Abstract
Background
Endotracheal intubation induces clinically adverse cardiovascular changes. Various pharmacological strategies for controlling these responses have been suggested with opioids being widely administered. In this study, the optimal effect-site concentration (Ce) of remifentanil for minimizing hemodynamic responses to fiberoptic nasotracheal intubation was evaluated.
Methods
Thirty patients, aged 18-63 years, scheduled for elective surgery were included. Anesthesia was induced with a propofol and remifentanil infusion via target-controlled infusion (TCI). Remifentanil infusion was initiated at 3.0 ng/mL, and the response of each patient determined the Ce of remifentanil for the next patient by the Dixon up-and-down method at an interval of 0.5 ng/mL. Rocuronium was administered after propofol and remifentanil reached their preset Ce; 90 seconds later fiberoptic nasotracheal intubation was initiated. Non-invasive blood pressure and heart rate (HR) were measured at pre-induction, the time Ce was reached, immediately before and after intubation, and at 1 and 3 minutes after intubation. The up-and-down criteria comprised a 20% change in mean blood pressure and HR between just prior to intubation and 1 minute after intubation.
Results
The median effective effect-site concentration (EC50) of remifentanil was 3.11 ± 0.38 ng/mL by the Dixon's up-and-down method. From the probit analysis, the EC50 of remifentanil was 3.43 ng/mL (95% confidence interval, 2.90-4.06 ng/mL). In PAVA, the EC50 and EC95 of remifentanil were 3.57 ng/mL (95% CI, 2.95-3.89) and 4.35 ng/mL (95% CI, 3.93-4.45). No remifentanil-related complications were observed.
Nasotracheal intubation is often necessary in patients undergoing oral and maxillofacial surgery [1]. The incidence of difficult airway intubation in these patients is 15.4%-16.9% due to temporomandibular joint diseases, facial abnormalities, and fractures [23]. Fiberoptic bronchoscope is considered the best choice for the management of difficult airways [4] and the most reliable tool for nasotracheal intubation [5].
Nasotracheal intubation, however, may cause hemodynamic changes. It may stimulate the sympathetic nerve system and cause a greater hypertensive response than orotracheal intubation [6]. The resultant increase in blood pressure and heart rate (HR) increases the risk of myocardial ischemia, left ventricular failure, arrhythmia, and cerebral hemorrhage [7]. The administration of local anesthetics, beta blockers, alpha-2 agonists, and opioids to prevent these problems during endotracheal intubation has been previously reported [89101112131415].
Remifentanil is one such opioid that may effectively ameliorate cardiovascular responses to short-term detrimental stimulations such as nasotracheal intubation [16]. It has a rapid onset and an ultrashort duration of action; it is more stable and has a shorter context-sensitive half-time than other opioids [17].
Previous studies have reported the use, but not the appropriate concentration of remifentanil for preventing cardiovascular changes secondary to nasotracheal intubation using a fiberscope. Therefore, the aim of this study was to evaluate the median effective effect-site concentration (EC50) of remifentanil for minimizing hemodynamic responses to fiberoptic nasotracheal intubation.
Consecutive patients scheduled for brief oral and maxillofacial surgery at Pusan National University Dental Hospital were eligible for enrollment in this study (Table 1). Written informed consent was obtained from all patients, and the study was approved by Institutional Review Board of Pusan National University Dental Hospital. Included patients were between the age of 18 and 63 years with an American Society of Anesthesiologists physical status classification of I or II. Patients with pre-existing cardiovascular, pulmonary, renal disease, or upper airway deformation, and those taking analgesic or antihypertensive medications were excluded. None of the patients were administered medication before the study. Each patient received an 18-gauge intravenous catheter inserted in the forearm, and the following standard monitoring was performed: electrocardiography, noninvasive blood pressure measurement, pulse oximetry (Solaris 8000M Patient Monitor, GE Medical Systems, Milwaukee, Wisconsin, USA), and bispectral index (BIS) monitoring (BIS-XP monitor, Aspect Medical Systems Inc., Natick, Massachusetts, USA). All patients were oxygenated for 5 minutes before induction of anesthesia.
Remifentanil was diluted with 50 mL normal saline. The resulting 20 µg/mL solution was introduced into a commercial target-controlled infusion (TCI) device (Orchestra Base Primea, Fresenius Vial, France). Also propofol was infused using same TCI device. A microvolume extension tube was connected to the intravenous catheter with a three-way stopcock. The pharmacokinetic models were Minto for remifentanil and Marsh and Schnider for propofol.
Oxygen saturation, systolic blood pressure, diastolic blood pressure, mean blood pressure (MBP), HR, BIS, and end-tidal CO2 were recorded before induction of anesthesia (baseline), at the time effect-site concentration (Ce) was reached, immediately before and after intubation, and at 1 and 3 minutes after intubation. Nasotracheal intubation was performed with a 6.0-mm Ring-Adair-Elwyn nasal tube (Portex®; Smiths Medical International, Hythe, UK) in women and with a 6.5-mm tube in men.
All patients received anesthesia with a combination of propofol and remifentanil, concomitantly with running fluid. The dose of propofol was an effect-site TCI of 4 µg/mL. Since the EC50 of remifentanil needed to prevent cardiovascular changes after nasotracheal intubation in the presence of propofol was unknown, the sample size was calculated using the up-and-down experimental design described by Dixon [1819].
After the Ce of propofol and remifentanil was reached, each patient received rocuronium 0.8 mg/kg intravenously, followed by nasotracheal intubation 90 seconds later. We initiated remifentanil infusions at 3 ng/mL; each patient's response determined the target Ce of remifentanil administered to the next patient. A response was considered successful if HR and MBP values recorded 1 minute after intubation did not exceed 20% of the values recorded immediately prior to intubation; if they did, a failed response was determined. If a response was successful, the target concentration of remifentanil was lowered by 0.5 ng/mL for the next patient; if a response failed, the target concentration of remifentanil was increased by 0.5 ng/mL [20]. For minimizine the bias, only one skilled anesthesiologist performed fiberoptic nasotracheal intubation.
An up-and-down method was used to calculate EC50. The transition between consecutive successful and failed responses comprised a crossover, with a midpoint concentration between the successful and failed response concentrations. The midpoint crossover concentrations were averaged to determine the EC50. A probit analysis and pooled-adjacent-violators algorithm (PAVA) were also used to calculate the EC50, the EC95 (the effect-site concentration of remifentanil necessary to prevent a response to a particular stimulus in 95% of patients), and the confidence interval (CI). Data were presented as mean ± standard deviations (SD). Statistical Package for Social Sciences version 13 (SPSS Inc., Chicago, Illinois, USA) was used for the statistical analyses; P-values < 0.05 were considered statistically significant.
Thirty patients were enrolled in the study initially. Three patients were excluded: one due to insufficient anesthesia, one due to a significant reduction in MBP, and one due to misuse of remifentanil concentration. Therefore, in total, 27 patients were included in the study (Table 1). In all patients, fiberoptic nasotracheal intubation was performed successfully, with no complications. Dose-response data obtained by Dixon's up-and-down method are shown in Fig. 1. The EC50 of remifentanil required to minimize cardiovascular changes secondary to fiberoptic nasotracheal intubation, calculated from seven crossover values, was 3.11 ± 0.38 ng/mL.
In the probit analysis, the EC50 and EC95 of remifentanil was 3.43 ng/mL (95% CI, 2.90-4.06 ng/mL) and 4.52 ng/mL (95% CI, 3.96-9.03 ng/mL), respectively (Fig. 2).
In PAVA, the EC50 and EC95 of remifentanil were 3.57 ng/mL (95% CI, 2.95-3.89) and 4.35 ng/mL (95% CI, 3.93-4.45).
Mean HR and MBP values decreased after infusion of propofol and remifentanil. These reductions were statistically significant, but were not clinically meaningful (Fig. 3).
Nasotracheal and endotracheal intubation activates the sympathoadrenal system, increasing catecholamine release and causing elevations in blood pressure, HR, and the incidence of arrhythmia [212223242526]. Severe sequelae such as myocardial ischemia may occur in patients with hypertension and ischemic heart disease risk factors [27]. Furthermore, a nasotracheal tube is passed through the nose and nasopharynx, stimulating areas unaffected by orotracheal intubation, resulting in a greater hypertensive response to intubation [2628]. Fiberoptic intubation can be performed either orally or nasally, and is less invasive than direct laryngoscopic intubation; however, this method also results in hemodynamic changes.
Several methods for reducing cardiovascular changes during endotracheal intubation have been studied. Stoelting [8] stated that laryngotracheal lidocaine administration immediately prior to tracheal intubation reduced changes in blood pressure and led to spontaneous recovery of MBP and HR. Siedlecki [9] reported that blockade of beta adrenergic receptors reduced the intensity of cardiovascular disturbances. Alpha-2 agonists such as clonidine and dexmedetomidine are used to reduce stimulation of the sympathetic nervous system and hemodynamic changes during anesthetic induction [1112131415]. Opioids have also been used to prevent these changes [10].
One such opioid is remifentanil; it effectively reduces hemodynamic changes during endotracheal intubation [16293031]. This short-acting opioid has a rapid onset and a blood-brain equilibrium half-time of 1 to 2 minutes [17]. It has a short context-sensitive half-time of 3 minutes, regardless of infusion time and does not accumulate, even in patients with hepatic or renal failure [1617]. For these reasons, remifentanil is an optimal choice during the substantially stimulating, but usually brief, airway manipulation in fiberoptic nasotracheal intubation.
No studies to date have reported the EC50 of remifentanil for the reduction of cardiovascular changes during fiberoptic nasotracheal intubation. Our results indicate that continuous administration of remifentanil stabilizes hemodynamic responses. Our calculations show that the remifentanil EC50 required to minimize cardiovascular changes after nasotracheal intubation with a fiberscope under general anesthesia, using a TCI of propofol, is 3.11 ± 0.38 ng/mL.
In this study, we administered remifentanil after initiating an infusion of propofol at 4 µg/mL. Many studies have reported the concentration of propofol required to produce appropriate unconsciousness during intravenous anesthesia; the propofol Ce required for loss of consciousness in 90% of patients is 4 µg/mL [3233]. Vuyk et al. [33] reported the EC50 and EC95 of propofol (to induce unconsciousness in 50% and 95% of patients, respectively) as 3.4 µg/mL and 4.34 µg/mL, respectively. Another study reported that the Ce of propofol required to maintain the BIS at 41.1 ± 2.5 was 3.5 µg/mL [34]. Based on the results of these studies, we determined that the Ce of propofol required to produce unconsciousness was 4 µg/mL. The depth of anesthesia induced by propofol can affect cardiovascular changes during nasotracheal intubation. Inadequate anesthesia during nasotracheal intubation may result in substantial changes in HR and blood pressure that make it difficult to determine the effect of remifentanil. To exclude the influence of inadequate anesthesia, we administered a propofol Ce of 4 µg/mL to reach a target BIS range of 40 to 60 before nasotracheal intubation.
A limitation of this study was the wide range of CIs for the EC50 and EC95 of remifentanil. The statistical precision of a study may be improved by increasing the sample size. Using the Dixon up-and-down method, however, a sample size of 20 to 40 patients provides reliable results for most realistic simulations [35]. A survey of the current utilization of the up-and-down method in anesthesia studies found that most include 20 or more patients in sequential trials. Therefore, while the sample size in this study was sufficient for the up-anddown method, the CIs for the EC50 and EC95 were unrealistically wide. We calculated the EC50 and EC95 using two statistical methods: a nonparametric turning point estimator and a parametric probit estimator. In the turning point estimator, the midpoint concentrations of crossovers were averaged. This method is commonly used in anesthesia up-and-down method studies [36]. The probit estimator method has been censured for some biases in its accuracy. This method has been adapted to the non-independence of up-and-down data; however, tolerance distribution symmetry is necessary [37]. For this reason, researchers should consider the use of an isotonic regression estimator with CI derived from boot strapping. This is a well-described variant of restricted least squares regression that constrains the point estimates as either monotonic increasing or monotonic decreasing [38]. This statistical method may be constant over a range of doses. Therefore, we attempted to estimate the EC50 and EC95 by an isotonic regression estimator.
The EC50 and EC95 derived from the isotonic regression estimator pooled-adjacent-violators algorithm (PAVA) was 3.57 ng/mL (95% CI, 2.95-3.89) and 4.35 ng/mL (95% CI, 3.93-4.45), respectively. The probit analysis and PAVA adjusted probability graphs overlapped because there were no probability violations against the dose elevations. Although the probit analysis showed a well-defined graph in this study, the CIs of the EC50 and EC95 were impractically wide because the parameter estimate of the regression slope was biased. The isometric regression estimator was superior to the probit analysis in terms of CI because it was calculated from the results of a boot strapping method and resulted in more acceptable CI ranges.
Another consideration in this study is the degree of cardiovascular changes in patients after infusion of propofol and remifentanil. Substantial changes in cardiovascular status may render it clinically difficult to administer propofol and remifentanil in combination, and may require the use of inotropic agents such as ephedrine. In our study, reductions in HR and MBP after administration of propofol and remifentanil were not clinically meaningful and did not require the administration of inotropic agents.
In conclusion, we found that the EC50 of remifentanil needed to minimize hemodynamic responses to fiberoptic nasotracheal intubation, calculated by the Dixon up-anddown method, is 3.11 ± 0.38 ng/mL, with a propofol Ce of 4 µg/mL. In a probit analysis, the EC50 was 3.43 ng/mL (95% CI, 2.90-4.06 ng/mL) and the EC95 was 4.52 ng/mL (95% CI, 3.96-9.03 ng/mL). The patients in this study maintained cardiovascular stability during fiberoptic nasotracheal intubation by remifentanil infusion.
Figures and Tables
Fig. 1
Up-and-down sequence. A successful response was defined as the increase of heart rate (HR) and mean blood pressure (MBP) 1 minute after nasotracheal intubation with a fiberscope that did not exceed 20% of the value just before intubation. If HR or MBP exceeded 20% of the pre-intubation value, it was defined as a failed response. A change between consecutive successful and failed responses is a "crossover" with a midpoint concentration between the successful and failed concentrations (arrows). Seven crossovers were averaged to determine the median effective effect-site concentration of remifentanil.
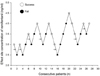
Fig. 2
The effect-site concentration and response curves of remifentanil from the probit analysis. EC50 is 3.43 ng/mL (95% confidence interval [CI], 2.90-4.06 ng/mL) and EC95 is 4.52 ng/mL (95% CI, 3.96-9.03 ng/mL).
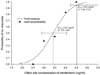
Fig. 3
Changes in heart rate (HR) and mean blood pressure (MBP). Data presented as mean ± SD. Baseline (B): before administration of drug, After infusion (tCe): after effect-site target concentration (Ce) of both propofol and remifentanil reached target concentration, Immediately after intubation (I0): right after nasotracheal intubation with fiberscope. * P < 0.05 as compared with tCe.
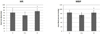
References
1. Hall CE, Shutt LE. Nasotracheal intubation for head and neck surgery. Anaesthesia. 2003; 58:249–256.


2. Matsuura H, Hirose I, Joh S, Sugiyama K, Niwa H, Shibutani T. A report of 14,195 applications of anesthetics to oral and maxillofacial surgery at one teaching dental hospital (1971–2000) centering around airway problems. J Clin Anesth. 2000; 12:460–467.


3. Tuzuner-Oncul AM, Kucukyavuz Z. Prevalence and prediction of difficult intubation in maxillofacial surgery patients. J Oral Maxillofac Surg. 2008; 66:1652–1658.


4. Dong Y, Li G, Wu W, Su R, Shao Y. Lightwand-guided nasotracheal intubation in oromaxillofacial surgery patients with anticipated difficult airways: A comparison with blind nasal intubation. Int J Oral Maxillofac Surg. 2013; 42:1049–1053.


5. Umesh G, Manjunath P, Kini G, Jasvinder K. A technique to overcome inability to advance a tracheal tube over a fiberscope during nasotracheal intubation. J Anesth. 2010; 24:819–820.


6. Shibata Y, Okamoto K, Matsumoto M, Suzuki K, Sadanaga M, Morioka T. Cardiovascular responses to fiberoptic intubation: A comparison of orotracheal and nasotracheal intubation. J Anesth. 1992; 6:262–268.


7. Roy WL, Edelist G, Gilbert B. Myocardial ischemia during non-cardiac surgical procedures in patients with coronaryartery disease. Anesthesiology. 1979; 51:393–397.


8. Stoelting RK. Blood pressure and heart rate changes during short-duration laryngoscopy for tracheal intubation: Influence of viscous or intravenous lidocaine. Anesth Analg. 1978; 57:197–199.
9. Siedlecki J. Disturbances in the function of cardiovascular system in patients following endotracheal intubation and attempts of their prevention by pharmacological bloackade of sympathetic system. Anaesth Resusc Intensive Ther. 1975; 3:107–123.
10. Stanley TH, Berman L, Green O, Robertson D. Plasma catecholamine and cortisol responses to fentanyl--oxygen anesthesia for coronary-artery operations. Anesthesiology. 1980; 53:250–253.


11. Hayashi Y, Maze M. Alpha 2 adrenoceptor agonists and anaesthesia. Br J Anaesth. 1993; 71:108–118.


12. Ghignone M, Quintin L, Duke PC, Kehler CH, Calvillo O. Effects of clonidine on narcotic requirements and hemodynamic response during induction of fentanyl anesthesia and endotracheal intubation. Anesthesiology. 1986; 64:36–42.


13. Virtanen R, Savola JM, Saano V, Nyman L. Characterization of the selectivity, specificity and potency of medetomidine as an alpha 2-adrenoceptor agonist. Eur J Pharmacol. 1988; 150:9–14.


14. Jaakola ML, Ali-Melkkila T, Kanto J, Kallio A, Scheinin H, Scheinin M. Dexmedetomidine reduces intraocular pressure, intubation responses and anaesthetic requirements in patients undergoing ophthalmic surgery. Br J Anaesth. 1992; 68:570–575.


15. Lawrence CJ, De Lange S. Effects of a single pre-operative dexmedetomidine dose on isoflurane requirements and peri-operative haemodynamic stability. Anaesthesia. 1997; 52:736–744.


16. Beers R, Camporesi E. Remifentanil update: Clinical science and utility. CNS Drugs. 2004; 18:1085–1104.
17. Glass PS, Hardman D, Kamiyama Y, Quill TJ, Marton G, Donn KH, et al. Preliminary pharmacokinetics and pharmacodynamics of an ultra-short-acting opioid: Remifentanil (GI87084B). Anesth Analg. 1993; 77:1031–1040.
18. Dixon WJ. Efficient analysis of experimental observations. Annu Rev Pharmacol Toxicol. 1980; 20:441–462.


20. Lee J, Jung C. The target concentration of remifentanil to suppress the hemodynamic response to endotracheal intubation during inhalational induction with desflurane. Korean J Anesthesiol. 2011; 60:12–18.


21. Shribman AJ, Smith G, Achola KJ. Cardiovascular and catecholamine responses to laryngoscopy with and without tracheal intubation. Br J Anaesth. 1987; 59:295–299.


22. Edwards ND, Alford AM, Dobson PM, Peacock JE, Reilly CS. Myocardial ischaemia during tracheal intubation and extubation. Br J Anaesth. 1994; 73:537–539.


23. Mikawa K, Nishina K, Maekawa N, Obara H. Comparison of nicardipine, diltiazem and verapamil for controlling the cardiovascular responses to tracheal intubation. Br J Anaesth. 1996; 76:221–226.


24. Yoo KY, Lee JU, Kim HS, Im WM. Hemodynamic and catecholamine responses to laryngoscopy and tracheal intubation in patients with complete spinal cord injuries. Anesthesiology. 2001; 95:647–651.


25. Burney RG, Winn R. Increased cerbrospinal fluid pressure during laryngoscopy and intubation for induction of anesthesia. Anesth Analg. 1975; 54:687–690.
26. Singh S, Smith JE. Cardiovascular changes after the three stages of nasotracheal intubation. Br J Anaesth. 2003; 91:667–671.


27. Roy WL, Edelist G, Gilbert B. Myocardial ischemia during non-cardiac surgical procedures in patients with coronary-artery disease. Anesthesiology. 1979; 51:393–397.


28. Fassoulaki A, Andreopoulou K, Saleh M, Kitharitzi D. Metabolic and cardiovascular responses following oral and nasal intubation of the trachea. Acta Anaesthesiol Belg. 1990; 41:281–286.
29. Burkle H, Dunbar S, Van Aken H. Remifentanil: A novel, short-acting, mu-opioid. Anesth Analg. 1996; 83:646–651.
30. Lallo A, Billard V, Bourgain JL. A comparison of propofol and remifentanil target-controlled infusions to facilitate fiberoptic nasotracheal intubation. Anesth Analg. 2009; 108:852–857.


31. Kim KO, Chung S, Chang EJ, Lee Y. Optimal effect-site concentration of remifentanil for preventing development of hypertension during tracheal intubation with inhaled desflurane induction. Korean J Anesthesiol. 2011; 60:8–11.


32. Smith C, McEwan AI, Jhaveri R, Wilkinson M, Goodman D, Smith LR, et al. The interaction of fentanyl on the Cp50 of propofol for loss of consciousness and skin incision. Anesthesiology. 1994; 81:820–828.


33. Vuyk J, Engbers FH, Burm AG, Vletter AA, Griever GE, Olofsen E, et al. Pharmacodynamic interaction between propofol and alfentanil when given for induction of anesthesia. Anesthesiology. 1996; 84:288–299.


34. Kil HY, Lee SI, Lee SJ, Lee SW, Lee DH. The bispectral index and modified observer's assessment of alertness/sedation scale comparable to effect site concentration of propofol in koreans. Korean J Anesthesiol. 2000; 38:251–257.


35. Stylianou M. Sequential analysis of durham and Flournoy's biased coin design for phase I clinical trials. American University;2000. p. 356.
37. Stylianou M, Flournoy N. Dose finding using the biased coin Up–and–Down design and isotonic regression. Biometrics. 2002; 58:171–177.


38. Robertson T, Wright F, Dykstra RL. Order restricted statistical inference. Wiley New York. 1988; 30:316.