Abstract
Background
For peripheral nerve regeneration, recent attentions have been paid to the nerve conduits made by tissue-engineering technique. Three major elements of tissue-engineering are cells, molecules, and scaffolds.
Methods
In this study, the attachments of nerve cells, including Schwann cells, on the nerve conduit and the effects of both growth factor and adhesion molecule on these attachments were investigated.
Results
The attachment of rapidly-proliferating cells, C6 cells and HS683 cells, on nerve conduit was better than that of slowly-proliferating cells, PC12 cells and Schwann cells, however, the treatment of nerve growth factor improved the attachment of slowly-proliferating cells. In addition, the attachment of Schwann cells on nerve conduit coated with fibronectin was as good as that of Schwann cells treated with glial cell line-derived neurotrophic factor (GDNF).
Peripheral nerve injuries continue to be among the most challenging problems faced by surgeons. The standard approach to repair a peripheral nerve when a gap is present is to bridge the severed ends with a segment of autologous donor nerve. However, this approach incurs some donor site morbidity and usually involves secondary surgical scars. Researchers in the past have also attempted to bridge severed nerve endings with a wide variety of autologous biological tubular structures, including artery, vein, inside-out vein conduit, skeletal muscle, and decalcified bone channels. Others have used cadaveric nerve allografts or xenografts to bridge long nerve defects [1]. While investigators have achieved promising results using many of these techniques, none of these trials have produced surpassed results achieved by autograft repair [2].
In recent, many artificial nerve conduits made of various materials have been used to study both peripheral nerve system (PNS) and central nerve system (CNS) regeneration [3]. Among them, the nerve conduit fabricated by tissue-engineering technique begins to attract new attentions because it has many advantages over those described above. It can avoid donor site morbidity and secondary surgical scars that are inevitable in autologous graft and it can also decrease graft rejection symptoms compared to allografts and xenografts. Also, the content and structure of the tissue-engineered nerve conduit can be easily modified [4]. We adopted the nerve conduit, a tissue-engineered mixture of ceramic (toothapatite), polymer (polyhydroxybutyrate-co-hydroxyvalerate, PHBV), and chitosan for this study.
It is well known that three major elements of tissue-engineering are cells, molecules, such as growth factor, adhesion molecule and cell signaling molecule, and scaffolds. Focusing the role of cells, growth factors, and adhesion molecules, we examined the attachment of various nerve cells on the nerve conduit and the morphological properties of cell-nerve conduit complexes. The effect of both nerve growth factors and extracellular matrix (ECM) adhesion molecules on cellular attachment was also evaluated in vitro. The previous studies mostly concentrated on only Schwann cells as cellular component in the artificial nerve conduit, however, on the assumption that higher content of nerve cells in the nerve conduit will lead to better peripheral nerve regeneration, PC12 cells (rat, pheochromocytoma), C6 cells (rat, glioma), HS683 cells (human, glioma), as well as Schwann cells were tested in this study. Though most of these cells are originated from tumors, many studies showed them to produce ECM molecules, express many cell adhesion molecules and receptors, and secrete a variety of neurotrophic factors in the process of tumor growth and metastasis. The feasibility of these properties of nerve cells in the peripheral nerve regeneration was investigated in this study.
Tissue-engineered nerve conduit, polymer-ceramic composite scaffolds, composed of polyhydroxybutyrate-co-hydroxyvalerate (PHBV) as a polymer, methylene chloride as a solvent, toothapatite which were made from calcination (950℃ for 1 hr) and pulverization (< 100 µm) of human teeth, and chitosan, was fabricated by a modified solvent casting and particulate leaching method with heat compression.
After gas sterilization with ethylene oxide, this nerve conduit was aseptically prepared as 5-mm cut and affixed to the bottom of a 24-well plate by 5 mg/ml collagen type I (Sigma-aldrich, St. Louis, USA) prepared in 2% acetic acid (Sigma) using its property of gelation beyond room temperature [5]. To minimize cell cytotoxicity, double-sided adhesive tape, mostly used in the previous studies [6], was not employed.
Primary Schwann cells were prepared from dorsal root ganglion (DRG) isolated from 1-day-old Sprague-Dawley rat pups (B&K Universal Ltd, Hull, UK). DRGs were treated with 0.25% collagenase (Gibco, Paisley, UK) and 0.2% DNase (Sigma) and stored in 37℃ for 1.5 hr. They were treated again by addition of 0.125% trypsin-EDTA (Sigma) and the cell mixture was stored in 37℃ for 10 min. After enzymatic solution was removed and feeding medium was added, cells were dissociated by repeated pipetting. The cell suspension was centrifuged for 10 min at 1,000 g. The cell pellet was resuspended in feeding medium, seeded on 75 ml Petri dish coated with poly-D-lysine (Sigma) and maintained in 95% air and 5% CO2 incubator at 37℃. Feeding medium consisted of minimum essential medium (MEM, Gibco), 5% heatinactivated horse serum (HS, Gibco), 10 mg/ml glucose (Sigma), 100 units/ml penicillin and 100 µg/ml streptomycin (Gibco). After 3 days, 5 µM cytosine arabinoside (Sigma) was added to the feeding medium to suppress fibroblast proliferation. And glial cell line-derived neurotrophic factor (GDNF, Gibco) 0.2 µg/ml and cholera toxin (Sigma) 1 µg/ml were added to the feeding medium to improve Schwann cell proliferation after 3 days. The feeding medium was changed once a 2 or 3 days for 2 weeks. Schwann cells were used in passage 2 to 3.
PC12 cells (rat, pheochromocytoma, KCL 21721), C6 cells (rat, glioma, KCL 10107) and HS683 cells (human, glioma, KCL 30138) were prepared from the Korean Cell Line Bank (KCLB). PC12 cell pellets were resuspended and plated on Petri dish in RPMI1640 medium (Gibco) containing 10% heat-inactivated fetal bovine serum (FBS, Gibco) and 5% HS. C6 and HS683 cell pellets were resuspended and plated in Dulbecco's modified Eagle's medium (DMEM, Gibco) containing 10% FBS. They were maintained in 95% air and 5% CO2 incubator at 37℃. The medium was changed once a 2 or 3 days. They were used between passage 3 to 5.
C6 cells and HS683 cells were assigned to Group I, as rapidly-proliferating cells (cell cycle time < 6 hr). PC12 cells and Schwann cells were assigned to Group II, as relatively slowly-proliferating cells (cell cycle time > 96 hr). Group III was Group II treated with growth factor, i.e., PC12 cells treated with nerve growth factor (NGF, Sigma) and Schwann cells with GDNF [7].
The 5 mm-cut nerve conduits were coated with adhesion molecules, tenasin and fibronectin (courtesy of professor Jang JH, Intellectual Biointerface Engineering Center, Seoul National University College of Dentistry) 5, 10, 15 nM, respectively. The content of coated adhesion molecules was confirmed by coomassie blue stain, comparatively measured by optical density (OD) at 595 nm. C6 cells and Schwann cells, selected from Group I and III representatively, were used in these nerve conduits coated with adhesion molecules.
To seed onto the nerve conduit, all the cells were enzymatically lifted using 0.25% trypsin-EDTA, incubated at 37℃ for 1-2 min and resuspended in known amount of medium. Cells were counted with a hemocytometer and diluted to 1X105 cells/ml in the medium. Aliquots of 2 ml of each cell suspension were seeded onto 5 nerve conduits respectively, prepared in a 24-well plate and maintained in 95% air and 5% CO2 incubator at 37℃ for 48 hr.
Cultured cell-nerve conduit complexes were prepared for scanning electron microscope (SEM) studies. They were incubated at low temperature in a fixative of 2.5% glutaraldehyde (Kishida chemicals, Tokyo, Japan) for 20 minutes and then washed twice in phosphate buffered saline (PBS, Gibco) for 10 minutes. And they were longitudinally cut in half to examine both inner and outer side. The complexes were then incubated for 20 minutes in a postfixative of 1% aqueous OsO4 and subsequently washed with PBS for 10 minutes. Samples were dehydrated through ascending ethanol (70, 80, 90, 95 and twice in 100%), allowed to air dry overnight and shadowed with 20 nm Au/Pd. Samples were observed by SEM (JEOL USA Inc, Peabody, MA).
Cell viability through cellular attachment on the nerve conduit was measured quantitatively by MTT (3-(4,5-dimethylthiazol-2-yl)-2,5-diphenyl tetrazolium bromide, Sigma) assay [8]. A 2 mg/ml stock solution of MTT was prepared in PBS and stored in the dark at 4℃ after filtering (0.22 µm). 50 µl of MTT dilution and 200 µl feeding medium were added to cell-nerve conduit complex. After 4 hour incubation, the supernatant was removed and 150 µl DMSO (Sigma) was added. After gentle shaking, 250 µl of solution was transferred to an ELISA microtiter plate and OD was measured at 570 nm.
Fig. 2 shows that the attachment of C6 cells and HS683 cells in Group I and PC12 cells in Group II on the nerve conduit was significantly higher than that of control group (P < 0.05). Only Schwann cells in Group II did not have higher attachment compared to control group.
The attachment of PC12 cells and Schwann cells in Group III was significantly increased compared to that of control group and Group II (P < 0.05, respectively: Fig. 3). There was no statistically significant difference between Group I and Group III. The relationship between cell cycle time, estimated from passage, and cellular attachment on nerve conduit was summarized in Table 1.
All the cells in Group I and II were attached to both inner and outer surfaces as they sustained their own shape (Fig. 4). But in Group III, PC12 cells with NGF treatment were morphologically changed from small and round shape to spindle shape with elongated cytoplasmic extension and reached confluency at the same 48 hr incubation (Fig. 5A and 5C). More elongated cytoplasmic extensons, compared to the Schwann cells in Group II, were also observed in Schwann cells treated with GDNF (Fig. 5B) [9]. The cells in Group I and III attached to the surface of the nerve conduit as they became stratified. In the areas of cell-nerve conduit complexes where cells were confluent, it was difficult to describe cell morphology separately.
Seeded on tenascin-coated nerve conduits, both C6 cells and Schwann cells did not have any difference in cellular attachment compared to none-coated nerve conduit (Fig. 6). On fibronectin-coated nerve conduits, the cellular attachment of Schwann cells on the nerve conduit coated with 15 nM was statistically higher than that of the none-coated nerve conduit (Fig. 7), and there was no statistically significant difference compared to the cellular attachment of Schwann cells treated with GDNF.
The prerequisite of the nerve conduits is that they can provide a controlled environment in which axons can elongate, fibrous tissue invasion is limited and humoral and cellular factors can accumulate [4]. The material used for nerve conduit in this study was originally designed as the accelerator of bone healing. As a mixture of polymer and ceramic, PHBV conferred moldability and biodegradability to this conduit and toothapatite conferred the wettability, reinforcement and biocompatibility. Also, chitosan is biodegradable cationic polyssacharide that is non-toxic, non-immunogenic and acts as a wound healing accelerator [10]. Its unique polarity could release a continuous electrical stimulation during the regrowth of severed axons [8]. Used as a nerve conduit, this material was supposed to act as a conduit allowing axons to grow through without fibrous tissue impeding during the regeneration period.
Schwann cells, mostly employed in the previous studies regarding to peripheral nerve regeneration, are known as neuroglial cells supporting axons in PNS and essential elements for axonal proliferation in peripheral nerve damage. They are also known to be involved in many nerve diseases, such as Guillan-Barré syndrome and neurofibromatosis. In the course of peripheral nerve regeneration, they produce structural and adhesive ECM molecules, express many cell adhesion molecules and receptors, and synthesize and secrete a cocktail of neurotrophic factor [911]. But other nerve cells used in this study, though they are originated from tumors, were found to have the role similar to Schwann cells in the process of tumor growth and invasion and this fact suggests that they should positively effect on peripheral nerve regeneration.
PC12 cells come from adrenal gland tumor and it was established as a neuron study model early in 1980. Recent study shows many genes and their associated molecules, induced after peripheral nerve injury in Schwann cells, are also induced in PC12 cells and PC12 cells treated by NGF [12], so PC12 cells were assumed to play an important role like Schwann cells in the process of nerve regeneration. C6 cells and HS683 cells were firstly used to investigate the brain tumor but now they were widely accepted to study the regulatory mechanisms during neuronal regeneration process [13]. They were firstly applied to the nerve conduits for pheripheral nerve regeneration in this study.
With respects to methodology, the previous studies utilized many kinds of methods to measure cellular attachment on a given material. Trypan blue exclusion test, which was firstly employed, was a reliable method but had a large individual variation according to researchers. It was also not certain whether this test could detach and count all the cells on both inner and outer surface of the conduit. On the principle that dehydrogenase in mitochondria of viable cells changes yellow-colored soluble MTT to purple-colored insoluble MTT, MTT assay is sensitive, accurate, easy and rapid method [14]. It was useful in cell cytotoxicity test which could quantify cell viability. Because in culture system, almost all the nerve cells, including those used in this study, can survive on the condition that they attach to nerve conduit, this test was applied to quantifying cellular attachment on nerve conduit.
Extracellular matrix (ECM), comprised largely of different collagens, proteoglycans, and glycoproteins, is a substrate that transduces and integrates intracellular signals via distinct cell surface receptors [15]. ECM-receptor interactions have a profound influence on major cellular programs, such as degeneration and regeneration. Tenascin was known to play a morphoregulatory role during development, tissue remodeling, and in disease by regulating the cell adhesive and signaling properties of neural and non-neural cells [16]. It is re-expressed in the adult during normal processes such as wound healing, nerve regeneration, and tissue involution, and in pathological states. Many studies showed tenascin had both adhesive and counteradhesive activities according to the condition [17]. In this study, tenascin did not improve the attachment of various nerve cells and the further study to determone the exact condition for tenascin to exert its adhesive activity.
Fibronectin was one of the most commonly used neurite promoting factors. Increased expression of fibronectin was found in damaged peripheral nerve during Wallerian degeneration [18]. When a gel mixture of collagen, laminin, and fibronectin was used to connect the ends of a severed sciatic nerve in the nerve conduit, successful regeneration of nerves across the gap occurred [19]. Fibronectin may facilitate the influx of non-neuronal cells which may provide additional neurotrophic factors for the growth of axons and also promote nerve regeneration by encouraging interactions between developing neuritis and substrata. So fibronectin cannot only improve the attachment of nerve cells on the nerve conduit and also have positive effects on the nerve regeneration if this nerve conduit coated with fibronectin is used.
In the process of peripheral nerve regeneration, nerve growth factors have the potential accelerating and maturating effects [20]. In vivo study, nerve growth factors improved the conductive function of injured peripheral nerve and facilitate regeneration of nerve. But these nerve growth factors are very expensive, so their clinical uses should be limited. As shown in this study, if fibronectin, relatively not so expensive as nerve growth factor, had similar improvement of attachment of nerve cells on the nerve conduit, fibronectin, as an ECM adhesion molecule, could be a good alternative for nerve growth factor in the study of peripheral nerve regeneration.
In conclusion, rapid-proliferating cells, without growth factor, were found to attach on the nerve conduit much better than slow-proliferating nerve cells in this study. Though rapid-proliferating cells are mostly originated from tumor, they could present another experimental utility in peripheral nerve regeneration. Further study will be needed to prove that this higher cellular attachment will lead to better nerve regeneration. Besides, slow-proliferating cells were found to improve cellular attachment on nerve conduits and altered morphological properties when treated with growth factor [21]. This findings supports the concept that growth factor changes nerve cell morphology and affects cell cycle time [2223].
It is noteworthy that Schwann cells did not attach to the nerve conduit so highly as other nerve cells. However, when treated with GDNF, Schwann cells demonstrate improved attachment, not significantly different with that of rapid-dividing cells. It indicates that nerve growth factor or fibronectin treatment is indispensable for Schwann cell to be used for implantation in artificial nerve conduits.
References
1. Siemionow M, Sonmez E. Nerve allograft transplantation: a review. J Reconstr Microsurg. 2007; 23:511–520. PMID: 18189213.


2. Pfister BJ, Gordon T, Loverde JR, Kochar AS, Mackinnon SE, Cullen DK. Biomedical engineering strategies for peripheral nerve repair: surgical applications, state of the art, and future challenges. Crit Rev Biomed Eng. 2011; 39:81–124. PMID: 21488817.


3. Colen KL, Choi M, Chiu DT. Nerve grafts and conduits. Plast Reconstr Surg. 2009; 124(6 suppl):e386–e394. PMID: 19952706.


4. Hadlock T, Elisseeff J, Langer R, Vacanti J, Cheney M. A tissue-engineered conduit for peripheral nerve repair. Arch Otolaryngol Head Neck Surg. 1998; 124:1081–1086. PMID: 9776185.


5. Evans GR, Brandt K, Katz S, Chauvin P, Otto L, Bogle M, et al. Bioactive poly(L-lactic acid) conduits seeded with Schwann cells for peripheral nerve regeneration. Biomaterials. 2002; 23:841–848. PMID: 11774850.


6. Takata T, Wang HL, Miyauchi M. Migration of osteoblastic cells on various guided bone regeneration membranes. Clin Oral Implants Res. 2001; 12:332–338. PMID: 11488862.


7. Fine EG, Decosterd I, Papaloizos M, Zurn AD, Aebischer P. GDNF and NGF released by synthetic guidance channels support sciatic nerve regeneration across a long gap. Eur J Neurosci. 2002; 15:589–601. PMID: 11886440.


8. Kotwal A, Schmidt CE. Electrical stimulation alters protein adsorption and nerve cell interactions with electrically conducting biomaterials. Biomaterials. 2001; 22:1055–1064. PMID: 11352099.


9. Shen ZL, Lassner F, Becker M, Walter GF, Bader A, Berger A. Viability of cultured nerve grafts: An assessment of proliferation of Schwann cells and fibroblasts. Microsurgery. 1999; 19:356–363. PMID: 10594909.


10. Jia Z, Shen D, Xu W. Synthesis and antibacterial activities of quaternary ammonium salt of chitosan. Carbohydr Res. 2001; 333:1–6. PMID: 11423105.


11. Bryan DJ, Holway AH, Wang KK, Silva AE, Trantolo DJ, Wise D, et al. Influence of glial growth factor and Schwann cells in a bioresorbable guidance channel on peripheral nerve regeneration. Tissue Eng. 2000; 6:129–138. PMID: 10941208.


12. Araki T, Nagarajan R, Milbrandt J. Identification of genes induced in peripheral nerve after injury. Expression profiling and novel gene discovery. J Biol Chem. 2001; 276:34131–34141. PMID: 11427537.
13. Copertino DW, Edelman GM, Jones FS. Multiple promoter elements differentially regulate the expression of the mouse tenascin gene. Proc Natl Acad Sci U S A. 1997; 94:1846–1851. PMID: 9050867.


14. Pellen-Mussi P, Fravalo P, Guigand M, Bonnaure-Mallet M. Evaluation of cellular proliferation on collagenous membranes. J Biomed Mater Res. 1997; 36:331–336. PMID: 9260104.


15. Chernousov MA, Carey DJ. Schwann cell extracellular matrix molecules and their receptors. Histol Histopathol. 2000; 15:593–601. PMID: 10809381.
16. Probstmeier R, Nellen J, Gloor S, Wernig A, Pesheva P. Tenascin-R is expressed by Schwann cells in the peripheral nervous system. J Neurosci Res. 2001; 64:70–78. PMID: 11276053.


17. Jakovcevski I, Miljkovic D, Schachner M, Andjus PR. Tenascins and inflammation in disorders of the nervous system. Amino Acids. 2013; 44:1115–1127. PMID: 23269478.


18. Ahmed Z, Brown RA. Adhesion, alignment, and migration of cultured Schwann cells on ultrathin fibronectin fibres. Cell Motil Cytoskeleton. 1999; 42:331–343. PMID: 10223638.


19. Chen YS, Hsieh CL, Tsai CC, Chen TH, Cheng WC, Hu CL, et al. Peripheral nerve regeneration using silicone rubber chambers filled with collagen, laminin and fibronectin. Biomaterials. 2000; 21:1541–1547. PMID: 10885726.


20. Varon S, Adler R. Nerve growth factors and control of nerve growth. Curr Top Dev Biol. 1980; 16:207–252. PMID: 6258863.
21. Simain-Sato F, Lahmouzi J, Kalykakis GK, Heinen E, Defresne MP, De Pauw MC, et al. Culture of gingival fibroblasts on bioabsorbable regenerative materials in vitro. J Periodontol. 1999; 70:1234–1239. PMID: 10534079.


22. Cheng L, Esch FS, Marchionni MA, Mudge AW. Control of Schwann cell survival and proliferation: autocrine factors and neuregulins. Mol Cell Neurosci. 1998; 12:141–156. PMID: 9790735.


23. DiStefano PS, Johnson EM Jr. Nerve growth factor receptors on cultured rat Schwann cells. J Neurosci. 1988; 8:231–241. PMID: 2828562.


Fig. 1
Morphological appearance of tissue-engineered nerve conduit. Grossly, it has hollow and round structure with 1-mm inner diameter and 0.2-mm thickness and prepared in 5-mm length (A). The SEM view shows the multi-porous outer surface (B, × 1000, left; × 3000, right).
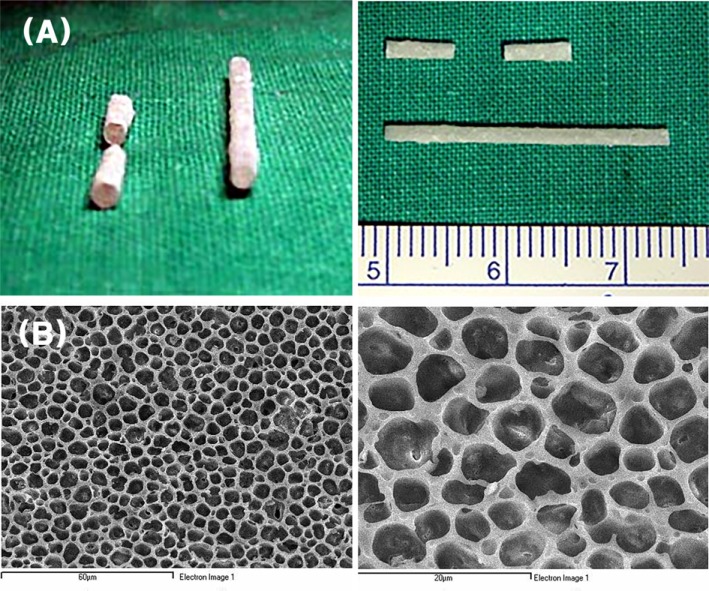
Fig. 2
Cellular attachment on tissue-engineered nerve conduit by MTT assay in control, Group I and Group II after 48 hr incubation (*P < 0.05, compared with control; SC = Schwann cell).
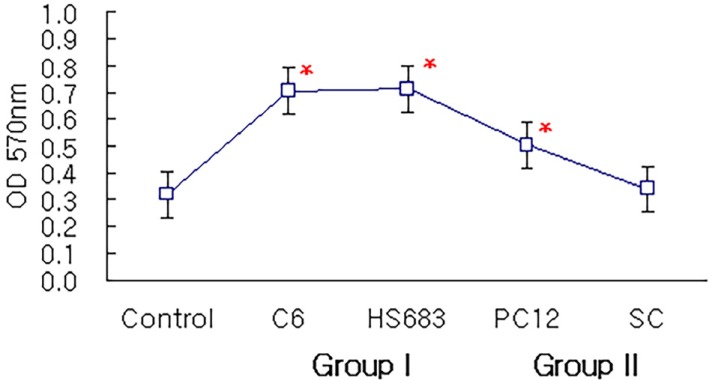
Fig. 3
Cellular attachment on tissue-engineered nerve conduit by MTT assay in control, Group II and Group III after 48 hr incubation. (*P < 0.05, compared with control, #P < 0.05, compared with Group II; SC = Schwann cell).
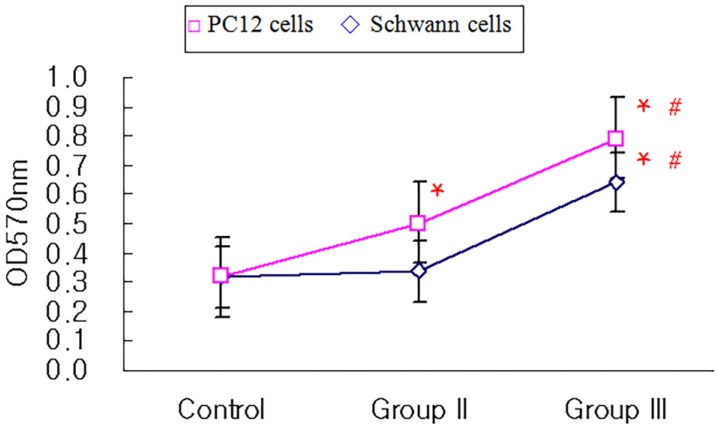
Fig. 4
SEM views of cell-nerve conduit complexes in Group I and Group II. C6 cells (A; ×1000), HS683 cells (B; ×1000) and Schwann cells (D; ×1000) show polygonal shape but PC12 cells (C; ×1000) show small and round shape.
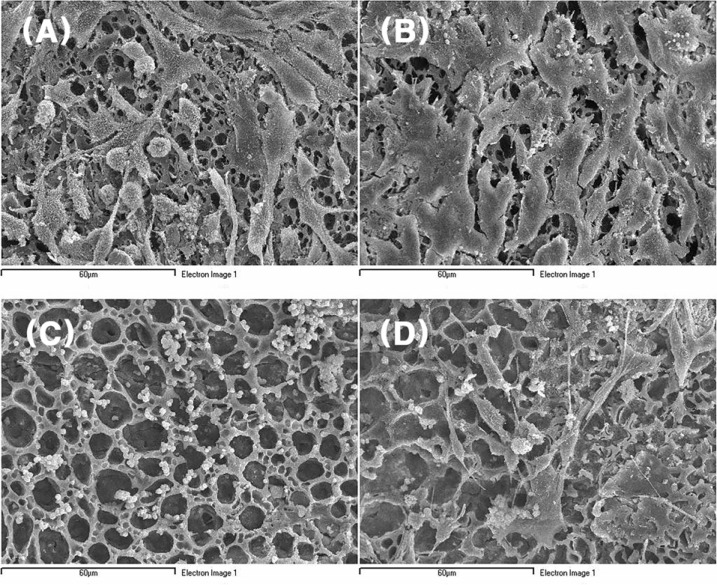
Fig. 5
SEM views of cell-nerve conduit complexes in Group III. The cells in Group III, PC 12 cells treated with NGF (A; ×1000) and Schwann cells with GDNF (B; ×1000), were morphologically changed from small and round shape to spindle shape with elongated cytoplasmic extension and reached confluency (C; ×1000) at the same 48 hr incubation.
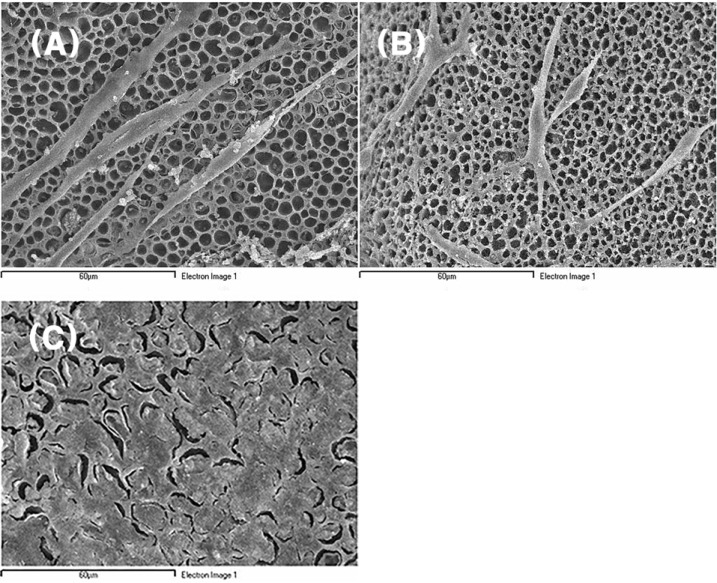
Fig. 6
Cellular attachment on tenascin-coated nerve conduit by MTT assay in C6 cells and Schwann cells after 48 hr incubation (TN = Tenascin, SC = Schwann cell).
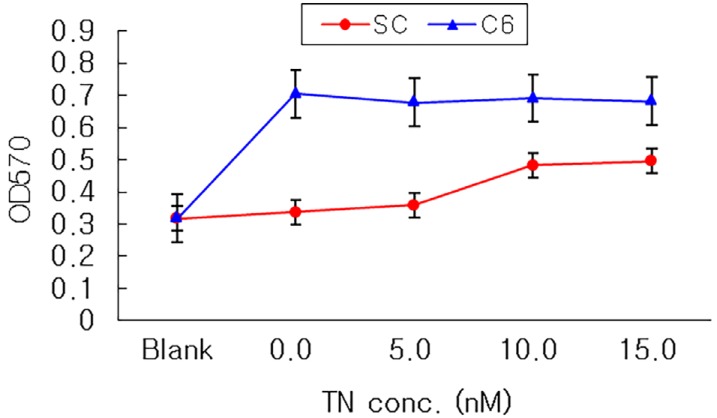
Fig. 7
Cellular attachment on fibronectin-coated nerve conduit by MTT assay in C6 cells and Schwann cells after 48 hr incubation (*P < 0.05, compared with none-coated nerve conduit; FN = Fibronectin, SC = Schwann cell).
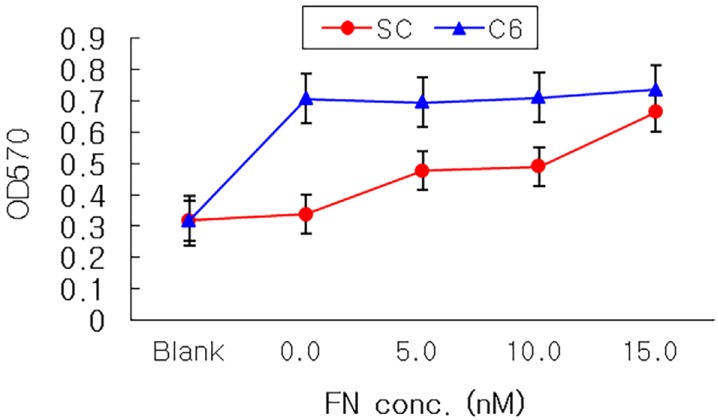
Table 1
The summary of the relationship between cell cycle and cellular attachment in Group I, Group II, and Group III.

Cell type | Cell cycle | Cell attachment | |
---|---|---|---|
Group I | C6 cells | +++ | +++ |
HS683 cells | +++ | +++ | |
Group II | PC12 cells | + | ++ |
Schwann cells | + | + | |
Group II | PC12 cells + NGF | ++ | +++ |
Schwann cells + GDNF | ++ | +++ |