested for the appropriate concentration ranges of luteolin 5-O-glucoside. As shown in Fig. 1, luteolin 5-O-glucoside showed no cytotoxicity to RAW 264.7 cells at concentrations of 10, 50, and 100 µM. These non-toxic concentrations were used for all further experiments.
Griess reagent was used for determination of nitrite concentration in the culture media to evaluate the anti-inflammatory activities of luteolin 5-O-glucoside in LPS-stimulated RAW 264.7 cells. Nitrite, a stable metabolite of NO, was used as an indicator of NO production in the medium. During the 18-hour incubation with LPS (1 µg/mL), NO production increased dramatically. As shown in Fig. 2, pretreatment with the luteolin 5-O-glucoside significantly suppressed LPS-stimulated NO production in RAW 264.7 cells. The results clearly indicated that luteolin 5-O-glucoside dose-dependently inhibited LPS-induced NO production in RAW 264.7 cells. AMT, a positive control for iNOS inhibition, significantly inhibited LPS-induced NO production (Fig. 2).
We next used western blot analysis to assess the activity of luteolin 5-O-glucoside on the expression of iNOS and COX-2, which are well characterized markers of NF-κ-Bresponsive inflammation.15 As shown in Fig. 3., iNOS and COX-2 protein expressions in unstimulated RAW 264.7 cells were almost undetectable, but were significantly stimulated upon treatment with 1 µg/mL of LPS. Pretreatment with luteolin 5-O-glucoside at concentrations of 10, 50, and 100 µM significantly downregulated the expression of iNOS protein in a concentration-dependent manner. However, the effect of luteolin 5-O-glucoside against COX-2 protein levels were less pronounced, and only at 100 µM did there appear to be a potential decrease in COX-2.
The effects of luteolin 5-O-glucoside on the transcriptional activation of NF-κB in LPS-induced RAW 264.7 cells were examined by Western blot analysis. Fig. 4, clearly shows that a number of p65 (NF-κB) subunits in the cells rapidly increased after LPS treatment in the control group and this effect was only inhibited in the 100 µM sample treated group. This result revealed that LPS induced the translocation of NF-κB into the nucleus and pretreatment with luteolin 5-O-glucoside slight suppressed this process at higher concentration (Fig. 4).
The phosphorylation levels of MAPKs, a group of signaling molecules that play a vital part in regulating the LPS-induced inflammatory process, were measured in LPS-treated RAW 264.7 cells by Western blot analysis. The phosphorylation of MAPKs is also closely linked to the regulation of NF-κB activation. Fig. 5, shows the inhibitory activities of luteolin 5-O-glucoside on ERK, JNK, and p38 phosphorylation after 2 h of LPS stimulation in RAW 264.7 cells. The results revealed that luteolin 5-O-glucoside significantly inhibited the phosphorylation of ERK in dose dependent manners (Fig. 5A), and mediocre inhibition at a concentration of 100 µM against phosphorylation of JNK, and p38 in LPS-treated RAW 264.7 cells.
Inflammation is mediated by cellular oxidative stress; therefore, we determined the inhibitory effects of luteolin 5-O-glucoside on LPS-induced ROS generation in RAW 264.7 cells. The cells were treated with LPS to generate ROS, and DCFH-DA was added for detection of ROS generation using a fluorescence microplate reader. As shown in Fig. 6, luteolin 5-O-glucoside did not show any inhibitory activity at 10 µM concentration against ROS generation. However, as the concentration of luteolin 5-O-glucoside was increased up to 100 µM, it exhibited significant inhibitory activity against ROS production compared with the positive control (Trolox).
HO-1 is a cytoprotective enzyme that provides cellular protection against oxidative stress. Western blotting was used to evaluate the effects of luteolin 5-O-glucoside on the upregulation of HO-1 protein expression. As shown in Fig. 7A, luteolin 5-O-glucoside at concentrations of 50 and 100 µM induced HO-1 protein exoression, while luteolin 5-O-glucoside at a concentration of 10 µM did not affect HO-1 expression. Therefore, the results revealed that luteolin 5-O-glucoside has the affinity to increased HO-1 protein expression significantly at higher concentration in LPS-induced RAW 264.7 cells (Fig. 7A).
Nrf-2, a transcription factor that regulates the expression of HO-1, provides protection against oxidative damage at the site of inflammation. Western blotting was performed to measure Nrf-2 protein expression in LPS-treated RAW 264.7 cells. As shown in Fig. 8, luteolin 5-O-glucoside significantly upregulated Nrf-2 expression in LPS-treated RAW 264.7 cells. As shown in Fig. 7B, the activation of Nrf-2 was shown by luteolin 5-O-glucoside at a concentration of 100 µM. However, luteolin 5-O-glucoside at concentrations of 10 and 50 µM did not show any noticeable upregulation of Nrf-2. Therefore, the results showed that luteolin 5-O-glucoside at higher concentration significantly upregulates Nrf-2 expression in LPS-treated RAW 264.7 cells.
As in vitro studies of luteolin 5-O-glucoside showed anti-inflammatory effects, we further evaluated the in vivo anti-inflammatory activity of luteolin 5-O-glucoside using a λ-carrageenan-induced mouse paw edema model. Paw edema was markedly attenuated after treatment with luteolin 5-O-glucoside at doses of 100 and 50 mg/kg body weight after 2, 5, and 7 h of λ-carrageenan stimulation. Furthermore, luteolin 5-O-glucoside at a dose of 100 mg/kg body weight reduced paw edema to a greater extent than the positive control group (*p < 0.05) (Fig. 8). Indomethacin is a common clinical NSAID that was used as a positive control; pretreatment with 10 mg/kg of indomethacin significantly reduced paw edema after 5 and 7 h of stimulation by carrageenan (*p < 0.05). Specifically, luteolin 5-O-glucoside at a dose of 100 mg/kg inhibited paw edema by 48.31% after 2, 5, and 7 h of λ-carrageenan stimulation, which was more effective than indomethacin (60.43%) (data not shown).
Cirsium maackii is a herbaceous perennial plant that grows abundantly in Korea, Japan, and China.16 A total of 250 species of thistle have been identified worldwide to date, and about 10 of these species have been found in Korea.17 The whole plant of C. maackii has been used in folk medicine for its anti-inflammatory properties.10 Luteolin 5-O-glucoside is the major compound isolated from C. maackii.13 The present work was undertaken to understand the molecular mechanism of luteolin 5-O-glucoside in the inhibition of inflammatory mediators. We demonstrated the in vitro effects of luteolin 5-O-glucoside on NF-κB and Nrf-2 signaling in LPS-stimulated RAW 264.7 cells and it's in vivo anti-inflammatory activity. These findings indicated that luteolin 5-O-glucoside effectively inhibits the production of NO through suppression of iNOS, COX-2, NF-κB, and MAPK signaling pathways as well as upregulation of Nrf-2/HO-1 signaling pathways. These data indicate that luteolin 5-O-glucoside may be applied as a pharmaceutical agent for prevention of inflammatory disease.
MTT assay is a sensitive, accurate, and colorimetric assay for testing cell metabolic activity and is widely used for the measurement of cytotoxicity.18 Non-toxic concentrations of luteolin 5-O-glucoside that did not show any cytotoxicity against RAW 264.7 cells were determined using the MTT assay. These safe concentrations of luteolin 5-O-glucoside were used for further evaluation of anti-inflammatory activity in LPS-induced RAW 264.7 cells. It has been reported that luteolin 5-O-glucoside did not significantly affect cell viability at concentrations of up to 200 µM.13
Excess production of NO and PGE2 is related to the inflammatory process. Several studies have shown that excess production of NO can lead to inflammatory disorders.1920 Therefore, inhibition of NO and PGE2 production and inhibition of iNOS and COX-2 protein expression might have vital therapeutic value for the prevention of inflammatory diseases. Luteolin 5-O-glucoside inhibited NO production in a dose-dependent manner in LPS-induced RAW 264.7 cells. Our study also showed that luteolin 5-O-glucoside significantly inhibited iNOS and COX-2 protein expression levels at a higher concentration in LPS-stimulated RAW 264.7 cells. Even if NO production was higher at high concentration in compared to positive control (AMT), the significant inhibition was shown in iNOS inhibition. These happened due to cell condition. However, we did not determine levels of PGE2 production. Further studies may be required to examine the inhibition of PGE2 production by luteolin 5-O-glucoside in LPS-induced RAW 264.7 cells.
A number of studies have shown that LPS-induced inflammation is highly related to various intracellular signaling pathways such as NF-κB and MAPK pathways. NF-κB is a vital regulator of a number of proinflammatory genes, including iNOS, COX-2, TNF-α, IL-1β, and IL-6, in LPS-induced inflammation.212223 Generally, the inactive complex of NF-κB with IκB exists in the cytoplasm. IκB is rapidly phosphorylated in response to proinflammatory stimuli, leading to dissociation of the complex.24 Free NF-κB rapidly translocates to the nucleus and promotes the transcription of target genes responsible for inflammation. Therefore, NF-κB could be an operative therapeutic target for the treatment of inflammatory diseases. The present data showed that luteolin 5-O-glucoside has a tendency to inhibit NF-κB protein expression at a higher concentration in LPS-induced RAW 264.7 cells.
ERK1/2, JNK, and p38 are members of the MAPK family that control cellular signal transduction in response to inflammation and ultimately activate NF-κB.25 The mechanism of NF-κB inactivation is linked to inhibition of phosphorylation of ERK1/2, JNK, and p38. Luteolin 5-O-glucoside significantly inhibited the phosphorylation of ERK, and has a tendency to inhibit the phosphorylation of JNK, and p38 at a higher concentration in LPS-stimulated RAW 264.7 cells. Furthermore, luteolin 5-O-glucoside more strongly inhibited the phosphorylation of ERK compared to that of JNK and p38.
ROS generation is an important factor in the pathogenesis of inflammation because it can damage cellular components such as cellular macromolecules, DNA, lipids, and proteins.26 LPS increases the cellular ROS levels, which are related to NF-κB-dependent inflammatory signaling pathways.27 Several studies revealed that oxidative stress is associated with NF-κB activation.28 It is also reported that antioxidants can inhibit the production of proinflammatory cytokines including IL-8.29 Our studies revealed that luteolin 5-O-glucoside significantly reduces LPS-activated ROS production at a 100 µM concentration in RAW 264.7 cells.
Recently, the inducible HO-1 enzyme was reported to be a stress-responsive protein.30 HO-1 produces iron, carbon monoxide, and biliverdin through the catalysis of heme, and biliverdin is subsequently converted into bilirubin, which acts as a strong antioxidant. A number of studies indicated that the induction of HO-1 suppresses LPS-induced inflammatory response and activates the Nrf-2 pathway.3132 Activation of Nrf-2 is the primary defense mechanism against cellular oxidative stress. Nrf-2 translocates into the nucleus after detachment from keap1 and binds to antioxidant response elements (AREs) to regulate the transcription of HO-1 protein in LPS-induced RAW 264.7 cells.33 Inducible HO-1 inhibits the excess production of TNF-α and IL-1β through Nrf-2 activation.34 In this study, we found that luteolin 5-O-glucoside significantly upregulates both HO-1 and Nrf-2 protein expression in LPS-induced RAW 264.7 cells.
λ-Carrageenan-induced edema is a widely used inflammation model for assessing the protective activity of natural products against the pathological changes associated with acute inflammation. Acute inflammation can be induced by carrageenan, which increases infiltration of phagocytes and oxidative stress as well as the release of inflammatory mediators such as TNF-α and NO.3536 In vivo experiments showed that carrageenan stimulates local inflammation and induces edema in paw tissues. Pretreatment with luteolin 5-O-glucoside at doses of 100 and 50 mg/kg body weight markedly attenuated paw edema at different time intervals after carrageenan stimulation. Based on both in vitro and in vivo experiments, the potent anti-inflammatory activity of luteolin 5-O-glucoside may contribute, at least in part, to inhibition of ROS generation and the release of pro-inflammatory mediators via the NF-κB and MAPK signaling pathway and to upregulation of HO-1/Nrf-2 signaling pathways.
It has been reported that natural flavonoids have anti-inflammatory effects in mammalian cells, which may be due to their ability to suppress the production of proinflammatory cytokines and mediators.37 It has also found that some flavonoids inhibit chronic inflammation in animal models.38 Flavonoids have long been put to use in Chinese medicine as an anti-inflammatory agent in the form of crude plant extracts. However, their overall function in vivo has yet to be clarified. In addition, it has been proven that a small fraction of ingested dietary flavonoids is absorbed in either the aglycone or glycoside form, while the major part is extensively degraded into different phenolic acids or carbon dioxide.39 It might be expected that the absorbed flavonoids and their metabolites display an in vivo anti-inflammatory activity. Therefore, it has suggested that flavonoids must be administered orally in their glycosidic form in order to be applied as anti-inflammatory agents.40 Interestingly, it has found that luteolin 5-O-glucoside is the high-yield active flavonoid of C. maackii rather than only luteolin.13 However, in vivo beneficial/harmful effects of luteolin 5-O-glucoside should be investigated further.
In conclusion, the mechanism underlying the anti-inflammatory activity of luteolin 5-O-glucoside was investigated using in vitro and in vivo models. Our findings confirmed that luteolin 5-O-glucoside exhibits anti-inflammatory activity via suppression of NF-κB activation at a higher concentration in RAW 264.7 cells, which in turn suppresses the release of the pro-inflammatory mediators NO, iNOS, and COX-2. In addition, the upregulatory activity of luteolin 5-O-glucoside on the HO-1/Nrf-2 pathway might provide an extra advantage in the treatment of inflammatory diseases. Therefore, understanding the mechanisms of action of the anti-inflammatory activity of luteolin 5-O-glucoside may lead to the development of more operative therapies for the treatment of inflammation-associated diseases.
Figures and Tables
Fig. 1
Cell viability of luteolin 5-O-glucoside measured by the MTT assay. Values represent the mean ± SD of three independent Experiments.
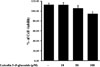
Fig. 2
Inhibitory effects of luteolin 5-O-glucoside on the production of NO in LPS-stimulated RAW 264.7 cells. Cells were pretreated with different concentrations of luteolin 5-O-glucoside for 2 h and then stimulated with LPS (1 µg/ml) for 24 h. The culture media were used to measure the amount of nitrite to determine NO production. Data are presented as mean ± SD of three independent experiments. #p < 0.05 indicates a significant difference from the control group. *p < 0.05 indicates a significant difference from the LPS-treated group.
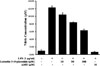
Fig. 3
Inhibitory effects of luteolin 5-O-glucoside on the expression of iNOS and COX-2 in LPS-stimulated RAW 264.7 cells. Cells were pretreated with the indicated concentration of luteolin 5-O-glucoside for 2 h and stimulated with LPS (1 µg/ml) for 18 h. The expression of iNOS, COX-2, and β-actin was detected by Western blotting using corresponding antibodies. The results presented are representative of three independent experiments. #p < 0.05 indicates a significant difference from the control group. *p < 0.05 indicates a significant difference from the LPS-treated group.
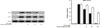
Fig. 4
Inhibitory effects of luteolin 5-O-glucoside on the expression of NF-κB in LPS-stimulated RAW 264.7 cells. Cells were pretreated with the indicated concentration of luteolin 5-O-glucoside for 2 h and stimulated with LPS (1 µg/ml) for 18 h. The expression of NF-κB and β-actin was detected by Western blot using the corresponding antibodies. The results presented are representative of three independent experiments. #p < 0.05 indicates a significant difference from the control group. *p < 0.05 indicates a significant difference from the LPS-treated group.
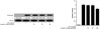
Fig. 5
Inhibitory effects of luteolin 5-O-glucoside on the expression of MAPKs in LPS-stimulated RAW 264.7 cells. Cells were pretreated with the indicated concentration of luteolin 5-O-glucoside for 2 h and stimulated with LPS (1 µg/ml) for 18 h. The expression of MAPKs was detected by Western blotting using corresponding antibodies. The results presented are representative of three independent experiments. #p < 0.05 indicates significant differences from the control group. *p < 0.05 indicates significant differences from the LPS-treated group.
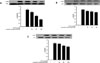
Fig. 6
Inhibitory effects of luteolin 5-O-glucoside on the production of ROS in LPS-stimulated RAW 264.7 cells. Cells were pretreated with different concentration of luteolin 5-O-glucoside for 2 h and then stimulated with LPS (1 µg/ml) for 24 h. ROS levels were measured by fluorescence analysis of DCFH-DA. Data are presented as means ± SDs of three independent experiments. #p < 0.05 indicates a significant difference from the control group. *p < 0.05 indicates a significant difference from the LPS-treated group.
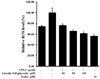
Fig. 7
Inhibitory effects of luteolin 5-O-glucoside on the expression of HO-1 and Nrf-2 in LPS-stimulated RAW 264.7 cells. Cells were pretreated with the indicated concentration of luteolin 5-O-glucoside for 2 h and stimulated with LPS (1 µg/ml) for 18 h. Expression of HO-1 and Nrf-2 was detected by Western blotting using the corresponding antibodies. The results presented are representative of three independent experiments. #p < 0.05 indicates a significant difference from the control group. *p < 0.05 indicates a significant difference from the LPS-treated group.
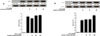
Fig. 8
Effects of luteolin 5-O-glucoside on carrageenan-induced hind paw edema in mice. Luteolin 5-O-glucoside was administered at doses of 25 mg/kg and 50 mg/kg 1 h before injection of carrageenan into the mice to alleviate acute inflammation. Paw volume was measured using a plethysmometer at 2 h, 5 h, and 7 h after carrageenan injection. The increase in paw volume was calculated based on the difference in volume between before carrageenan injection and at different time intervals after carrageenan injection. Values are expressed as the mean ± SD; n = 6 mice per group. *p < 0.05 indicates a significant difference from the vehicle control group. Indomethacin was used as a positive control.
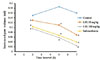
Acknowledgments
This work was supported by a Research Grant of Pukyong National University (2017 year).
References
1. Choudhari AS, Raina P, Deshpande MM, Wali AG, Zanwar A, Bodhankar SL, Kaul-Ghanekar R. J Ethnopharmacol. 2013; 150:215–222.
2. Joung EJ, Lee B, Gwon WG, Shin T, Jung BM, Yoon NY, Choi JS, Oh CW, Kim HR. Int Immunopharmacol. 2015; 29:693–700.
3. Giuliani C, Napolitano G, Bucci I, Montani V, Monaco F. Clin Ter. 2001; 152:249–253.
4. May MJ, Ghosh S. Immunol Today. 1998; 19:80–88.
5. Tak PP, Firestein GS. J Clin Invest. 2001; 107:7–11.
6. Kim AR, Lee MS, Shin TS, Hua H, Jang BC, Choi JS, Byun DS, Utsuki T, Ingram D, Kim HR. Toxicol In Vitro. 2011; 25:1789–1795.
7. Pae HO, Chung HT. Immune Netw. 2009; 9:12–19.
8. Chen TY, Sun HL, Yao HT, Lii CK, Chen HW, Chen PY, Li CC, Liu KL. Food Chem Toxicol. 2013; 55:257–264.
9. Taha R, Blaise G. Funct Food Health Dis. 2014; 4:510–523.
10. Kim JG. Illustrated Natural Drugs Encyclopedia. Korea: Namsandang;1984. p. 37.
11. Iwashina T, Ito T, Ootani S. Ann Tsukuba Bot Gard. 1989; 8:15–19.
12. Jung HA, Kim YS, Choi JS. Food Chem Toxicol. 2009; 47:2790–2797.
13. Jung HA, Jin SE, Min BS, Kim BW, Choi JS. Food Chem Toxicol. 2012; 50:2171–2179.
14. Morris CJ. Methods Mol Biol. 2003; 225:115–121.
16. Lee CB. Flora of Korea. Korea: Hyangmoonsa;1979. p. 274.
17. Lee SJ. Korean Folk Medicine. Korea: Seoul National University;1966. p. 145–146.
18. Keiser K, Johnson CC, Tipton DA. J Endod. 2000; 26:288–291.
19. Meda L, Cassatella MA, Szendrei GI, Otvos L Jr, Baron P, Villalba M, Ferrari D, Rossi F. Nature. 1995; 374:647–650.
20. Dandona P, Chaudhuri A, Dhindsa S. Diabetes Care. 2010; 33:1686–1687.
21. Marks-Konczalik J, Chu SC, Moss J. J Biol Chem. 1998; 273:22201–22208.
22. Islam MN, Choi RJ, Jin SE, Kim YS, Ahn BR, Zhao D, Jung HA, Choi JS. J Ethnopharmacol. 2012; 144:175–181.
23. Chen JJ, Huang WC, Chen CC. Mol Biol Cell. 2005; 16:5579–5591.
24. Rajapakse N, Kim MM, Mendis E, Kim SK. Immunology. 2008; 123:348–357.
25. Kaminska B. Biochim Biophys Acta. 2005; 1754:253–262.
26. Hancock JT, Desikan R, Neill SJ. Biochem Soc Trans. 2001; 29:345–350.
27. Choi SY, Hwang JH, Ko HC, Park JG, Kim SJ. J Ethnopharmacol. 2007; 113:149–155.
28. Siomek A. Acta Biochim Pol. 2012; 59:323–331.
29. Ryan KA, Smith MF Jr, Sanders MK, Ernst PB. Infect Immun. 2004; 72:2123–2130.
30. Kim JH, Choo YY, Tae N, Min BS, Lee JH. Int Immunopharmacol. 2014; 22:420–426.
31. Lee IS, Lim J, Gal J, Kang JC, Kim HJ, Kang BY, Choi HJ. Neurochem Int. 2011; 58:153–160.
32. Paine A, Eiz-Vesper B, Blasczyk R, Immenschuh S. Biochem Pharmacol. 2010; 80:1895–1903.
33. Lee MY, Lee JA, Seo CS, Ha H, Lee H, Son JK, Shin HK. Food Chem Toxicol. 2011; 49:1047–1055.
34. Tsoyi K, Lee TY, Lee YS, Kim HJ, Seo HG, Lee JH, Chang KC. Mol Pharmacol. 2009; 76:173–182.
35. Salvemini D, Wang ZQ, Bourdon DM, Stern MK, Currie MG, Manning PT. Eur J Pharmacol. 1996; 303:217–220.
36. Rocha AC, Fernandes ES, Quintão NL, Campos MM, Calixto JB. Br J Pharmacol. 2006; 148:688–695.
37. Yuan G, Wahlqvist ML, He G, Yang M, Li D. Asia Pac J Clin Nutr. 2006; 15:143–152.
38. Camuesco D, Comalada M, Rodríguez-Cabezas ME, Nieto A, Lorente MD, Concha A, Zarzuelo A, Gálvez J. Br J Pharmacol. 2004; 143:908–918.
39. Halliwell B, Zhao K, Whiteman M. Free Radic Res. 2000; 33:819–830.
40. Comalada M, Ballester I, Bailón E, Sierra S, Xaus J, Gálvez J, de Medina FS, Zarzuelo A. Biochem Pharmacol. 2006; 72:1010–1021.